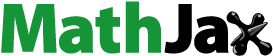
Abstract
Atmospheric polycyclic aromatic hydrocarbons (PAHs) are associated with adverse health effects in exposed populations. Understanding PAH pollution in ambient air and its adverse impacts is essential for developing effective actions for combating air pollution. This review paper summarised and discussed the findings of research work that determined the concentrations, distribution sequence, and health risk exposure levels of atmospheric PAHs in cities worldwide. It also gave an overview of the sources and the methodology for appraising the health risk of exposure to atmospheric PAHs. Eighty-five articles published in peer-reviewed journals from 2010 to 2023 were identified in academic research databases, with 55 reporting on sources and distribution of atmospheric PAHs, while 30 evaluated the health risk of exposure to PAHs in ambient air. The narrative review approach was employed to critically analyse and produce descriptive summaries of distribution patterns and health risk exposure levels of atmospheric PAHs in a tabular form. The review showed that the total PAH concentration in urban areas was about eight times greater than in rural environments and emanated from diverse sources. However, vehicle exhaust and industrial emissions were the primary sources of atmospheric PAHs. The distribution sequence of PAHs in particulate and gas phases was HMW>MMW>LMW PAHs and LMW>MMW>HMW PAHs, respectively. Chronic exposure to PAHs in the environment increased the risk of asthma, cardiovascular disease, and lung cancer. Adoption of stricter emission standards, cleaner energy sources, and more efficient industrial technology will aid in the reduction of particulate PAH pollution and its negative health consequences in urban areas.
PUBLIC INTEREST STATEMENT
PAH pollution in ambient air remains an issue worldwide. PAHs are classified as priority pollutants due to their mutagenic and carcinogenic attributes. In the atmosphere, PAHs exist in the gas and as a sorbent to aerosols. Particulate PAHs can move into the lungs and deploy their toxic impacts over longer periods than gaseous PAHs. In contrast, PAHs present in the gas phase can reach the alveolar epithelium through inhalation and rapidly transfix into the circulatory system. A better insight into PAH pollution in the air and its adverse health implications is vital for designing effective sustainable actions for abating air pollution. In this study, existing knowledge about the sources, distribution, and health risk exposure levels of atmospheric PAHs was summarised and discussed. The summarised data will help inform the rationale for specific intervention studies and the design of targeted public health actions to deal with PAH pollution in atmospheric air.
1. Introduction
Air pollution has been a major challenge worldwide and the biggest environmental risk to health (Asril et al., Citation2023; Gibergans-Baguena et al., Citation2020). Atmospheric aerosol is one of the air pollutants that has been identified and regulated by the World Health Organization (Citation2014) as a measure for protecting public health. Atmospheric particulates contain several harmful organic compounds including polycyclic aromatic compounds (Kalisa et al., Citation2018; Nowakowski et al., Citation2022), which are of concern because of their immense adverse impacts on human health (S. N. Kumar et al., Citation2014). Due to the widespread sources and persistent characteristics, polycyclic aromatic hydrocarbons (PAHs) spread through atmospheric circulation and exist almost everywhere (Hussain & Medhi, Citation2018). The persistent nature of PAHs in the atmospheric environment is primarily due to their low water solubility and high thermal stability (Kim et al., Citation2013).
PAHs are released into the atmosphere through natural processes such as bushfires and volcanic eruptions. However, high temperature-induced processes such as incomplete burning of wood biomass, municipal waste, and fossil fuel (oil, coal, and natural gas) are the main sources of atmospheric PAHs (Lammel, Citation2015). The origin of PAHs in ambient particulates in southern China was investigated (P. Li et al., Citation2015). The study revealed that vehicle emissions, coal burning, and unburnt fossil fuels were the sources of PAHs in the study area. The results of an investigation that ascertained sources of particulate-bound PAHs in an industrial location in India showed that emissions from coal, gasoline, diesel, and natural gas combustion were the major sources of atmospheric PAHs (Lakhani, Citation2012).
The findings of a study that measured the levels of PAH composition in aerosol particulates from residential cookstoves indicated that wood burning in the natural-draft stove recorded the highest PAH emissions followed by wood combustion in the forced-draft stove and kerosene burning (Shen et al., Citation2012). In a similar study that diagnosed the sources of atmospheric PAHs in an urban setting in Central Poland, it was found that coal combustion for domestic heating and industrial use was the most noticeable PAH source (Siudek & Frankowski, Citation2018). Analysis of the findings shown earlier indicates that atmospheric PAHs are emitted from mixed sources. Awareness of the contribution levels of emission sources is needed to adequately manage PAH pollution in the atmosphere (Yang et al., Citation2021).
The relative distribution of individual PAH congeners is critical for assessing health risks and allocating pollution sources (Gupta et al., Citation2011). Moradi et al. (Citation2022) reported that two- and three-ringed PAHs contributed up to 39% and 76%, respectively, to the total PAHs in urban and semi-urban areas in Toronto, Canada. Also, the distribution sequence of atmospheric PAHs and source detection using biomarkers from five Asian megacities were established (Saha et al., Citation2017). The study revealed that the atmospheric PAH concentrations and distribution patterns in the five cities differed with average concentrations of 2.99, 3.95, 7.99, 63.5, and 142.8 ng/m3. Furthermore, the most abundant atmospheric PAHs were chrysene, benz[a]anthracene, benzo[b]fluoranthene, benzo[a]pyrene, and benzo[e]pyrene. The variation in atmospheric PAH levels in the cities was attributed to differences in emission sources, pollution levels, and atmospheric conditions (Wu et al., Citation2019). Similar findings have been reported by other studies carried out in cities worldwide (Abdallah & Atia, Citation2014; Arinaitwe et al., Citation2012; Lai et al., Citation2017; Lhotka et al., Citation2019). The heterogeneity in research findings about atmospheric PAH distribution makes regional and global planning and the design of sustainable strategies for abating PAH pollution in the ambient air, hence the need to summarise and discuss the concentrations and distribution patterns of atmospheric PAHs in cities worldwide.
Exposure to atmospheric PAHs causes asthma, pulmonary infections, skin diseases, and allergies (Oliveira et al., Citation2019). The health effects of individual PAHs differ from one another (Abdel-Shafy & Mansour, Citation2015). The intensity of health effects of PAH exposure is hinged on the dose, the duration of exposure, and individual characteristics such as age, sex, nutritional status, family traits, lifestyle, and state of health (International Agency for Research on Cancer, Citation2010). Research findings of most studies have shown that the cancer risks due to atmospheric PAH exposure through inhalation were high (Aslam et al., Citation2022; Kongpran et al., Citation2021; Safo-Adu et al., Citation2014; Y. Wang et al., Citation2022; Y. Zhang et al., Citation2019). However, only a few findings have indicated otherwise (Froehner et al., Citation2011; Jamhari et al., Citation2014). This is worrying and needs urgent attention. Better insight into the health impacts associated with airborne PAH exposure is mandatory for developing effective strategies for combating PAH pollution in atmospheric air and protecting public health.
Globally, air quality deterioration due to gas–particle PAH pollution remains an issue (Guerreiro et al., Citation2014). The health risk of exposure to atmospheric PAHs is on the rise, despite the numerous guideline values established to control PAHs emissions and ambient air pollution (Garrido et al., Citation2014; Lhotka et al., Citation2019). Most papers that reviewed existing knowledge about atmospheric PAHs focused on occurrence, transport, fate, and toxicity (Abdel-Shafy & Mansour, Citation2015; Bessagnet & Allemand, Citation2020; Lawal & Fantke, Citation2017; Nowakowski et al., Citation2022). Reports that discussed the current research findings on distribution and health risk exposure levels of atmospheric PAHs were few (Hussain & Medhi, Citation2018; Kim et al., Citation2013). This has served as an obstacle to understanding PAH pollution in ambient air and its associated health implications, which are vital for designing effective strategies for combating PAH pollution in the atmospheric environment. Limited databases with extensive geographical coverage and long temporal periodicity hamper most of the efforts to efficiently assess issues concerning atmospheric PAH pollution (Garrido et al., Citation2014). This paper seeks to fill this literature gap. This review paper summarised and discussed the findings of research work that determined the distribution patterns and health risk exposure levels of atmospheric PAHs. It also gives an overview of the sources and methodologies for appraising the health risk of exposure to atmospheric PAHs. This review paper provides policymakers with comprehensive information to formulate policies and strategies to curb the global health impact of exposure to atmospheric PAHs. The summarised data will help inform the rationale for specific intervention studies and the design of targeted public health actions to deal with PAH pollution in ambient air.
2. Method
A search of the Scopus, PubMed, and Google Scholar databases for papers published in peer-reviewed journals from 2010 to 2023 was conducted. Studies published before 2010 were excluded because they had already been covered by other review papers. This review included only studies that focused on the sources, concentration levels, distribution patterns, and health risks of exposure to atmospheric PAHs. Databases were comprehensively searched laterally using broad search terms or keywords combined using Boolean operators, such as the “AND/OR” command to ensure a broad scope of papers. “Levels of atmospheric PAHs” OR “PAHs concentration in ambient air”, “PAHs distribution pattern in ambient air” OR “distribution pattern of atmospheric PAHs”, “sources of atmospheric PAHs” OR “sources of ambient air PAHs”, “health impacts of atmospheric PAH” OR “health risks of exposure to PAH in ambient air”, health risk assessment of airborne PAHs” were among the keywords used during the search. The search was restricted to English-language papers. Two independent reviewers assessed the paper’s eligibility and quality, and any disagreements were resolved through consensus. This review included 85 articles, with 29 and 26 articles reporting on the sources and distribution of atmospheric PAHs, respectively, and 30 assessing the health risk of exposure to PAHs in ambient air. The narrative review method was used to critically analyse and produce descriptive summaries of atmospheric PAH concentrations, distribution patterns, and health risk exposure levels in tabular form.
3. Results and discussion
3.1. Characteristics of PAHs
PAHs are organic compounds with two or more fused benzene rings (Nowakowski et al., Citation2022). They are lipophilic and have very characteristic UV absorbance spectra. PAHs have high thermal stability and are hardly affected by photochemical and biological oxidation (Gao et al., Citation2017). As a result, most PAHs persist and bioaccumulate in the environment.
Several PAHs have been detected globally. However, 16 of the PAHs have been identified by the United States Environmental Protection Agency (US EPA) as priority pollutants that are recommended for environmental monitoring (Siudek & Frankowski, Citation2018). PAHs are classified based on their physical properties such as boiling points, melting points, molecular weight, and vapour pressure. Tables present the physical properties of US EPA priority and non-priority PAHs, respectively.
Table 1. Properties of US EPA priority PAHs (B. K. Lee & Vu, Citation2010; Ghosal et al., Citation2016; N. Zhang et al., Citation2022; Patel et al., Citation2020; Yang et al., Citation2021)
Table 2. Properties of some non-priority PAHs (B. K. Lee & Vu, Citation2010; Ghosal et al., Citation2016; N. Zhang et al., Citation2022; Patel et al., Citation2020; Yang et al., Citation2021)
PAHs can be categorised into three families based on their molecular weight. They include low-molecular-weight (LMW) PAHs, medium-molecular-weight (MMW) PAHs, and high-molecular-weight PAHs (Hussain & Medhi, Citation2018). LMW PAHs have two to three aromatic ring groups (Figure ) and have a molecular weight ranging from 152 to 178 g/mol. In addition, LMW PAHs have high vapour pressure and subsist in the gas phase (Yang et al., Citation2019). MMW PAHs have four aromatic rings (Figure ) with a molecular weight of 202 g/mol. HMW PAHs contain five to seven aromatic rings (Figure ), and they have molecular weights greater than or equal to 250 g/mol (L. Singh & Agarwal, Citation2020; L. Zhang et al., Citation2020). Furthermore, HMW PAHs have lower vapour pressure as a result, they occur in the particulate phase; while the vapour pressure of MMW PAHs lies between that of LMW and HMW PAHs, indicating that they exist in both gas and particulate phases (Hu et al., Citation2013). The half-lives of atmospheric PAHs span from a few hours to days. However, the half-lives of MMW and HMW PAHs are longer than that of LMW PAHs (Yang et al., Citation2019).
