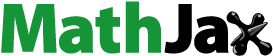
Abstract
Hot-dip galvanization is a common corrosion-prevention technique for steel structures. The steel is submerged in a molten zinc bath, which forms a zinc coating on the steel’s surface. This provides a barrier against the corrosive elements. However, the galvanized covering can still deteriorate, especially in harsh environments. As a consequence, several studies have been conducted to improve the corrosion resistance of galvanized steel by adding metallic elements to the zinc coating. The aim of this study is to look at how metallic additions (Al addition up to 1%, Bi addition up to 1%, Pb addition up to 1%, Sb addition up to (1% and 2%), Sn addition up to 5%, Zn-Bi-Pb 1% addition, Zn-Sb-Sn 1% addition) affect the zinc coating by hot dipping galvanization. The microhardness, thickness, adherence, microstructure test, coating adhesion (Pull-Off test), and corrosion rate are studied.
1. Introduction
Hot-dip galvanizing protects the steel from corrosion. Adding metallic components like aluminum, magnesium, and zinc to hot-dip galvanized coatings improves their effectiveness. By adding metal elements, galvanized coatings become corrosion-resistant and mechanically stronger, making them suitable for many applicatios. In hot-dip galvanization, metallic additions play an important role.
Several galvanized coatings were studied using EIS by Barranco et al. (Citation2004). For pure zinc and Zn-5%Al coatings, electrochemical corrosion values were overestimated compared to gravimetric values, but not for Zn-10%Fe. As a result of corrosion products that layer barriers, immersion time was linearly related to attack time (Barranco et al., Citation2004).
Cabral et al. (Citation2006) examined the anticorrosion performance of a novel pretreatment AA2024-T3 and hot-dip galvanized steel. EIS was used to assess corrosion resistance in NaCl solutions. Results demonstrated the pre-treatment provides excellent corrosion protection and improved corrosion protection due to improved barrier properties and cerium-based inhibitors in the silane matrix (Cabral et al., Citation2006).
The texture and corrosion resistance of hot-dip galvanized coatings were studied by Asgari et al. (Citation2009) (00.2) texture coatings had lower corrosion current densities, and thicker coatings had lower (00.2) texture intensity. A variety of morphologies were observed on dull and bright spangles in the saline spray test (Asgari et al., Citation2009).
Using hot-dip galvanized steel, Manchet et al. (Citation2010) assessed its electrochemical and physical properties. The research examined the homogeneity, defects, electrochemical behavior, and composition of the conversion layer, which contains organic phases and manganese phosphate. As a result, Cr-free solutions reduced corrosion current density and restricted oxygen passage to hot-dip galvanized substrates. Neither the organic compound nor the manganese phosphate altered the surface morphology or functionality of the conversion layer (Le Manchet et al., Citation2010).
Azimi (Citation2012) examined pimple defects in hot-dip galvanized steel sheets produced in continuous processes, their microstructure, and their impact on corrosion and mechanical behavior. The research found that metal chips and embedded particles on the sheet surface were the primary causes of pimples. This reduced rust resistance and ductility but had no significant effect on strength (Azimi et al., Citation2012).
Akamphon, et al. (Citation2012) tested novel galvanizing techniques for long-term hot-dip galvanized coatings. When the pre-coating layer is thin, electrodeposition or electroless deposition in a new process reduces zinc consumption, improves corrosion protection, and is cost-effective. By reducing zinc consumption and eliminating fluxing, cost savings can be achieved. In conclusion, the study offers useful insight into implementing environmentally friendly galvanizing techniques (Akamphon et al., Citation2012).
Okafor et al. (Citation2013) investigated the microstructure and fracture behaviour of Grade 65 steels used in wind turbines. The fracture toughness of two different carbon level chemistries was investigated using the 3-point bend test. Results showed lower carbon steel had greater fracture resistance after zinc galvanization. SEM analysis revealed that zinc and zinc bath additives at the fracture ends caused the loss of ductility (Okafor et al., Citation2013).