3.2. Sources of atmospheric PAHs
PAHs are emitted into the atmosphere primarily from partial combustion and high-temperature pyrolysis of carbon-containing substances (Abdel-Shafy & Mansour, Citation2015). The combustion sources can be either natural or human-induced (Cui et al., Citation2020).
3.2.1. Natural sources of atmospheric PAHs
Natural emission sources contributing to the concentration of atmospheric PAHs include volcanic eruptions, forests, and bushfires due to lightning strikes, erosion of sedimentary rocks containing petroleum hydrocarbons, and degradation of vegetation litter fall (Abdel-Shafy & Mansour, Citation2015).
3.2.2. Human-induced sources of atmospheric PAHs
Human-induced emission sources are the main determinants of PAH pollution in cities (Mojiri et al., Citation2019). The anthropogenic sources of atmospheric PAHs are categorised mainly into three, namely, mobile sources, stationary sources, and agricultural activities (Patel et al., Citation2020).
3.2.2.1. Mobile sources
Exhaust fumes from motor vehicles, railways, ships, and aircraft are the major mobile sources contributing to PAHs in the atmosphere. Emission of PAHs from mobile sources is linked to the utilisation of fuels such as coal, gasoline, diesel, oil, and lubricant oils. The most predominant source of PAHs in atmospheric aerosols is vehicular emissions (Gupta et al., Citation2011). An investigation that characterised the concentrations of atmospheric PAHs in the city of Shanghai, China, revealed that mobile emissions, particularly gasoline-powered vehicles, played a more important role in traffic-related PAH contamination (X. Wang et al., Citation2010). The findings of a study conducted on a major highway in Accra, Ghana, revealed that there was a strong positive correlation between total PAHs and daily vehicle traffic (R2 = 0.72), suggesting that vehicle traffic contributed to the high levels of atmospheric PAHs (Safo-Adu et al., Citation2014).
PAH emissions from vehicle exhaust are at ground level, widely dispersed, and concentrated in urban areas (B. K. Lee & Vu, Citation2010). Vehicle exhaust emissions, according to Shimada et al. (Citation2022), are the primary source of atmospheric PAHs in an urban environment. Based on the findings of a study that determined the sources of atmospheric PAHs at urban sites in the United Kingdom (Jang et al., Citation2013), vehicle exhaust contributed the most particulate PAHs in the study area. Similar findings were observed in other studies that diagnosed the sources of particulate PAHs in urban areas (Byambaa et al., Citation2019; Kalisa et al., Citation2018; Mishra et al., Citation2016; Qu et al., Citation2022). On the contrary, Nadali et al. (Citation2021) quantified the levels of PAHs in ambient air in Hamadan, Iran, and identified the major sources of PAH emissions to be light-duty vehicles, petrol combustion, and pyrogenic sources. It is suggested that atmospheric PAHs should be monitored periodically, and ecologically friendly fuels should be utilised to prevent PAHs pollution and health concerns in urban cities (Angelevska et al., Citation2021; Aslam et al., Citation2022).
3.2.2.2. Stationary sources
Stationary sources of atmospheric PAHs include emissions from industrial and domestic activities comprising combustion and pyrolysis of gas, garbage, oil, coal, wood, and other organic materials (World Health Organisation, Citation2021). Industrial activities such as heat and power generation, aluminium and coke production, cement production, crude oil processing, and waste incineration emit PAHs into the atmosphere (Y. Lee et al., Citation2010). Integrated iron and steel plants release a huge quantity of PAHs through stacks, fugitive emissions, and accidental spillages during the operations of various processing units (Gilio et al., Citation2020).
Industrial emissions contribute 65% to 80% to the level of atmospheric PAHs (Cui et al., Citation2020; Marinaite et al., Citation2022). W. Wang et al. (Citation2021) diagnosed the sources of atmospheric PAHs in Baoshan city in Shanghai, China, and discovered that immobile sources such as the steel industry made an immense contribution to the concentration of PAHs in the study area. Notwithstanding, the results of a study that determined the origin of PAHs in ambient air at urban, industrial, and semi-urban sites in Malaysia showed that the concentrations of PAHs measured at the industrial site were high (2.24 to 4.84 ng/m3) followed by semi-urban (1.64 to 3.45 ng/m3) and urban (1.33–2.97 ng/m3) areas (Jamhari et al., Citation2014). Besides, more than 80% of the total PAHs consisted of 5-ring and 6-ring PAHs, such as BaP, IcdP, BbF, BkF, and BghiP. Furthermore, Han et al. (Citation2011) ascertained the sources of particulate-bound PAHs in Anshan, an iron and steel production city, and in Qianshan, a non-industrial city in North-Eastern China, and found that a high concentration of PAHs occurred in the industrial city with high fractions of HMW PAHs than in the non-industrial city. An evaluation of the findings outlined above points to the fact that the contribution of industrial activities to PAHs in the atmosphere is immense and needs to be given serious attention.
Domestic activities comprising household burning of garbage, coal coking, wood burning for fish smoking and cooking, cooking on gas burners, and other residential activities contribute to the total emissions of PAHs to the atmosphere (Eriksson et al., Citation2014; Patel et al., Citation2020; Shen et al., Citation2018; Siudek & Frankowski, Citation2018). Siudek and Frankowski (Citation2018) identified the origin of atmospheric PAHs in a city in Central Poland and indicated that 5-ring PAH compounds such as BaP, DahA, BkF, and BbF emanated from mixed sources including combustion, wood burning, and industrial emission. On the contrary, research that evaluated the long-term trends in PAHs and their sources at a rural background site in Central Europe linked the sources of atmospheric PAHs to local heating (Lhotka et al., Citation2019). It was suggested that the planned substitution of old-fashioned combustion sources in households could enhance the air quality situation in the study area.
The speciation composition of PAHs in PM2.5 from open burning of municipal solid waste in China revealed that HMW PAH was dominant as compared to LMW PAHs (Cheng et al., Citation2022), while a study that investigated the diurnal variation of residential wood burning emissions and their contribution to the level of atmospheric PAH ascertained that residential wood combustion emissions contributed 62% to the total PAHs measured (Poulain et al., Citation2011). Areas with intense use of wood stoves and fireplaces had elevated levels of PAHs in ambient air. A similar finding was observed in a study that investigated the emission factors of PAHs from wood combustion for home cooking and heating in south-central Chile where residential wood biomass burning was a significant source of particulate PAH pollution in populated areas (Jimenez et al., Citation2017). The extent of emissions depended on the type of wood, the technology, and the operational conditions of the wood stove. It was recommended that apprehension of emissions from wood species and wood stove performance would help to identify better biomass fuels and technologies to abate air pollution from domestic wood burning in urban cities (Jimenez et al., Citation2017).
3.2.2.3. Agricultural sources
The emission of particulate PAHs from agricultural activities including agricultural residue combustion in farmlands is a major global concern as it harms human health and climate (Gurjar et al., Citation2016). High PAH pollution in rural areas is mainly due to agricultural sources (Patel et al., Citation2020). In developing countries, most farmers dispose of heaps of agricultural waste through combustion (Cheewaphongphan et al., Citation2011). This operation releases greenhouse gases and aerosol smoke containing PAHs into the environment, causing air pollution.
The outcome of research conducted in Vietnam showed that the total concentration of PAHs in fine particulates after open combustion of the rice straw was significantly higher than the level observed before open burning (Pham et al., Citation2019). Other studies reported similar findings (Fakinle et al., Citation2022; Pongpiachan et al., Citation2017; Shen et al., Citation2012; Z. Li et al., Citation2020). The PAH emissions from the burning of rice straws in agricultural farmlands are high. Immense efforts are required to curb airborne PAHs from the combustion of agricultural residues; otherwise, human health could be under threat, especially upon long-term exposure (Fakinle et al., Citation2022).
3.3. Source apportionment of atmospheric PAHs
The identification of primary sources of atmospheric PAHs serves as a tool for the adoption of sustainable abatement strategies for air pollution and is an essential step toward adequate protection of public health from exposure to PAHs (Afshar-Mohajer et al., Citation2016). Again, insight into the contributions of the various emission sources is needed to adequately manage PAH levels in the atmosphere. PAH molecular diagnostic ratios and receptor models have been used to identify the sources of atmospheric PAHs in many studies (Gupta et al., Citation2011; Qu et al., Citation2022; Y. Liu et al., Citation2015).
3.3.1. PAHs diagnostic ratios
The PAHs diagnostic ratio model is easy to use for source apportionment and does not rely on emission inventories as compared to supplicated atmospheric models (Dvorská et al., Citation2011; Gupta et al., Citation2011). The use of the molecular diagnostic ratio model for source apportionment of PAHs is hinged on the notion that controlled PAH sources produce emission and remain persistent over time (Marinaite et al., Citation2022). Due to the lack of PAH emission inventories in many countries, PAH diagnostics have been largely utilised to apportion the sources of PAH pollution (A. Kumar et al., Citation2019; Nowakowski et al., Citation2022; Oliveira et al., Citation2019). Table summarises the PAH diagnostic ratios used to identify atmospheric PAH sources.
Table 3. PAH diagnostic ratios utilised as source indicators
The use of diagnostic ratio was employed to study the sources of ambient PAHs in Central Europe (Dvorská et al., Citation2011). The study indicated that BaA/(BaA+Chr) and IcdP/(IcdP+BghiP) were capable of distinguishing between traffic and residential heating, while the ratio Ant/(Ant+Phe) was the least useful diagnostic ratio due to its low variability. To ascertain the sources of atmospheric PAHs in the Brisbane Metropolitan area, the PAH isomeric ratios were computed (Mishra et al., Citation2016). Based on the LWM/HMW and BaA (BaA+Chr) values, it was deduced that petrogenic and combustion sources were the predominant sources of PAH emission in the study area. Kural et al. (Citation2018) diagnosed the emission sources of atmospheric PAHs in an urban area in Istanbul using PAH diagnostic ratios and showed that diesel and gasoline emissions were the main sources of PAHs in the atmosphere, while W. Wang et al. (Citation2021) utilised BaA/(BaP+Chr) and IcdP(IcdP+BghiP) values to identify the sources of PAHs and showed that petroleum combustion and diesel emissions were key sources of PAHs in the atmosphere.
The sources of atmospheric PAHs in urban, industrial, and semi-urban areas in Malaysia were identified using the diagnostic ratio model (Jamhari et al., Citation2014). It was discovered that traffic emissions made a substantial contribution to atmospheric PAH pollution with negligible impact from coal burning and natural gas emissions. This suggests that the presence of atmospheric PAHs emanated from mixed sources. Similar findings were observed in other studies conducted in urban cities (Aslam et al., Citation2022; Kongpran et al., Citation2021; Marinaite et al., Citation2022).
The ratio of PAH congeners can be changed due to the reactivity of some PAHs with other atmospheric pollutants (ozone and nitrogen oxides), volatility, solubility, and reactivity differentials of PAH compounds (Pulster et al., Citation2019). Besides, decomposition may occur during the sampling process, which can amend the atmospheric PAH concentrations and their ratios. It is therefore admonished that more than one molecular diagnostic ratio should be utilised for source apportionment of PAHs. PAH congeners used for computing ratios should be of similar physicochemical properties. PAH ratios provide only a crude and qualitative source apportionment assessment and should be utilised in conjunction with more qualitative methods such as receptor models (Dvorská et al., Citation2011; Han et al., Citation2019).