Okafor et al. (Citation2013) examine the microstructure and fracture behavior of ASTM 572 Grade 65 steels. The fracture toughness of zinc-coated and uncoated samples was investigated using two carbon-level chemistries. Higher fracture resistance was seen after zinc galvanizing. According to SEM analysis, zinc, and zinc bath additives reduce ductility (Okafor et al., Citation2013).
Yadav (Citation2021) studied hot-dip galvanization. The researchers examined temperature, immersion time, extraction speed, and sample thickness as factors affecting galvanization quality. 3–5 m/min provided the highest quality in gauges 18, 22, and 28. Temperature and gauge also affect galvanization quality (Yadav, Citation2021).
Ding et al. (Citation2022) studied zinc slag flaws that lower hot-dip galvanized steel sheets’ surface quality. SEM was used to examine the large, round zinc slag defects. A variety of factors can lessen the quantity and amount of zinc slag defects, including adjusting air knife pressure, zinc tank aluminum content, and RCS fan opening. These modifications can improve the steel plate’s surface quality and make it suitable for automotive use, according to the study (Ding et al., Citation2022).
It is reported by Chakraborty et al. (Citation2022) that metallic pre-coatings like iron, nickel, copper, and/or their alloys like Ni-P, and Cu-Sn are capable of creating defect-free galvanized coatings. With a focus on microstructural development and interface formation, this review provides a thorough, comparative, and critical overview of the literature in this area. The review also discusses how such pre-coatings affect liquid metal embrittlement and corrosion performance, data from filed patents, industrial practices, recent industrial adaptations, and future research directions (Chakraborty et al., Citation2022).
According to Kancharla et al (Citation2022), copper (Cu) pre-coating improves the quality of hot-dip galvanized (GI) coatings on high-strength C-Mn steel strips. A high-quality, defect-free coating was achieved with the GI coating with Cu pre-coating because it had better surface quality and a higher texture coefficient (TC) of the crystal plane of Zn. Cu-pre-coated GI steel also created a continuous iron aluminide (Fe-Al) intermetallic interfacial layer, resulting in greater corrosion resistance (Kancharla et al., Citation2022).
Huang, et al. (Citation2022) studied the surface morphology, make-up, and rate at which plate thickness changes during stamping on DP590 alloyed hot-dip galvanized dual-phase steel. It appears that upgrading dies and optimizing plate design can resolve to stamp dezincification flaws (Huang et al., Citation2022).
Xiong, et al. (Citation2022) studied coarse stripe flaws in alloyed hot-dip galvanized steel slabs. A defect area had concave-convex features, insufficient alloying, and perhaps oxide. By using cleaning zones and adjusting the furnace atmosphere, defect occurrence was reduced by 76%. Surface flaws in alloyed hot-dip galvanized steel plates can be minimized by the findings (Xiong et al., Citation2022).
Verma (Citation2022) examined how nickel content and dipping duration affect coating thickness. 0.05% nickel and 3 minutes of dipping time achieved the minimal coating thickness. For steels with 0.18% and 0.28% silicon, respectively, the thickness reduction was 38.96% and 29.57%. Results of the study can help industries gain a competitive edge and enhance the quality of their products (Verma et al., Citation2022).
The purpose of this study is to investigate the effect of elemental additions on zinc coatings applied by hot-dip galvanization to steel alloys. A study examines the characteristics of microhardness, thickness, and adhesion, as well as the microstructure, coating adhesion (Pull-Off Test), and corrosion rate of the coating.
2. Experimental work
2.2. Hot-dip galvanization procedure
Hot-dip galvanization begins with thoroughly cleaning the steel surface to remove any oil, grease, or other contaminants that may interfere with the zinc’s bonding. At approximately 450°C, the steel is immersed in liquid zinc. In steel, zinc reacts with iron to produce intermetallic layers that prevent corrosion. Galvanizing steel is an effective and cost-effective method of protecting it from corrosion because it gives it a long-lasting, low-maintenance coating. The excellent corrosion resistance and the high strength-to-weight ratio of galvanized steel make it ideal for construction, automotive, and manufacturing applications.
Hot-dip galvanization, however, is not appropriate for all applications. The thickness of the coating depends on the shape and size of the object being galvanized, and it can cause distortion or warping. Extreme temperatures or harsh chemicals may make galvanized steel unsuitable (Bondareva & Melnikov, Citation2016).
Galvanizing the material was accomplished using a common type of sheet metal that is commonly used in construction. The material was a standard steel sheet with dimensions of 100 × 50 cm and a 2 mm thickness. To facilitate the galvanizing process and to separate samples, the plate was cut into smaller pieces, sheet components of steel alloy shown in Tables shown zinc alloy components.
Table 1. Chemical composition analysis of AISI 1010 sheet
Table 2. Zinc alloy components before any addition used in the galvanizing process
In order to dip the sample in zinc, it is first divided into 4 × 4 cm pieces and then cleaned with a metal brush to remove the oxides and rust from the sample’s surface. After polishing the sample’s surface with various grades of sandpaper, residual oxidation is removed with acid. It is then immersed in a flux solution prepared by 30% flux powder and 70% pure water for 4 min. Depending on the manufacturer’s recommendations of flux powder, the solution temperature must be between 40 and 60 ºC to function properly. Afterward, it is hot dipped at 100°C until a saline layer forms on its surface. The zinc ammonium chloride flux with chemical formula Cl4H8N2Zn is used to remove the oxide film that forms after acid cleaning and to deposit a protective layer to prevent further oxides from forming on the steel surface before immersion in molten zinc. Fluxing is the final step before immersion in molten zinc after cleaning samples or products in general. The melting temperature of zinc is 419.5 ºC. To make molten zinc sufficiently fluid for immersion in water, factories use zinc kettles to melt it and maintain its temperature between 435 and 455 ºC. A zinc kettle at the Basrah galvanizing factory uses natural gas to heat it. Since these possibilities were not available, an electric furnace and a crucible were used to melt the zinc, as well as for the immersion process of the samples. As shown in Table , the galvanizing process has been repeated several times with different additive percentages.
Table 3. The elements added to zinc with their percentages
2.2. Micro-hardness Test
Vickers hardness test method has been used with accordance of ASTM E92 (, Citation2017) for evaluation the effect of adding metallic elements on surface properties. The hardness of coated samples was measured by pressing an indenter into its surface under a load of 100 g and for a dwell time of 30 s. Different locations on the surface of the samples were chosen to measure the hardness value and the average of those values has been reported as a final value.
2.3. Thickness Test
The thickness test shows the amount of protection from ambient conditions since the coating layer thickness represents the outer covering of the sample surface. Galvanized coatings provide greater corrosion protection the thicker they are, so the thickness test will let us know how much corrosion protection they provide. The measuring procedure was done in accordance with ASTM B499 (, Citation2021).
2.4. Coating Adhesion Test (Pull-Off Test)
An accurate pull-off adhesion test measures coating quality by measuring the force required to pull an area of the coating from the substrate using a 20 mm diameter dolly. The adhesive preparation process takes 2 hours, and excess adhesive is removed before fixing the dolly to the galvanized coating for at least 24 h. The procedure was accomplished according to ASTM D4541 (ASTM, Citation2022).
2.5. Surface Morphology Examination
The OLYMPUS type (Model G×41) Optical microscopy was used to examine the surface of the galvanized samples and evaluate the effect of alloying additives on the surface morphology.
2.6. Corrosion Rate Test
The corrosion process weakens metal and causes it to fail. The ASTM G5 (, Citation2105) standard performs a corrosion test with a standard scanning rate of 0.1667 mV/s to determine the corrosion rate of a coated specimen using a Potentiostat. The corrosion potential (ECorr) between the working electrode and the reference electrode is measured using an electrolyte solution of NaCl (3.5%).
3. Results and discusion
3.1. Results of micro hardness test
A Vickers microhardness test was carried out according to ASTM E92 standard, Table . Presents the results.