3.3.2. Receptor models
The most widely utilised receptor models for determining possible sources of aerosols at local and regional levels include chemical mass balance (CMB) and multivariate models such as positive matrix factorisation (PMF), principal component analysis (PCA), factor analysis (FA)/multiple linear regression (MLR), and Unimix (D. Liu et al., Citation2017; Gupta et al., Citation2011). The receptor models make use of different mathematical algorithms to quantitatively identify PAHs to their sources by decomposing a data matrix into two or more matrices (Belis et al., Citation2014; Hopke, Citation2016).
The origins of atmospheric PAHs in Palm Beach County, Florida, were identified using Chemical Mass Balance, and it was observed that sugarcane combustion and mobile vehicular sources were the major contributors to atmospheric PAHs in the study area (Afshar-Mohajer et al., Citation2016). Notwithstanding, the PMF model was applied to diagnose the sources of PAH on an island in the middle of the Bohai Sea in China (Qu et al., Citation2022). The results suggested that coal combustion (43.1%), biomass burning (25.8%), vehicle exhaust (24.7%), and petroleum release (6.39%) accounted for the level of PM2.5-bound PAHs in the study area. In a similar study, an improved non-negative matrix model and PMF model apportioned PAHs to three sources including vehicle exhaust, biomass burning, and coal combustion (H. Liu et al., Citation2021). Biomass burning (48.6%) contributed the highest to the total PAH level, followed by coal combustion (34.6%) and vehicle exhaust emissions (16.8%).
On the contrary, three receptor models (FA/MLR, PMF, and Unimix models) were employed to diagnose the source of PAHs in the atmospheric environment of Alexandria in Egypt (Khairy & Lohmann, Citation2013). The study revealed that diesel vehicle exhaust emissions (36–49%), gasoline vehicle exhaust emissions (19–34%), and natural gas combustion (11–27%) were the dominant emission sources of PAHs in the study area. It was found that the PMF and Unimix models gave better results than the FA/MLR model. However, Ishtiaq et al. (Citation2021) diagnosed the origins of atmospheric PAHs in eight cities in Pakistan using PCA and MLR models and reported that biomass combustion, vehicular emissions, and diesel combustion in power generators were the potential PAH emissions sources in the study areas.
An assessment of findings about source apportionment of atmospheric PAHs indicates that emissions of PAHs into the atmosphere are from diverse sources. However, anthropogenic emission sources are the major determinants of PAH pollution in the atmosphere. Reliable source apportionment of atmospheric PAHs is essential for the development of reliable sustainable actions for PAH pollution (Y. Liu et al., Citation2015). The emission sources of atmospheric PAHs affect their characteristics, distribution patterns, and toxicity (B. K. Lee & Vu, Citation2010).
3.4. Behaviour, phases, and distribution pattern of atmospheric PAHs
The behaviour of PAHs in the atmosphere is governed by physical and chemical reactions, dry and wet settling, and photochemical modifications (Hussain & Medhi, Citation2018; Safo-Adu et al., Citation2014). In the atmosphere, PAHs can undergo photo-degradation and react with other pollutants, such as sulphur dioxide, nitrogen oxides, and ozone (Safo-Adu et al., Citation2014).
PAH isomers in the atmosphere subsist in the gaseous and particulate phases (S. N. Kumar et al., Citation2014). The concentration and phase distribution of PAHs in the atmosphere are governed by aerosol concentration, atmospheric conditions, and volatility of PAH compounds (Wu et al., Citation2019). Moreover, the partitioning of PAHs between the particulate and the gaseous phases greatly determines their fate and circulation in the atmosphere and the manner they enter the human body (Abdel-Shafy & Mansour, Citation2015). The elimination of PAHs from the atmosphere through dry and wet settling is controlled by their gas/particle partitioning (Abdel-Shafy & Mansour, Citation2015).
There are compositional differences between the particulate and gas phases of PAHs (Patel et al., Citation2020; Shimada et al., Citation2022; Yang et al., Citation2021). The differentials in individual PAH distributions in both gaseous and particulate phases are functions of their molecular weights and the number of aromatic rings (Abdallah & Atia, Citation2014). Ascertaining the relative distribution of individual PAHs is essential for assessing health risks and identifying pollution sources (Gupta et al., Citation2011). Table presents the distribution of PAHs in ambient air across some cities worldwide.
Table 4. Distribution of PAHs in ambient air across some cities worldwide
Most studies reported on the distribution of atmospheric PAHs in the particulate phase, while few studies reported on the levels of PAHs in both gas and particulate phases. About 38% and 27% of the studies reported on PAH distribution in PM2.5 and PM10, respectively, while 8% reported on PAH distribution in both PM10 and PM2.5. The total concentration of PAHs in PM2.5 was relatively higher, followed by PM10 and total suspended particles.
The research findings shown in Table indicate that the PAH composition of particle and gas phases diffesr. The LMW and HMW PAHs are dominant in the particulate and gas phases, respectively. LMW PAHs such as Nap, Ace, and Acy are emitted in the gaseous phase, while HMW PAHs such as Pyr, BaA, and BbF are usually emitted in the particulate phase (B. K. Lee & Vu, Citation2010). The results of a study conducted by Abdallah and Atia (Citation2014) in nine communities in Assiut, Egypt, revealed that LMW PAHs (Nap, Ace, Fl, Phe, and Ant) were predominant in the gaseous phase, accounting for more than 85% of the total PAHs in gas phase, while HMW PAHs (BbF, Chy, BghiP, BaP, and BkF) were most dominant in the particulate phase, accounting for more than 60% of total particulate PAH concentration. It was further reported that 4- to 6-ring PAHs dominated the particulate phase, while 2- to 3-ring PAHs were abundant in the gas phase with 80% and 87% contributions, respectively, to total atmospheric PAHs.
The gas–particle concentration and distribution of PAHs were determined in a traffic area in Giza, Egypt (Hassan & Khoder, Citation2012). The most dominant PAH in the particle and gas phases was BaP and Nap, respectively. Besides, the total mass concentration of PAHs was higher in the gas phase than in the particulate phase. On the contrary, the contribution of total carcinogenic PAH concentration to the total PAH level was higher in the particulate phase than in the gas phase. Furthermore, the study indicated that the distribution sequence and the relative contribution levels of aromatic rings to total PAHs in the particulate phase differed from that of the gas phase (Table ). The results of research conducted in upper north Thailand revealed that the average level of gas phase PAHs was two times higher than that of the particle phase during a haze period (Kongpran et al., Citation2021). In addition, LMW and HMW PAHs were detected only in the gaseous and particulate phases, respectively. However, MMW PAHs were found in both the gas and particulate phases. The findings presented in Table indicate that the concentration of PAHs in the gaseous-phase decreases with increasing molecular weight.
Atmospheric PAHs are mainly found bound to particulate matter, and when they are present in the gas phase, they have a duration of less than a day (World Health Organisation, Citation2021). According to Hassanvand et al. (Citation2015), more than 80% of particle-bound PAHs are associated with PM2.5, and PAH concentrations in inhalable particulates are highly dependent on fine particles. PAH concentrations increase effectively with increasing mass concentration of PM2.5 because combustion and high-temperature sources produce primarily fine particulates with high PAH content. Studies have confirmed this assertion as their findings showed that there was a strong correlation between PM2.5 and total PAH concentrations (A. Kumar et al., Citation2019; Ambade et al., Citation2022; Bootdee et al., Citation2016). Generally, a smaller particle has a higher PAH concentration due to the availability of a large surface area per unit, which makes them excellent adsorbent (A. Kumar et al., Citation2019).
On the contrary, a study in Pune, India, that quantified 13 PAHs in PM10 and PM2.5 particulates revealed that the concentration of individual PAHs measured in PM10 was higher than the levels measured in PM2.5 (Roy et al., Citation2019). However, the most dominant PAH in both size fractions of particulate matter was Nap, while IcdP was the least. It was further reported that the distribution pattern of PAHs based on aromatic rings (3R > 4R > 2R > 5R > 6R) and molecular weight (LMW>HMW>MMW) in both PM10 and PM2.5 were similar. Most findings showed that the distribution sequence of particulate PAHs in terms of molecular weight was HMW>MMW>LMW (A. Kumar et al., Citation2019; Ambade et al., Citation2022; Hassan & Khoder, Citation2012; Jang et al., Citation2013).
The distribution pattern of particulate PAHs based on the number of aromatic rings in an urban atmosphere along a major highway in Ghana was in the order 5R > 4R > 6R > 3R > 2R (Safo-Adu et al., Citation2014). Similar findings were observed by Y. Zhang et al. (Citation2019) and Hassan and Khoder (Citation2012) in the atmospheric environment of Wuhan, China, and Giza, Egypt, respectively (Table ). On the contrary, Arinaitwe et al. (Citation2012) found that the distribution pattern of particulate PAH concentrations in the air of the watershed of Lake Victoria area in East Africa was 3R > 2R > 4R > 5R > 6R. Moreover, Hassine et al. (Citation2014) reported that the distribution sequence of particulate PAHs in the atmosphere in Bizerte city Tunisia was 4R > 5R > 6R > 3R. This agreed with the findings observed at an urban site in Central Poland (Siudek & Frankowski, Citation2018).
Seasonality is a vital factor that controls PAH concentration in the atmosphere (Hussain & Medhi, Citation2018). To establish the seasonal variation in atmospheric PAHs at nine megacities in China, the concentration of PAHs in PM2.5 was determined from 2013 to 2014 (Li et al., Citation2019). The study revealed that the concentration of total PAHs increased from 10 to 80 folds in the winter rather than in the summer. Besides, 4- to 6-ring PAHs contributed more than 90% to total PAHs and were dominated by Flu, followed by BbF, Pyr, and Chr. Notwithstanding, Kalisa et al. (Citation2018) reported that the mean concentration of atmospheric PAHs was high in the dry season than in the wet season due to the rapidly particulate PAH deposition rate in the wet season, while Ramírez et al. (Citation2011) observed spatial and seasonal variability in PAH concentrations in Southern Europe with the highest PAH level found in the gas phase in the winter than summer and autumn.
The effect of atmospheric conditions on the seasonal variation of particulate PAHs at an urban site in Central Poland was investigated (Siudek & Frankowski, Citation2018). The results showed a decrease in PAH concentrations during the summer while higher concentrations of PAHsduring the winter. The dominant PAH congeners (pyrene, BaP, DahA, BkF, and BbF) contributed about 82.0% and 20.0% to the levels of PAHs in the cold and warm seasons, respectively. Furthermore, the outcome of a study that determined the seasonal variations in particulate-bound PAHs in three cities in the region of the Lower Sinos River Basin of Rio Grande do Sul revealed that the total PAH concentration in both fine and coarse particulates was higher in the winter than in the summer (Teixeira et al., Citation2013). Similar findings were reported by A. Kumar et al. (Citation2019), Y. Zhang et al. (Citation2019), Tsiodra et al. (Citation2021), and Aslam et al. (Citation2022). Chen et al. (Citation2018) observed a seasonal variation in atmospheric PAHs with the highest concentration obtained in the winter, followed by autumn, spring, and summer. However, the gas phase showed the opposite variation, with the highest and lowest concentrations obtained in the summer and winter, respectively.
The concentration of PAH congeners in the particulate phase increases in the winter with the lowering of temperature (Hussain & Medhi, Citation2018). Lower atmospheric mixing height as a result of a decrease in temperature impedes the mixing of atmospheric pollutants leading to a lower spread of PAH emission sources in the winter (Lakhani, Citation2012; S. N. Kumar et al., Citation2014). In contrast, higher wind speed and temperature in the summer cause higher spread and photo-dissociation of PAHs, resulting in a lower concentration of atmospheric PAHs (Masih et al., Citation2012; P. Li et al., Citation2015).