Table 4. Relationship between alloying additions and microhardness
It is possible to determine the amount of difference and the effect the additions have on zinc plating. The additives (Zn-Pb 1% and Zn-Sb-Sn 1%) reduced microhardness, and Zn-Sb 1% and Zn-Bi-Pb 1% gave approximately the same level of microhardness as pure zinc. As for the rest of the additives, they provide higher microhardness values than pure zinc, which is considered an advantage. Figure illustrates these differences and values.
3.2. Results of thickness test
In order to measure the thickness of the test sample, a magnetic thickness gauge model (Posi Tector 6000 FNS Probe) was used. It complies with ASTM B 499. Using this test, the device must be calibrated by measuring the thickness of an iron piece before use. This is compared to measurements of a device (shims) containing a thickness gauge for each piece of measurement of a device specified in a specific color starting at 24 microns and ending at 519 microns. With just one click on the surface, samples can be taken, and readings can be taken for the sample, and then the galvanized coating thickness can be recorded, the results shown in Table .
Table 5. Relationship between alloying additions and thickness
In addition, the addition of lead is the only factor that has increased the thickness of the paint. Additionally, Sb 2% gives us almost the same thickness, while the rest of the additives give us lower thickness values. As can be seen in Figure , the values and differences are presented.
3.3. Results of coating adhesion test (Pull-Off Test)
A manual hydraulic pump designed to apply continuous smooth pressure in one stroke (model AT_M20) was used to perform the test in accordance with ASTM D4541 as illustrated in Figure .
The pull-off test indicates that the addition of Zn-Al (1%), and Zn-Bi-Pb 1% has increased the pull-off force, which indicates an increase in the coating’s adhesion strength. And the rest of the additions reduced the pull-off force. Figure shows the values and differences.
3.4. Results of Microstructure test
The test is conducted using optical microscopes the results of the microstructure test were obtained under a microscope with a magnification force of 200× as shown in Figure .
3.5. Results of Adherence test
Although the adherence test can be performed using a knife with several sharp blades to scratch the surface vertically once and horizontally again. Until 25 squares are formed on the surface and then stick an adhesive and remove it, if the galvanized coating did not remove, it is sound. All samples achieved 100% adhesion. This test is applied according to standard ASTM-D3359-09 (Jiang et al., Citation2021).
3.6. Results of corrosion test
Corrosion is undesirable phenomena that weaken the metal and cause failure corrosion test is performed according to standard ASTM G44 (Citation2105), to get the corrosion rate of coated specimen using a potentiostat which is an electronic instrument (Citation2105), the results shown in Table .
Table 6. Relationship between alloying additions and corrosion rate
A Tafel plot is a graphical (typically logarithmic) representation of the relationship between a metal’s electrode potential and the current generated in an electrochemical cell. Each sample has two curves: the top depicts the sample’s anodic behavior, and the bottom indicates the solution’s cathodic behavior , for the corrosion rate, the additions of (Zn-Sb 1% and Zn-Sb 2%) Reduced corrosion rate (increasing the corrosion resistance). This means a longer endurance period before collapsing and the metal get corrosion, increasing the corrosion resistance is considered an advantage. The values and differences are shown in Figures .
As shown in the previous table, the values of pure zinc are the reference that the rest of the additives compared with. Overall, pure zinc is the best coating approved by the galvanizing manufacturer because it achieves the best average values that can be obtained (Almeraya-Calderón et al., Citation2022).
Neglecting (Zn-Sb-Sn 1%) from the comparison, because all tests indicated negatively on it. The rest of the additions can be used in certain placesfor example, Addition of antimony at a percentage of 1&2% for applications that require a low corrosion rate. Because the antimony achieved a low rate of corrosion with no significant effect on the rest of the properties, it is true that it was reduced, but not excessively. It is kind of successful addition (Rossi et al., Citation2022).
The addition of aluminum in a very small percentage of 1% can also be used to improve the adhesion strength as well as the microhardness, and it can be used in applications where thickness and corrosion rate are not important, because according to the tests, these values have been reduced. It is kind of successful addition (Al-Negheimish et al., Citation2021).