Atmospheric PAH levels are higher in urban centres than in rural areas. To characterise atmospheric PAHs at urban and rural sites in East India, the mass concentrations of PM2.5-bound PAH congeners were determined (A. Kumar et al., Citation2019). The PAH concentrations at urban sites were higher than at rural sites. IcdP was the predominant PAH, accounting for about 17% and 16% of the total PAHs at urban and rural sites, respectively. The distribution pattern of PAHs was in the sequence of 5R (46.2%)>6R (32.4%)>4R (12.4%)>3R (6.9%)>2R (1.9%) for both urban and rural sites. The higher level of atmospheric PAHs in urban than in rural areas has been attributed to the high population growth, huge vehicle emissions, and the prevalence of fossil fuel power stations (Kalisa et al., Citation2018). Again, the particulate-phase concentration of PAHs was determined in an urban city and rural site of Bizerte city, Tunisia (Hassine et al., Citation2014). Pyr and Flu were the most dominant PAHs, accounting for about 21% and 17% of the total PAHs. The levels of particulate PAHs in the urban city atmosphere were about eight times greater than those sampled at the rural site. The distribution of atmospheric PAHs was in the order 4R (51.7%)>5R (26.7)>6R (11.7%)>3R (9.8%).
The results of research conducted in a city centre and a university campus in Kumasi, Ghana, showed that the level of total atmospheric PAHs in the city centre was higher than that of the university campus (Bortey-Sam et al., Citation2015). The abundance of PAHs in the ambient air at the city centre and the university campus was BghiP (29%) and Phe (21%) respectively. HMW PAHs were highly distributed in the city centre, indicating that vehicle emissions and biomass combustion accounted for the ambient air PAH pollution in the city centre. Sánchez-Piñero et al. (Citation2021) quantified 15 PAH compounds and revealed that BbF and BeP were the most abundant PAHs in inhalable particulates (PM10). In addition, the distribution of PAH congeners followed the sequence BghiP>Chy> IP> BaP>BkF >Ft > Pyr>BaA >Phe>DahA, Ace> Fl, Ant.
A study that determined 20 PAHs in PM2.5 in an urban city in the Czech Republic showed that Flu (4.09 ng/m3) and Pyr (3.75 ng/m3) were the predominant PAHs (Polachova et al., Citation2019). It was found that the BaP level exceeded the European average emission limit of 1 ngm−3. Other studies carried out in cities recorded high levels of BaP in the atmosphere, which exceeded the European average emission limit (A. Kumar et al., Citation2019; Ambade et al., Citation2022; Kalisa et al., Citation2018; Polachova et al., Citation2019; Safo-Adu et al., Citation2014), indicating that the human population in these cities stands a chance of getting cancer upon long-term exposure.
The analysis of research findings presented in Table indicates that the mass concentrations and distribution of atmospheric PAHs differed from one geological location to another. As a result, the most dominant PAHs in the atmosphere also varied. The outcome of a study carried out by Garrido et al. (Citation2014) in Europe confirmed this assertion as it was found that the mass concentrations of total atmospheric PAHs varied across European cities. Atmospheric PAHs vary significantly as a function of their emission sources (Jamhari et al., Citation2014). It is said that a more restricted control of particulate PAHs pollution is needed to improve environmental air quality (Mesquita et al., Citation2014).
3.5. Health Implications of exposure to PAHs
PAHs are linked to different short-term symptoms such as nausea, vomiting, eye irritation, and diarrhoea, and long-term health effects including a decrease in the number of red blood cells, liver and kidney damage, and an increased risk of asthma, cardiovascular diseases, and lung cancer in infants, children, the elderly, and pregnant women (Kim et al., Citation2013; World Health Organisation, Citation2021). The carcinogenic ability of PAHs increases with increasing molecular weight. HMW PAHs are more carcinogenic, while LMW PAHs are characterised by increased toxicity (Kim et al., Citation2013). PAHs have been categorised based on their toxic abilities as probable (2A) and possible (2B) carcinogens, which include BaP, DahA, BaA, Chr, BbF, BkF, BghiP, and IcdP (International Agency for Research on Cancer, Citation2010). BaP has been recognised as an indicator of the whole group of PAHs being extremely carcinogenic (L. Singh & Agarwal, Citation2020).
The carcinogenic five- and six-ring PAH congeners are dominantly associated with particulate matter and, depending on meteorological factors, may subsist for days and circulate over long distances (Hussain & Medhi, Citation2018; S. N. Kumar et al., Citation2014; Yang et al., Citation2021). The findings of a study carried out by Bootdee et al. (Citation2016) in the city of Chiang Mai in Thailand revealed that fine particulate concentrations moderately correlated with carcinogenic PAHs (r = 0.618), indicating that carcinogenic compounds are dominant in fine particulates. The risk of exposure to carcinogenic PAHs depends on the form by which the PAH enters the body. Particulate phase PAHs can move into the lungs and deploy their toxic impacts over longer periods than gaseous PAHs (Hassan & Khoder, Citation2012). In contrast, PAHs present in the gas phase can reach the alveolar epithelium through inhalation and rapidly transfix into the circulatory system (W. Wang et al., Citation2021).
Quantitatively, humans are exposed to pollutants in the environment through three main pathways. These include inhalation, dermal absorption, and ingestion (Roy et al., Citation2019). Inhalation has been considered a significant pathway for human exposure to atmospheric PAHs (Ramírez et al., Citation2011).
3.6. Health risk assessment of PAHs exposure
The incremental lifetime lung cancer risk (ILCR) model has been utilised to estimate the level of harm to human health caused by exposure to atmospheric PAHs through the inhalation route (Cheng et al., Citation2022). ILCR depends on the toxicity equivalent factors, inhalation unit risks, and the number of PAHs (Sánchez-Piñero et al., Citation2021). To estimate the ILCR level, the benzo(a)pyrene equivalence (BaPeq) toxicity of individual PAH is first determined by multiplying individual PAH concentrations with the corresponding toxic equivalent factor (TEF) as presented in EquationEq. 1(1)
(1) (B. P. Singh et al., Citation2023).
where Cn = individual PAH concentration in the complex mixture, TEFn = toxic equivalent factor of individual PAH, and BaPeq = Benzo[a]pyrene equivalence. The toxic equivalent factor represents the toxicity of an individual PAH compound relative to BaP.
Second, total benzo(a)pyrene equivalence toxicity concentration, also known as the toxicity equivalent concentration (TEQ), is computed. TEQ is the sum of the product of individual concentrations of PAHs and the corresponding TEF as shown in EquationEq. 2(2)
(2) .
where Cn = individual PAH concentration in the complex mixture, TEFn = Toxic Equivalent Factor of individual PAH, and k = the total number of PAH compounds.
Thirdly, ILCR can be estimated by multiplying the toxic equivalence concentration (TEQ) by the inhalation unit risk (IUR) as presented in EquationEq. 3(3)
(3) (Ramírez et al., Citation2011).
Where IUR represents inhalation unit risk, which describes the theoretical upper limit possibility of contracting cancer calculated for daily exposure to BaP concentration in air of 1.0 μgm−3 for a 70-year average life span (United States Environmental Protection Agency, Citation2019). Different IUR values have been used in the scientific literature to estimate the inhalation cancer risk. The IUR values recommended by World Health Organisation (Citation2021), United States Environmental Protection Agency (Citation2019), and the Office of Environmental Health Hazard Assessment (Citation2015) are 8.7 × 10−5, 6.0 × 10−7, and 1.1 × 10−6 m3/mg, respectively, over a lifetime of 70 years of PAH exposure.
Alternatively, the ILCR can also be determined by multiplying the lifetime average daily dose (LADD) by the cancer slope factor (CSF) as presented in EquationEq. 4(4)
(4) .
The LADD is computed using EquationEq. 5(5)
(5)
where LADD is the amount of intake per kilogram of body weight per day of PAHs (Bortey-Sam et al., Citation2015), Cs is the mean concentration of PAH (ng/m3), IR is the intake rate, ET is the exposure time, EF is the exposure frequency, ED represents the exposure duration, CF is the unit conversion factor (CF = 10−6), BW is the average body weight, and AT is the average time (Ambade et al., Citation2022; Bortey-Sam et al., Citation2015; Chen et al., Citation2018).
The US EPA threshold limit for assessing lifetime lung cancer risk over 70 years is 1 × 10−6. ILCR less than or equal to 10−6 denotes negligible or virtual safety under most regulatory programmes (Sánchez-Piñero et al., Citation2021). ILCR between 10−6 and 10−4 indicates a potential risk, while ILCR greater than 10−4 indicates a high risk (Cao et al., Citation2015).
Globally, extensive epidemiological studies have been carried out concerning the health risk of exposure to airborne PAHs. Most of such studies analysed the health risk exposure to the 16 US EPA priority PAHs (Ambade et al., Citation2022; Ana et al., Citation2012; Cheng et al., Citation2022; Oliveira et al., Citation2019; S. N. Kumar et al., Citation2014). However, few studies focused on health risk assessment of exposure to PAHs more and in some cases less than the 16 US EPA priority particulate-bound PAHs (Alam et al., Citation2013; Bootdee et al., Citation2016; Polachova et al., Citation2019; Sánchez-Piñero et al., Citation2021). Table presents health risk exposure levels to atmospheric PAHs in some cities worldwide.
Table 5. Health risk exposure levels to atmospheric PAHs in some cities worldwide
Few studies carried out to appraise the health risk of exposure to atmospheric PAHs in cities found the ILCR level to be lower than the acceptable limit of 10−6 (Table ). The outcome of research conducted in Malaysia revealed that the estimated ILCR of exposure to airborne PAHs was negligible at all sampling sites for all age-specific groups (Jamhari et al., Citation2014). In addition, the total BaPeq concentration accounted for more than 50% of the potential health risk, indicating that the total BaPeq concentration provides a better estimation of carcinogenicity activities (Jamhari et al., Citation2014).
In a similar study that investigated the toxicity of PAHs in PM2.5 in rural and urban areas in East India (A. Kumar et al., Citation2019), it was found that DahA contributed 46% to total PAH carcinogenicity in both rural and urban areas. Besides, the ILCR values were below the acceptable limit range of 10−6 to 10−4. Similar findings were observed in a study that assessed the health risk of inhabitants’ exposure to particulate PAHs at six locations in Curitiba city in Brazil (Froehner et al., Citation2011) and Tehran megacity in Iran (Hoseini et al., Citation2015). Furthermore, sensitivity analysis showed that the BaP level and the cancer slope factor accounted for the ILCR value (Hoseini et al., Citation2015).
PAHs in three different size fractions (PM1, PM2.5, and PM10) of particulate matter were quantified, and their health risks of exposure were computed in Bab Ezzouar, Algeria (Teffahi et al., Citation2021). The mean ILCR of exposure to PAHs in PM1 (2 × 10−6) was higher than that of PM2.5 (8.87 × 10−7) and PM10 (1 × 10−6), respectively. The cancer risks of public exposure to PAHs in PM2.5 and PM10 were relatively low. However, the ILCR for exposure to PAHs in PM1 was twice the acceptable limit. Cancer risk of exposure to PAHs in ultrafine was higher than in fine and inhalable particulates. Y. Wang et al. (Citation2022) evaluated the health risk of exposure to PAHs in total suspended particles in Vladivostok, a port city in Russia, and obtained ILCR values that ranged from 1.0 × 10−7 to 1.5 × 10−5, indicating a potential carcinogenic risk. Similar findings were reported in studies conducted in other cities (Byambaa et al., Citation2019; Ishtiaq et al., Citation2021; Kalisa et al., Citation2018).
From Table , more than 50% of studies conducted reported that exposure to atmospheric PAHs potentially posed health risks. For instance, Ambade et al. (Citation2022) found that the ILCR levels of exposure to atmospheric PAHs at all sampling sites in Central East India ranged from 2.6 × 10−5 to 7.6 × 10−5, which were above the acceptable limit of 10−6 but below the tolerable level of 10−4. Furthermore, the outcome of a study conducted by Bortey-Sam et al. (Citation2015) revealed that the ILCR of exposure to airborne PAHs at a university campus and a city centre in Kumasi, Ghana, was within a tolerable range of 10−6 to 10−4. Besides, it was found that the toxicity equivalence concentration of airborne PAHs in the city centre was 18 times higher than that obtained on the university campus. N. Zhang et al. (Citation2022) found that the average inhalation carcinogenic risk of PAH exposure (1.6 × 10−5) in Tianjin, China, was above the acceptable risk level of 1 × 10−6 but below the tolerance limit of 1 × 10−4, indicating the potential of atmospheric PAHs to pose a cancer risk.
The results of most studies conducted in cities showed that the health risk of exposure to atmospheric PAHs was high (Alamri et al., Citation2021; Fakinle et al., Citation2022; Khairy & Lohmann, Citation2013). Sánchez-Piñero et al. (Citation2021) found that the ILCR (2.4 × 10−4) of exposure to airborne PAHs in a Southwestern European coastal city exceeded the risk level of 1 × 10−4 when inhalation unit risk of 8.7 × 10−5 recommended by the WHO (Citation2000) was utilised to calculate the ILCR. Nonetheless, the lung cancer risk was decreased to a potential cancer risk when the inhalation unit risk values of 6.0 × 10−7 (US EPA, Citation2019) were used to compute the ILCR. Besides, carcinogenic PAHs (BbF, Chr, IP, BaP, BkF, BaA, and DahA) and non-carcinogenic PAHs (Ace, Fl, Phe, Ant, Flu, Pyr, BeP, and BghiP) concentrations contributed about 58–60% and 40–42% to PM10-bound PAHs, respectively. Ramírez et al. (Citation2011) applied the WHO unit risk (UR) to estimate lifetime cancer risk of exposure to atmospheric PAHs in Southern Europe and observed that the total estimated average lifetime lung cancer risk (1.2 × 10−4) was higher than 1 × 10−6. In addition, the contribution of gas-phase PAHs to the total BaPeq value was between 34% and 86%, suggesting that the contribution of gas-phase PAHs to the health risk of exposure to airborne PAHs is significant and cannot be ignored.
The cancer risks of PAHs in fine particulates were investigated in an urban area in Wuhan, Central China (Y. Zhang et al., Citation2019). It was observed that the overall ILCR through inhalation exposure was 3.03 × 10−4. Vehicular emissions, biomass burning, and petrogenic sources contributed 57.1%, 31.0%, and 11.9% to the total risk level with carcinogenic risk contributions of 1.6 × 10−4, 9.6 × 10−5, and 3.6 × 10−5, respectively. This implies that PM2.5-PAHs posed a cancer risk to the inhabitants of Wuhan. Safo-Adu et al. (Citation2014) computed ILCR due to hawkers’ exposure to PAHs in PM10 through inhalation to be 71 × 10−3, which is significantly higher than the acceptable risk limit. This aligns with findings observed in a study conducted in Lahore city, Pakistan (Aslam et al., Citation2022) and Upper North Thailand (Kongpran et al., Citation2021).
A comparative analysis of the findings of research conducted by A. Kumar et al. (Citation2019) in East India and that carried out by Abdallah and Atia (Citation2014) indicated that the health risk of exposure to atmospheric PAHs was high in urban areas than in rural and suburban areas. In contrast, Ambade et al. (Citation2022) reported that the ILCR of exposure to PAHs in fine particles through inhalation at the industrial site was highest (60.97 × 10−6), followed by urban (15.99 × 10−6) and semi-urban (14.16 × 10−6) areas.
In summary, most studies determined the levels and distribution of PAHs in fine particulates (PM2.5), inhalable particulates (PM10) and total suspended particulates (TSP). However, few studies constituting about 8% concurrently determined the distribution of PAHs in PM2.5 and PM10. Again, the majority of studies reported on the distribution of atmospheric PAHs in the particulate phase, while few studies reported on the levels of PAHs in both gas and particulate phases. This finding is in parallel with a review carried out by Hussain and Medhi (Citation2018), which found that research has mostly emphasised the estimation of particulate-bound PAHs, while the gas phase has been overlooked. Atmospheric PAHs were widely distributed in gas and particulate phases and emanated from mixed sources. However, anthropogenic emission sources constituted the primary source of PAHs in ambient air. The emission sources of atmospheric PAHs affected their characteristics, distribution sequence, and toxicity. The distribution sequence of PAHs in particulate and gas phases was HMW>MMW>LMW PAHs and LMW>MMW>HMW PAHs, respectively. LMW PAHs (Nap, Ace, Fl, Phe, and Ant) accounted for 85% of the total PAHs in the gas phase, while HMW PAHs (BbF, Chy, BghiP, BaP, and BkF) contributed 60% to the total particulate PAHs. In addition, 4–6 rings PAH dominated the particle phase, whilw 2–3 rings PAH was predominant in the gas phase.
Epidemiological studies conducted in most cities worldwide showed that the cancer risk level due to atmospheric PAH exposure was higher than the US EPA threshold value of 10−6. Besides, the BaP level exceeded the European Commission's permissible limit of 1 ng/m3 in most cities. Similar findings were observed in other reviews that appraised the health risk levels of exposure to PAHs in atmospheric air (Hussain & Medhi, Citation2018; Kim et al., Citation2013; Oliveira et al., Citation2019). The total BaPeq concentration accounted for 50% of the potential health risks of PAHs exposures, while gas phase PAHs contributed 34% to 86% to BaPeq concentration. The contribution of gas-phase PAHs to the health risk of exposure to airborne PAHs is significant and should be ignored.
4. Conclusions
The data retrieved from available literature on sources, distribution, and health risk exposure levels varied by geographical location and may not represent the levels of a specific country or the entire world. This review also considered various air sampling approaches, measurement techniques, and temporal coverage. This could have caused variability in the data obtained. Despite these limitations, the study used a narrative review approach to synthesise research findings in the literature on the sources, distribution patterns, and health risk exposure levels of atmospheric PAHs.
The main findings of the study are summarised below:
The majority of studies looked at PAHs in the particulate phase of the atmosphere, while only a few looked at PAHs in both the particulate and gas phases. About 38% and 27% of the studies reported on the distribution of PAHs in PM2.5 and PM10, respectively, while the remaining 8% reported on the distribution of PAHs in both PM10 and PM2.5. PM2.5 had the highest total PAH concentration, followed by PM10 and total suspended particles.
Analysis of the research findings revealed that atmospheric PAHs in urban areas were approximately eight times higher than in rural environments and came from a variety of sources. However, vehicle exhaust and industrial emissions were the primary sources, accounting for 60–80% of PAHs in the atmosphere.
In most cities, higher levels of atmospheric PAHs were reported in the winter than in the summer, autumn, and spring, implying that seasonality is an important factor in controlling atmospheric PAHs.
PAHs were distributed in the following order in the particulate and gas phases: HMW>MMW>LMW PAHs and LMW>MMW>HMW PAHs. LMW PAHs were found to account for 85% of the total PAHs in the petrol phase, while HMW PAHs contributed roughly 60% of total particulate PAHs. Furthermore, 4–6 rings PAHs dominated the particulate phase, while 2–3 rings PAHs were abundant in the petrol phase, accounting for 80% and 87% of the total atmospheric PAHs, respectively.
According to the studies, carcinogenic PAHs (BbP, Chr, IP, BaP, BkF, BaA, and DahA) and non-carcinogenic PAHs (Ace, Fl, Phe, Ant, Flu, Pyr, BeP, and BghiP) contributed about 58–60 and 40–42%, respectively, to particulate PAHs.
The BaP concentration level in most cities’ ambient air exceeded the European Commission’s threshold limit of 1 ng/m3, and the incremental lung cancer risk level (1.2 × 10−1−7.6 × 10−5) of inhaling atmospheric PAHs was higher than the permissible value of 10−6.
In infants, children, and the elderly, chronic exposure to PAHs in the environment reduced the number of red blood cells and increased the risk of asthma, cardiovascular disease, and lung cancer. The cancer risk from PAH exposure was higher in ultrafine (21 × 10−6) than in fine (8.87 × 10−7) and inhalable particulates (1 × 10−6).
More research is needed to investigate the distribution and health risks of exposure to atmospheric PAHs in both particulate and gas phases in urban cities. To improve environmental air quality, more stringent control of particulate PAH pollution is required. Furthermore, the adoption of stricter emission standards, cleaner energy sources, and more efficient industrial technology will aid in the reduction of particulate PAH pollution and its associated health consequences in cities around the world.
Acknowledgments
The authors thank Editor-in-Chief and anonymous reviewers for their valuable and constructive suggestions to improve the quality of the paper
Disclosure statement
No potential conflict of interest was reported by the author(s).
Additional information
Notes on contributors

Godfred Safo-Adu
Godfred Safo-Adu is a Lecturer in the Department of Environmental Science at the Faculty of Science Education of the University of Education, Winneba in Ghana. Currently, he is pursuing Ph.D. in Environmental Engineering and Management at the University of Energy and Natural Resources, Sunyani in Ghana. With his background in Chemistry and Environmental Science, he worked as a Process Plant Supervisor in an Integrated Oily Waste Treatment Facility in the Western Region of Ghana for over eight years. He has published in reputable refereed journals locally and at the international level in Environmental Science. His research interest includes groundwater quality modelling, aerosol science, design of indigenous techniques for wastewater recycling, environmental epidemiology, waste-to-energy, soil chemistry, and toxicology.
References
- Abdallah, M. A., & Atia, N. N. (2014). Atmospheric concentrations, gaseous – particulate distribution, and carcinogenic potential of polycyclic aromatic hydrocarbons in Assiut, Egypt. Environmental Science and Pollution Research, 21(13), 8059–29. https://doi.org/10.1007/s11356-014-2746-6
- Abdel-Shafy, H. I., & Mansour, M. S. M. (2015). A review on polycyclic aromatic hydrocarbons: Source, environmental impact, effect on human health and remediation. Egyptian Journal of Petroleum, 25(1), 0–17.
- Afshar-Mohajer, N., Wilson, C., Wu, C., Stormer, J. E., Wilson, C., Wu, C., Stormer, J. E., & County, B. (2016). Source apportionment of atmospheric polycyclic aromatic hydrocarbons (PAHs) in palm beach. Journal of the Air & Waste Management Association, 66(3), 377–386. https://doi.org/10.1080/10962247.2016.1138902
- Alam, M. S., Delgado-Saborit, J. M., Stark, C., & Harrison, R. M. (2013). Using atmospheric measurements of PAH and quinone compounds at the roadside and urban background sites to assess sources and reactivity. Atmospheric Environment, 77, 24–35. https://doi.org/10.1016/j.atmosenv.2013.04.068
- Alamri, S. H., Ali, N., Mohammed, H., Ali, S., Rashid, M. I., Rajeh, N., Mohammed, M., Qutub, A., & Malarvannan, G. (2021). Polycyclic aromatic hydrocarbons in indoor dust collected during the COVID-19 pandemic lockdown in Saudi Arabia: Status, sources and human health risks. International Journal of Environmental Research and Public Health, 18(5), 1–13. https://doi.org/10.3390/ijerph18052743
- Alani, R., Zhao, S., Liu, X., Akinrinade, O., Agunbiade, F., Ayejuyo, O., & Zhang, G. (2021). Concentrations, profiles and exposure risks of polycyclic aromatic hydrocarbons (PAHs) in passive air samples from Lagos, Nigeria. Atmospheric Pollution Research, 12(9), 101162. https://doi.org/10.1016/j.apr.2021.101162
- Ambade, B., Kumar, A., Kumar, A., & Sahu, L. K. (2022). Temporal variability of atmospheric particulate-bound polycyclic aromatic hydrocarbons (PAHs) over central East India: Sources and carcinogenic risk assessment. Air Quality, Atmosphere, & Health, 15(1), 115–130. https://doi.org/10.1007/s11869-021-01089-5
- Ana, G. R. E. E., Sridhar, M. K. C., Emerole, G. O., Sridhar, M. K. C., & Polycyclic, G. O. E. (2012). Polycyclic aromatic hydrocarbon burden in ambient air in selected Niger Delta communities in Nigeria. Journal of the Air & Waste Management Association, 2247(62), 1–24. https://doi.org/10.1080/10473289.2011.628900
- Angelevska, B., Atanasova, V., & Andreevski, I. (2021). Urban air quality guidance based on measures categorisation in road transport. Civil Engineering Journal, 7(2), 253–266. https://doi.org/10.28991/cej-2021-03091651
- Arinaitwe, K., Kiremire, B. T., Muir, D. C. G., Fellin, P., Li, H., Teixeira, C., & Mubiru, D. N. (2012). Atmospheric concentrations of polycyclic aromatic hydrocarbons in the Watershed of Lake Victoria, East Africa. Environmental Science and Technology, 46(21), 11524–11531. https://doi.org/10.1021/es302238w
- Aslam, R., Sharif, F., Baqar, M., & Shahzad, L. (2022). Source identification and risk assessment of polycyclic aromatic hydrocarbons (PAHs) in air and dust samples of Lahore City. Scientific Reports, 12(2459), 1–15. https://doi.org/10.1038/s41598-022-06437-8
- Asril, M., Sugiarto, S., & Zurfi, A. (2023). Airborne microbial quality assessment in the educational buildings during COVID-19 pandemic. Civil Engineering Journal, 9(1), 114–126. https://doi.org/10.28991/CEJ-2023-09-01-09
- Bai, L., & Li, C. (2022). Investigation of Indoor polycyclic aromatic hydrocarbons. Buildings, 12(2), 153. https://doi.org/10.3390/buildings12020153
- Belis, C., Larsen, B., Amato, F., Haddad, I., Favez, O., Harrison, R., & Hopke, P. (2014). European guide on air pollution source apportionment with receptor models. European Commission, 15(4), 1–88.