As for the rest of the additions, they only enhance one single property. It does not consider useful to adding them and it may be economically costly to enhance just one property.
As for the grain size, the larger the size, the lower the microscopic hardness. The pictures taken by the microscopic, despite the fact that the size of the magnification force is equal for all samples (X200). However, not all samples gave clear pictures of the size of the particles. It is due to two reasons. The first is not to use a etch solution, because the etch solution are acidic in nature. And zinc has a grudge with acids because it causes it to dissolve. The second reason may be that the grain size is very small and the mentioned magnification force could not detect them. Or because of the heterogeneous nature of the surface. For example, in adding aluminum (Zn-Al 1%), we notice that the size of the particles has increased indeed. But it was accompanied by an increase in microscopic hardness, this is due to the formation of side compounds and their deposition on the grain boundaries. This can be observed from the difference in the thickness of the grain boundary lines. As for the addition of lead (Zn-Pb 1%), the increase in the size of the particles is accompanied by a decrease in the microhardness, and this is fully consistent with the mentioned theory. And so on for the rest of the additions.
4. Conclusions
1 – Al addition up to 1% improves Mechanical properties of galvanization coating by increasing microhardness, decrease coating thickness, increase adhesion and pull-off force, but slightly increase corrosion rate.
2 – Bi addition up to 1% improves Mechanical properties of galvanization coating by increasing microhardness, decrease coating thickness, but slightly increase corrosion rate and decrease adhesion and pull-off force.
3 – Pb addition up to 1% reduces Mechanical properties by increasing thickness and corrosion rate.
4 – Sb addition up to (1% and 2%) generally improves mechanical properties of galvanization coating by increasing microhardness, and decreasing corrosion rate, but slightly decrease. adhesion and pull-off forces.
5 – Sn addition up to 5% improves Mechanical proprieties by significant increase in microhardness and increase corrosion rate.
6 – Zn-Bi-Pb 1% addition slightly increase corrosion rate without effect on microhardness.
7 – Zn-Sb-Sn 1% addition reduces all Mechanical properties without enhancing any, except reducing thickness which is not enough.
Disclosure statement
No potential conflict of interest was reported by the authors.
References
- Akamphon, S., Sukkasi, S., & Boonyongmaneerat, Y. (2012). Reduction of zinc consumption with enhanced corrosion protection in hot-dip galvanized coatings: A process-based cost analysis. Resources Conservation & Recycling, 58, 1–12. https://doi.org/10.1016/j.resconrec.2011.10.001
- Almeraya-Calderón, F., Samaniego-Gámez, O., Maldonado-Bandala, E., Nieves-Mendoza, D., Olguín-Coca, J., Jáquez-Muñoz, J. M., Cabral-Miramontes, J., Flores De Los Rios, J., Bautista-Margulis, R., & Gaona-Tiburcio, C. (2022). Corrosion behavior of passivated martensitic and semi-austenitic precipitation hardening stainless steel. Metals, 12(6), 1033.. https://doi.org/10.3390/met12061033
- Al-Negheimish, A., Hussain, R. R., Alhozaimy, A., & Singh, D. D. N. (2021). Corrosion performance of hot-dip galvanized zinc-aluminum coated steel rebars in comparison to the conventional pure zinc coated rebars in concrete environment. Construction and Building Materials, 274, 121921.. https://doi.org/10.1016/j.conbuildmat.2020.121921
- Asgari, H., Asgari, A., & Golozar, M. A. (2009). Effect of coating thickness on modifying the texture and corrosion performance of hot-dip galvanized coatings. Current Applied Physics, 9(1), 59–66. https://doi.org/10.1016/j.cap.2007.10.090
- ASTM B499. (2021) . Standard test method for measurement of coating thicknesses by the magnetic method: nonmagnetic coatings on magnetic basis metals. ASTM International.
- ASTM D4541. (2022) . Standard test method for pull-off strength of coatings using portable adhesion testers. ASTM International.
- ASTM E92. (2017) . Standard test methods for vickers hardness and knoop hardness of metallic materials. ASTM International.