- Bessagnet, B., & Allemand, N. (2020). Review on black carbon and polycyclic aromatic hydrocarbon emission reductions induced by particulate matter emission abatement techniques. TFTEI Technology Scientific.
- Bootdee, S., Chantara, S., & Prapamontol, T. (2016). Determination of PM2.5 and polycyclic aromatic hydrocarbons from incense burning emission at shrine for health risk assessment. Atmospheric Pollution Research, 7(4), 680–689. https://doi.org/10.1016/j.apr.2016.03.002
- Bortey-Sam, N., Ikenaka, Y., Akoto, O., Nakayama, S. M. M., Yohannes, Y. B., Baidoo, E., Mizukawa, H., & Ishizuka, M. (2015). Levels, potential sources and human health risk of polycyclic aromatic hydrocarbons (PAHs) in particulate matter (PM10) in Kumasi, Ghana. Environmental Science and Pollution Research, 22(13), 9658–9667. https://doi.org/10.1007/s11356-014-4022-1
- Byambaa, B., Yang, L., Matsuki, A., Nagato, E. G., Gankhuyag, K., Chuluunpurev, B., Banzragch, L., Chonokhuu, S., Tang, N., & Hayakawa, K. (2019). Sources and characteristics of polycyclic aromatic hydrocarbons in ambient total suspended particles in Ulaanbaatar City, Mongolia. International Journal of Environmental Research and Public Health, 16(3), 442. https://doi.org/10.3390/ijerph16030442
- Cao, S., Duan, X., Zhao, X., Wang, B., Ma, J., Fan, D., Sun, C., He, B., Wei, F., & Jiang, G. (2015). Health risk assessment of various metals (loid) via multiple exposure pathways on children living near a typical lead-acid battery plant, in China. Environmental Pollution, 200, 16–23. https://doi.org/10.1016/j.envpol.2015.02.010
- Cheewaphongphan, P., Garivait, S., & Pongpullponsak, A. (2011). Inventory of pollution from rice field residue open burning based on field survey. Proceedings of the 2nd Conference in Environmental Science and Technology, Singapore, 6, 93–97.
- Cheng, K., Li, J., Wang, Y., Ji, W., & Cao, Y. (2022). Characterisation and risk assessment of airborne polycyclic aromatic hydrocarbons from open burning of municipal solid waste. Frontiers in Environmental Science, 10, 1–10. https://doi.org/10.3389/fenvs.2022.861770
- Chen, P., Kang, S., Li, C., Li, Q., Yan, F., Guo, J., Ji, Z., Zhang, Q., Hu, Z., Tripathee, L., & Sillanpää, M. (2018). Source apportionment and risk assessment of atmospheric polycyclic aromatic hydrocarbons in Lhasa, Tibet, China. Aerosol and Air Quality Research, 18(5), 1294–1304. https://doi.org/10.4209/aaqr.2017.12.0603
- Cui, S., Lei, R., Wu, Y., Huang, D., Shen, F., Wang, J., Qiao, L., Zhou, M., Zhu, S., Ma, Y., & Ge, X. (2020). Characteristics of black carbon particle-bound Polycyclic aromatic hydrocarbons in two sites of Nanjing and Shanghai, China. Atmosphere, 11(2), 1–14. https://doi.org/10.3390/atmos11020202
- Dvorská, A., Lammel, G., & Klánová, J. (2011). Use of diagnostic ratios for studying source apportionment and reactivity of ambient polycyclic aromatic hydrocarbons over Central Europe. Atmospheric Environment, 45(2), 420–427. https://doi.org/10.1016/j.atmosenv.2010.09.063
- Eriksson, A. C., Nordin, E. Z., Bergvall, E., Pettersson, E., Swietlicki, C., Boman, R., Westerholm, C., Pagels, J. H., & Pagels, J. H. (2014). Particulate PAH emissions from residential biomass combustion: Time-resolved analysis with aerosol mass spectrometry. Environmental Science and Technology, 48(12), 7143–7150. https://doi.org/10.1021/es500486j
- Fakinle, B., Leke, E., Ike-Ojukwu, C., Olubukola, O., Abiodun, O., Winner, F., Olawale, D., & Aremu, C. O. (2022). Quantification and health impact assessment of polycyclic aromatic hydrocarbons (PAHs) emissions from crop residue combustion. Helion, 8(3), e09113. https://doi.org/10.1016/j.heliyon.2022.e09113
- Froehner, S., Maceno, M., Machado, K. S., & Grube, M. (2011). Health risk assessment of inhabitants exposed to PAHs particulate matter in the air. Journal of Environmental Science and Health, 4529(8), 817–823. https://doi.org/10.1080/10934529.2011.579843
- Gao, H., Liu, N., Guan, W., Liu, N., & Jiang, S. (2017). Pollution characteristics and potential ecological risk assessment of polycyclic aromatic hydrocarbons in wastewater irrigated soil. Journal of Environmental Health, 79(9), 1–6.
- Garrido, A., Jimenez-Guerrero, P., & Ratola, D. (2014). Levels, trends and health concerns of atmospheric PAHs in Europe. Atmosphere, 99, 99. https://doi.org/10.1016/j.atmosenv.2014.10.011
- Ghosal, D., Ghosh, S., Dutta, T. K., & Ahn, Y. (2016). The current state of knowledge in microbial degradation of polycyclic aromatic hydrocarbons (PAHs): A review. Frontiers in Microbiology, 7. https://doi.org/10.3389/fmicb.2016.01369
- Gibergans-Baguena, J., Hervada-Sala, C., & Jarauta-Bragulat, E. (2020). The quality of urban air in Barcelona: A new approach applying compositional data analysis methods. Emerging Science Journal, 4(2), 113–121. https://doi.org/10.28991/esj-2020-01215
- Gilio, A. D., Palmisani, J., Trizio, L., Saracino, G., Giua, R., & de Gennaro, G. (2020). Total p-PAH levels nearby a complex industrial area: A tailored monitoring experiment to assess the impact of emission sources. Atmosphere, 11(5), 1–13. https://doi.org/10.3390/atmos11050469
- Guerreiro, C. B. B., Foltescu, V., & Leeuw, F. D. (2014). Air quality status and trends in Europe. Atmospheric Environment, 98, 376–384. https://doi.org/10.1016/j.atmosenv.2014.09.017
- Gupta, S., Kumar, K., Srivastava, A., Srivastava, A., & Jain, V. K. (2011). Size distribution and source apportionment of polycyclic aromatic hydrocarbons in aerosol particle samples from the atmospheric environment of Delhi, India. The Science of the Total Environment, 409(22), 4674–4680. https://doi.org/10.1016/j.scitotenv.2011.08.008
- Gurjar, B. R., Ravindra, K., & Nagpure, A. S. (2016). Air pollution trends over Indian megacities and their local-to-global implications. Atmospheric Environment.
- Han, B., Ding, X., Bai, Z., Kong, S., & Guo, G. (2011). Source analysis of particulate matter associated polycyclic aromatic hydrocarbons (PAHs) in an industrial city in northeastern China. Journal of Environmental Monitoring, 13(2597), 2597–2604. https://doi.org/10.1039/c1em10251f
- Han, B., You, Y., Liu, Y., Xu, J., Zhou, J., Zhang, J., Niu, C., Zhang, N., & He, F. (2019). Inhalation cancer risk estimation of source-specific personal exposure for particulate matter–bound polycyclic aromatic hydrocarbons based on positive matrix factorisation. Environmental Science and Pollution Research.
- Hassan, S. K., & Khoder, M. I. (2012). Gas–particle concentration, distribution, and health risk assessment of polycyclic aromatic hydrocarbons at a traffic area of Giza, Egypt. Environmental Monitoring and Assessment, 184(6), 3593–3612. https://doi.org/10.1007/s10661-011-2210-8
- Hassanvand, M., Nadda, K., Faridi, S., Nabizadeh, R., Sowlat, M. H., Momeniha, F., Gholampour, A., Arhami, M., Kashani, H., Zare, A., Niazi, S., Rastkari, N., Nazmara, S., Ghani, M., & Yunesian, M. (2015). Science of the total environment characterization of PAHs and metals in indoor/outdoor PM10/PM2.5/PM1 in a retirement home and a school dormitory. The Science of the Total Environment, 528, 100–110. https://doi.org/10.1016/j.scitotenv.2015.05.001
- Hassine, S. B., Hammami, B., Ameur, B., Megdiche, E., Barhoumi, B., & Driss, M. (2014). Particulate Polycyclic Aromatic Hydrocarbons (PAH) in the atmosphere of Bizerte City, Tunisia. Bull. Environmental Contamination and Toxicology, 93(1), 375–385.
- Hopke, P. K. (2016). Review of receptor modelling methods for source apportionment. Journal of Air and Waste Management and Association, 66(3), 237–259. https://doi.org/10.1080/10962247.2016.1140693
- Hoseini, M., Yunesian, M., & Nabizadeh, R. (2015). Characterisation and risk assessment of polycyclic aromatic hydrocarbons (PAHs) in urban atmospheric Particulate of Tehran, Iran. Environmental Science and Pollution Research.
- Hu, S., Herner, J. D., Robertson, W., Kobayashi, R., Chang, O., Huang, S., Zielinska, B., Rieger, P., Collins, J. F., Rieger, P., Huai, T., & Ayala, A. (2013). Emissions of polycyclic aromatic hydrocarbons (PAHs) and nitro-PAHs from heavy-duty diesel vehicles with DPF and SCR. Journal of Air and Waste Management Association, 2247(8), 984–996. https://doi.org/10.1080/10962247.2013.795202
- Hussain, K., & Medhi, S. (2018). Polycyclic aromatic hydrocarbons in aerosol and its health implications: A review. Journal of Environmental Research and Development, 12(4), 483–492.
- International Agency for Research on Cancer. (2010) . IARC monographs on the evaluation of carcinogenic risks to humans. WHO Publishers.
- Ishtiaq, J., Hussain, J., Azeem, W., Hamid, N., Jamshed, M., Chaudhry, I., Shahnawaz, M., Nasir, J., Hussain, S., Rizvi, H., Chakraborty, P., Li, J., & Zhang, G. (2021). Atmospheric polycyclic aromatic hydrocarbons (PAHs) at urban settings in Pakistan: Spatial variations, sources and health risks. Chemosphere, 274, 1–10. https://doi.org/10.1016/j.chemosphere.2021.129811
- Jamhari, A., Sahani, M., Talib, M., Meng, K., Seng, H., Khan, F., & Mohd, N. (2014). Concentration and source identification of polycyclic aromatic hydrocarbons (PAHs) in PM10 of urban, industrial and semi-urban areas in Malaysia. Atmospheric Environment, 86, 16–27. https://doi.org/10.1016/j.atmosenv.2013.12.019
- Jang, E., Alam, M. S., & Harrison, R. M. (2013). Source apportionment of polycyclic aromatic hydrocarbons in urban air using positive matrix factorisation and spatial distribution analysis. Atmospheric Environment, 79, 271–285. https://doi.org/10.1016/j.atmosenv.2013.06.056
- Jimenez, J., Farias, O., Quiroz, R., & Yañez, J. (2017). Emission factors of particulate matter, polycyclic aromatic hydrocarbons, and levoglucosan from wood combustion in south-central Chile. Journal of Air and Waste Management Association, 67(7), 806–813. https://doi.org/10.1080/10962247.2017.1295114
- Kalisa, E., Nagato, E. G., Bizuru, E., Lee, K. C., Tang, N., Pointing, S. B., Hayakawa, K., Archer, S. D. J., & Lacap-Bugler, D. C. (2018). Characterisation and risk assessment of atmospheric fine and coarse particulate-bound PAHs and NPAHs in Rwanda, Central-East Africa. Environmental Science and Technology, 52(21), 12179–12187. https://doi.org/10.1021/acs.est.8b03219
- Khairy, M., & Lohmann, R. (2013). Source apportionment and risk assessment of polycyclic aromatic hydrocarbons in the atmospheric environment of Alexandria, Egypt. Chemosphere, 91(7), 895–903. https://doi.org/10.1016/j.chemosphere.2013.02.018
- Kim, K., Ara, S., Kabir, E., & Brown, R. J. C. (2013). A review of airborne polycyclic aromatic hydrocarbons (PAHs) and their human health effects. Environment International, 60, 71–80. https://doi.org/10.1016/j.envint.2013.07.019
- Kongpran, J., Kliengchuay, W., Niampradit, S., Sahanavin, N., Siriratruengsuk, W., & Tantrakarnapa, K. (2021). The health risks of airborne polycyclic aromatic hydrocarbons (PAHs): Upper North Thailand. Advancing Earth and Space Science, 5(4), 1–10. https://doi.org/10.1029/2020GH000352
- Kumar, A., Sankar, T. K., Sethi, S. S., & Ambade, B. (2019). Characteristics, toxicity, source identification and seasonal variation of atmospheric polycyclic aromatic hydrocarbons over East India. Environmental Science and Pollution Research, 27(1), 678–690. https://doi.org/10.1007/s11356-019-06882-5
- Kumar, S. N., Verma, P., Bastia, B., & Jain, A. K. (2014). Health risk assessment of polycyclic aromatic hydrocarbons: A review. Journal of Pathology and Toxicology, 1(1), 16–30.
- Kural, Balkis, N. C., Aksu, M. A., & Kural, G. (2018). Source identification of Polycyclic Aromatic Hydrocarbons (PAHs) in the urban environment of Istanbul. International Environment and Geoinformatics, 5(1), 53–67. https://doi.org/10.30897/ijegeo.412341
- Lai, Y., Tsai, C., Chen, Y., & Chang-Chien, G. -P. (2017). Distribution and sources of atmospheric polycyclic aromatic hydrocarbons at an industrial region in Kaohsiung, Taiwan. Aerosol and Air Quality Research, 17(3), 776–787. https://doi.org/10.4209/aaqr.2016.11.0482
- Lakhani, A. (2012). Source apportionment of particle-bound polycyclic aromatic hydrocarbons at an industrial location in Agra, India. Scientific World Journal, 2012, 1–10. https://doi.org/10.1100/2012/781291
- Lammel, G. (2015). Polycyclic Aromatic Compounds in the Atmosphere – a Review Identifying Research Needs. Polycyclic Aromatic Compounds, 6638(2–4), 316–327. https://doi.org/10.1080/10406638.2014.931870
- Lawal, A. T., & Fantke, P. (2017). Polycyclic aromatic aromatic hydrocarbons. A review. Cogent Environmental Science, 3(1), 1339841. https://doi.org/10.1080/23311843.2017.1339841
- Lee, Y., Hsieh, Y., Huang, B., Mutuku, J. K., Chang-Chien, G. -P., & Huang, S. (2021). An overview: PAH and nitro-PAH emission from the stationary sources and their transformations in the atmosphere. Aerosol and Air Quality Research, 22(7), 1–20. https://doi.org/10.4209/aaqr.220164
- Lee, B. K., & Vu, T. V. (2010). Sources, distribution and toxicity of polycyclic aromatic hydrocarbons in particulate matter. Air Pollution, 5(1), 100–122.
- Lhotka, R., Pokorna, P., & Zikova, N. (2019). Long-term trends in PAH concentrations and sources at rural background sites in Central Europe. Atmosphere, 10(689), 1–16. https://doi.org/10.3390/atmos10110687
- Li, J., Sai, S., Ho, H., Feng, B., Xu, H., Wang, T., Wu, R., Huang, W., Qu, L., Wang, Q., & Cao, J. (2019). Characterisation of particulate-bound polycyclic aromatic compounds (PACs) and their oxidations in the heavily polluted atmosphere: A case study in urban Beijing, China during haze events. The Science of the Total Environment, 660(660), 1392–1402. https://doi.org/10.1016/j.scitotenv.2019.01.078
- Liu, D., Lin, T., Hussain, J., Zhineng, C., Xu, Y., Li, K., Gan, Z., & Li, J. (2017). Concentration, source identification, and exposure risk assessment of PM2.5-bound parent PAHs and nitro-PAHs in atmosphere from typical Chinese cities. Scientific Reports, 7(1), 1–12. https://doi.org/10.1038/s41598-017-10623-4
- Liu, H., Li, B., Qi, H., Ma, L., Xu, J., Wang, M., Ma, W., & Tian, C. (2021). Source apportionment and toxic potency of polycyclic aromatic hydrocarbons (PAHs) in the air of Harbin, a cold city in Northern China. Atmosphere, 12(3), 1–13. https://doi.org/10.3390/atmos12030297
- Liu, Y., Wang, S., Lohmann, R., Yu, N., Zhang, C., Gao, Y., Zhao, J., & Ma, L. (2015). Source apportionment of gaseous and particulate PAHs from traffic emission using tunnel measurements in Shanghai, China. Atmospheric Environment, 107, 129–136. https://doi.org/10.1016/j.atmosenv.2015.02.041
- Li, Z., Wang, Y., Li, Z., Guo, S., & Hu, Y. (2020). Levels and Sources of PM2.5-associated PAHs during and after the Wheat Harvest in a Central Rural Area of the Beijing-Tianjin-Hebei (BTH) Region. Aerosol and Air Quality Research, 20(5), 1070–1082. https://doi.org/10.4209/aaqr.2020.03.0083
- Li, P., Wang, Y., Li, Y., Li, H., & Yi, X. (2015). Origin and distribution of PAHs in ambient particulate samples at high mountain region in southern China. Advances in Meteorology, 2015, 1–8. https://doi.org/10.1155/2015/245438
- Marinaite, I., Penner, I., Molozhnikova, E., Shikhovtsev, M., & Khodzher, T. (2022). Polycyclic aromatic hydrocarbons in the atmosphere of the southern Baikal region (Russia): Sources and relationship with meteorological conditions. Atmosphere, 13(420), 1–13. https://doi.org/10.3390/atmos13030420
- Masih, J., Singhvi, R., Kumar, K., Jain, V. K., & Taneja, A. (2012). Seasonal variation and sources of polycyclic aromatic hydrocarbons(PAHs) in indoor and outdoor air in a semi-arid tract of Northern India. Aerosol and Air Quality Research, 12(4), 515–525. https://doi.org/10.4209/aaqr.2011.11.0192
- Mesquita, R. S., Drooge, B. L. V., Reche, C., Guimarães, L., Grimalt, J. O., Barata, C., & Piña, B. (2014). Toxic assessment of urban atmospheric particle-bound PAHs: Relevance of composition and particle size in Barcelona (Spain). Environmental Pollution, 184, 555–562. https://doi.org/10.1016/j.envpol.2013.09.034
- Mishra, N., Ayoko, G. A., & Morawska, L. (2016). Atmospheric polycyclic aromatic hydrocarbons in the urban environment: Occurrence, toxicity, and source apportionment. Environmental Pollution, 208, 110–117. https://doi.org/10.1016/j.envpol.2015.08.015
- Mojiri, A., Zhou, J. L., Ohashi, A., Ozaki, N., & Kindaichi, T. (2019). A comprehensive review of polycyclic aromatic hydrocarbons in water sources, their effects, and treatments. The Science of the Total Environment, 696(696), 133971. https://doi.org/10.1016/j.scitotenv.2019.133971
- Moradi, M., Hung, H., Li, J., Park, R., Shin, C., Alexandrou, N., Iqbal, M. A., Takhar, M., Chan, A., & Brook, R. (2022). Assessment of alkylated and unsubstituted polycyclic aromatic hydrocarbons in the air in urban and semi-urban areas in Toronto, Canada. Environmental Science and Technology, 56(5), 2959–2967. https://doi.org/10.1021/acs.est.1c04299
- Nadali, A., Leili, M., Bahrami, A., Karam, M., & Afkhami, A. (2021). Phase distribution and risk assessment of PAHs in ambient air of Hamadan, Iran. Ecotoxicology and Environmental Safety, 209, 111807. https://doi.org/10.1016/j.ecoenv.2020.111807
- Nowakowski, M., Rykowska, P., Wolski, R., & Andrzejewski, R. (2022). Polycyclic aromatic hydrocarbons (PAHs) and their derivatives (O‑PAHs, N‑PAHs, OH‑PAHs): Determination of suspended particulate matter: A review. Environmental Process, 9(2), 1–27. https://doi.org/10.1007/s40710-021-00555-7
- Office of Environmental Health Hazard Assessment. (2015) . Risk assessment guidelines: A guidance manual for preparation of health risk assessments. California EPA Publication.
- Oliveira, M., Slezakova, K., Delerue-Matos, C., Carmo, M., & Morais, S. (2019). Children environmental exposure to particulate matter and polycyclic aromatic hydrocarbons and biomonitoring in school environments: A review on indoor and outdoor exposure levels, major sources and health impacts. Environment International, 124, 180–204. https://doi.org/10.1016/j.envint.2018.12.052
- Patel, A. B., Shaikh, S., Jain, K. R., Desai, C., & Madamwar, D. (2020). Polycyclic aromatic hydrocarbons: Sources, toxicity, and remediation approaches. Frontiers in Microbiology, 11, 11. https://doi.org/10.3389/fmicb.2020.562813
- Pham, C., Boongla, Y., Nghiem, T., Le, H., Tang, N., Toriba, A., & Hayakawa, K. (2019). Emission characteristics of polycyclic aromatic hydrocarbons and nitro-polycyclic aromatic hydrocarbons from Open burning of rice straw in the north of Vietnam. International Journal of Environmental Research and Public Health, 16(2343), 1–17. https://doi.org/10.3390/ijerph16132343
- Polachova, A., Gramblicka, T., Parizek, O., Sram, R. J., Hajslova, J., & Pulkrabova, J. (2019). Estimation of human exposure to polycyclic aromatic hydrocarbons (PAHs) based on the dietary and outdoor atmospheric monitoring in the Czech Republic. Environmental Research, 182(12), 1–39.
- Pomata, D., Filippo, P. D., Riccardi, C., Castellani, F., Simonetti, G., Sonego, E., & Buiarelli, F. (2021). Toxic organic contaminants in airborne particles: Levels, potential sources, and risk assessment. International Journal of Environmental Research and Public Health, 18(8), 1–22. https://doi.org/10.3390/ijerph18084352
- Pongpiachan, S., Hattayanone, M., & Cao, J. (2017). The effect of MWCNT treatment by H2O2 and/or UV on fulvic acids sorption. Atmospheric Environmental Research, 155, 1–12. https://doi.org/10.1016/j.envres.2017.01.037
- Poulain, L., Iinuma, Y., Müller, K., Birmili, W., Weinhold, K., Brüggemann, E., Gnauk, T., Hausmann, A., Löschau, G., Wiedensohler, A., & Herrmann, H. (2011). Diurnal variations of ambient particulate wood burning emissions and their contribution to the concentration of polycyclic aromatic hydrocarbons (PAHs) in Seiffen, Germany. Atmospheric Chemistry and Physics, 11(24), 12697–12713. https://doi.org/10.5194/acp-11-12697-2011
- Pulster, E. L., Johnson, G., Hollander, D., Mccluskey, J., & Harbison, R. (2019). Levels and Sources of Atmospheric Polycyclic Aromatic Hydrocarbons Surrounding an Oil Refinery in Curaçao. Journal of Environmental Protection, 10(03), 431–453. https://doi.org/10.4236/jep.2019.103025
- Qu, L., Yang, L., Zhang, Y., Wang, X., Sun, R., Li, B., Lv, X., Chen, Y., Wang, Q., Tian, C., & Ji, L. (2022). Source Apportionment and Toxic Potency of PM2.5-Bound Polycyclic Aromatic Hydrocarbons (PAHs) at an Island in the. Atmosphere, 13(699), 1–15. https://doi.org/10.3390/atmos13050699
- Ramírez, N., Cuadras, A., Rovira, E., Marcé, R. M., & Borrull, F. (2011). Risk assessment related to atmospheric polycyclic aromatic hydrocarbons in gas and particle phases near industrial sites. Environmental Health Perspectives, 119(8), 1110–1116. https://doi.org/10.1289/ehp.1002855
- Rostami, R., Zarei, A., Saranjam, B., Ghaffari, H. R., Hazrati, S., Poureshg, Y., & Fazlzadeh, M. (2019). Exposure and risk assessment of PAHs in indoor air of waterpipe cafés in Ardebil, Iran. Building and Environment, 155, 47–57. https://doi.org/10.1016/j.buildenv.2019.03.031
- Roy, R., Jan, R., Gunjal, G., Bhor, R., Pai, K., & Satsangi, P. G. (2019). Particulate matter bound PAHs: Toxicity and health risk assessment of exposed inhabitants. Atmospheric Environment, 210, 1–20. https://doi.org/10.1016/j.atmosenv.2019.04.034
- Safo-Adu, G., Ofosu, F. G., Carboo, D., Serfor, Y., & Ofosu, F. G. (2014). Health risk assessment of exposure to particulate polycyclic aromatic hydrocarbons at a tollbooth on a major highway. American Journal of Scientific and Industrial Research, 5(4), 110–119.
- Saha, M., Maharana, D., Kurumisawa, R., Takada, H., Yeo, B. G., & Rodrigues, A. C., et al (2017). Seasonal trends of atmospheric PAHs in Four Asian megacities and source detection using suitable biomarkers. Aerosol and Air Quality Research, 17, 2247–2262.
- Sánchez-Piñero, J., Moreda-Piñeiro, J., Turnes-Carou, I., Fernández-Amado, M., Muniategui-Lorenzo, S., & López-Mahía, P. (2021). Polycyclic aromatic hydrocarbons in atmospheric particulate matter (PM10) at a Southwestern Europe coastal city: Status, sources and health risk assessment. Air Quality, Atmosphere and Health.
- Shen, G., Preston, W., Ebersviller, S. M., Williams, C., Faircloth, J. W., Jetter, J. J., & Hays, M. D. (2018). Polycyclic aromatic hydrocarbons in fine particulate matter are emitted from burning kerosene, liquid petroleum gas, and wood fuels in household cookstoves. United States Environmental Protection Agency, 31(3), 1–14. https://doi.org/10.1021/acs.energyfuels.6b02641
- Shen, G., Tao, X., Yang, Y., Ding, J., Xue, M., Min, Y., Li, H., Shen, W., Wang, W., Wang, B., Wang, R., Wang, S., Russell, A. G., & Russell, A. G. (2012). Emissions of PAHs from Indoor Crop Residue Burning in a Typical Rural Stove: Emission Factors, Size Distributions, and Gas−Particle Partitioning. Environmental Science and Technology, 45(4), 1206–1212. https://doi.org/10.1021/es102151w
- Shimada, K., Nohchi, M., Maeshima, K., Uchino, T., Kobayashi, Y., Ono, K., Ogata, H., Katsumi, N., Inazu, K., & Okochi, H. (2022). Effects of changes in polycyclic aromatic hydrocarbons (PAHs) emissions and degradation on their concentrations in Tokyo from 2007 and 2016. Scientific Reports, 12(1), 1–9. https://doi.org/10.1038/s41598-022-08138-8
- Singh, L., & Agarwal, T. (2020). Quantification of polycyclic aromatic hydrocarbons in kitchen depositions by SUPRA’S-LC-FLR and human health risk assessment. Environmental Research, 187, 109648. https://doi.org/10.1016/j.envres.2020.109648
- Singh, B. P., Zuhaibi, T. A., Alharthy, S. A., Al-Asmari, A., & Rahman, S. (2023). Statistical analysis, source apportionment, and toxicity of particulate and gaseous-phase PAHs in the urban atmosphere. Frontiers in Public Health, 10, 1070663. https://doi.org/10.3389/fpubh.2022.1070663
- Siudek, P., & Frankowski, M. (2018). The role of sources and atmospheric conditions in the seasonal variability of particulate phase PAHs at the urban site in Central Poland. Aerosol and Air Quality Research, 3(18), 1405–1418. https://doi.org/10.4209/aaqr.2018.01.0037
- Teffahi, A., Kerchich, Y., Moussaoui, Y., Romagnoli, P., Balducci, C., Malherbe, C., Kerbachi, R., Eppe, G., & Cecinato, A. (2021). Exposure levels and health risk of PAHs associated with fine and ultrafine aerosols in an urban site in northern Algeria. Air Quality, Atmosphere, & Health, 14(9), 1375–1391. https://doi.org/10.1007/s11869-021-01028-4
- Teixeira, E. C., Dalla, C., Mattiuzi, P., de Oliveira Garcia, K., & Wiegand, F. (2013). Polycyclic aromatic hydrocarbons study in atmospheric fine and coarse particles using diagnostic ratios and receptor model in urban/industrial regions. Environmental Monitoring and Assessment, 185(11), 9587–9602. https://doi.org/10.1007/s10661-013-3276-2
- Tobiszewski, M., & Namie, J. (2012). PAH diagnostic ratios for the identification of pollution emission sources PAH diagnostic ratios for the identification of pollution emission sources. Environmental Pollution, 162, 110–119. https://doi.org/10.1016/j.envpol.2011.10.025
- Tsiodra, I., Grivas, G., Tavernaraki, K., Bougiatioti, A., Apostolaki, M., Paraskevopoulou, D., Gogou, A., Oikonomou, K., Tsagkaraki, M., Zarmpas, P., Nenes, A., & Mihalopoulos, N. (2021). Annual exposure to PAHs in urban environments linked to wintertime wood-burning episodes. Atmospheric Chemistry and Physics, 21(23), 1–24.
- United States Environmental Protection Agency. (2019) . Guidelines for human exposure assessment. US EPA Publication.
- Wang, X., Qing, W., Li, B., Ming, Y., Qian, L., Min, L., Jian, X., Ma, M., Li, Y., & Peng, Y. (2010). Characteristics and sources of atmospheric polycyclic aromatic hydrocarbons (PAHs) in Shanghai, China. Environmental Monitoring and Assessment, 165(1–4), 295–305. https://doi.org/10.1007/s10661-009-0946-1
- Wang, W., Wang, Q., Nakajima, D., Lu, S., Xiao, K., Chowdhury, T., Suzuki, M., & Liu, F. (2021). Industrial source contributions and health risk assessment of fine particle-bound polycyclic aromatic hydrocarbons (PAHs) during spring and late summer in the Baoshan area, Shanghai. Processes, 9(11), 1–15. https://doi.org/10.3390/pr9112016
- Wang, Y., Zhang, H., Zhang, X., Bai, P., Neroda, A., Mishukov, V. F., Zhang, L., Hayakawa, K., Nagao, S., & Tang, N. (2022). PM-bound polycyclic aromatic hydrocarbons and nitro-polycyclic aromatic hydrocarbons in the ambient air of Vladivostok: Seasonal variation, sources, health risk assessment, and long-term variability. International Journal of Environmental Research and Public Health, 19(5), 2878. https://doi.org/10.3390/ijerph19052878
- World Health Organisation. (2000). Air quality guidelines for Europe (2nd ed.). WHO Publishers.
- World Health Organisation. (2014) . World Health Statistics. WHO Publisher.
- World Health Organisation. (2021). Human health effects of polycyclic aromatic hydrocarbons as ambient air pollutants: Report of the working group on polycyclic aromatic hydrocarbons of the joint task force on the health aspects of air Pollution. WHO Publishers.
- Wu, M., Lin, P., Pan, C., & Peng, C. (2019). Risk assessment of personal exposure to polycyclic aromatic hydrocarbons and aldehydes in three commercial cooking workplaces. Scientific Reports, 9(1661), 1–11. https://doi.org/10.1038/s41598-018-38082-5
- Yang, L., Suzuki, G., Zhang, L., Zhou, Q., Zhang, X., Xing, W., Shima, M., Yoda, Y., Nakatsubo, R., Hiraki, T., Sun, B., Fu, W., Qi, H., Hayakawa, K., Toriba, A., & Tang, N. (2019). The characteristics of polycyclic aromatic hydrocarbons in different emission source areas in Shenyang, China. International Journal of Environmental Research and Public Health, 16(16), 1–11. https://doi.org/10.3390/ijerph16162817
- Yang, L., Zhang, H., Zhang, X., Xing, W., Wang, Y., Bai, P., Zhang, L., Hayakawa, K., Toriba, A., & Tang, N. (2021). Exposure to atmospheric particulate matter-bound polycyclic aromatic hydrocarbons and their health effects: A review. International Journal of Environmental Research and Public Health, 18(4), 2177. https://doi.org/10.3390/ijerph18042177
- Zhang, N., Geng, C., Xu, J., Zhang, L., Li, P., Han, J., Gao, S., Wang, X., Yang, W., Bai, Z., Zhang, W., & Han, B. (2022). Characteristics, source contributions, and Source-specific health risks of PM2.5-bound polycyclic aromatic hydrocarbons for senior citizens during the heating season in Tianjin, China. International Journal of Environmental Research and Public Health, 19(8), 5–9. https://doi.org/10.3390/ijerph19084440
- Zhang, L., Yang, L., Zhou, Q., Zhang, X., Xing, W., Zhang, H., Tang, K., Hayakawa, N., & Tang, N. (2020). Impact of the COVID-19 outbreak on the long-range transport of particulate PAHs in East Asia. Aerosol and Air Quality Research, 20(10), 2035–2046. https://doi.org/10.4209/aaqr.2020.07.0388
- Zhang, Y., Zheng, H., Zhang, L., Zhang, Z., Xing, X., & Qi, S. (2019). Fine particle-bound polycyclic aromatic hydrocarbons (PAHs) at an urban site of Wuhan, central China: Characteristics, potential sources, and cancer risks apportionment. Environmental Pollution, 246, 319–327. https://doi.org/10.1016/j.envpol.2018.11.111