- ASTM G5. (2105) . Standard reference test method for making potentiodynamic anodic polarization measurements. ASTM International.
- Azimi, A., Ashrafizadeh, F., Toroghinejad, M. R., & Shahriari, F. (2012). Metallurgical analysis of pimples and their influence on the properties of hot dip galvanized steel sheet. Engineering Failure Analysis, 26, 81–88. https://doi.org/10.1016/j.engfailanal.2012.05.026
- Barranco, V., Feliu, S., Jr., & Feliu, S. (2004). EIS study of the corrosion behaviour of zinc-based coatings on steel in quiescent 3% NaCl solution. Part 1: Directly exposed coatings. Corrosion Science, 46(9), 2203–2220. https://doi.org/10.1016/j.corsci.2003.09.032
- Bondareva, O. S., & Melnikov, A. A. (2016, November). Effect of the silicon content in steel on the hot-dip zinc coating microstructure formation. Proceedings of the IOP Conference Series: Materials Science and Engineering, Tomsk, Russian Federation (Vol. 156, No. 1, pp. 012015). IOP Publishing.
- Cabral, A. M., Trabelsi, W., Serra, R., Montemor, M. F., Zheludkevich, M. L., & Ferreira, M. G. S. (2006). The corrosion resistance of hot dip galvanised steel and AA2024-T3 pre-treated with bis-[triethoxysilylpropyl] tetrasulfide solutions doped with Ce (NO3) 3. Corrosion Science, 48(11), 3740–3758. https://doi.org/10.1016/j.corsci.2006.01.010
- Chakraborty, A., Ghosh, R., Sudan, M., & Mondal, A. (2022). Improvement in hot dip galvanized coating microstructure and properties by pre-metallic deposition on steel surface: A comprehensive review. Surface & Coatings Technology, 449, 128972. https://doi.org/10.1016/j.surfcoat.2022.128972
- Ding, X., Feng, H., & Zhao, C. (2022, December). Study on zinc slag defects on surface of pure hot dip galvanized steel sheet. Proceedings of the Journal of Physics: Conference Series, Guangzhou, China (Vol. 2390, No. 1, pp. 012022). IOP Publishing.
- Huang, G., Liu, X., & Li, L. (2022, December). Analysis of stamping dezincification of DP590 alloyed hot dip galvanized dual phase steel. Proceedings of the Journal of Physics: Conference Series, Guangzhou, China (Vol. 2390, No. 1, pp. 012015). IOP Publishing.
- Jiang, H., Liao, Y., Jing, L., Gao, S., Li, G., & Cui, J. (2021). Mechanical properties and corrosion behavior of galvanized steel/Al dissimilar joints. Archives of Civil and Mechanical Engineering, 21(4), 1–13.. https://doi.org/10.1007/s43452-021-00320-5
- Kancharla, H., Mandal, G. K., Singh, S. S., & Mondal, K. (2022). Effect of prior copper-coating on the microstructural development and corrosion behavior of hot-dip galvanized Mn containing high strength steel sheet. Surface & Coatings Technology, 437, 128347. https://doi.org/10.1016/j.surfcoat.2022.128347
- Le Manchet, S., Landoulsi, J., Richard, C., & Verchère, D. (2010). Study of a chromium-free treatment on Hot-Dip Galvanized steel: Electrochemical behaviour and performance in a saline medium. Surface & Coatings Technology, 205(2), 475–482. https://doi.org/10.1016/j.surfcoat.2010.07.009
- Okafor, I. C., O’Malley, R. J., Prayakarao, K. R., & Aglan, H. A. (2013). Effect of zinc galvanization on the microstructure and fracture behavior of low and medium carbon structural steels. Engineering, 05(08), 656–666. https://doi.org/10.4236/eng.2013.58079
- Rossi, S., Pinamonti, M., & Calovi, M. (2022). Influence of soil chemical characteristics on corrosion behaviour of galvanized steel. Case Studies in Construction Materials, 17, e01257.. https://doi.org/10.1016/j.cscm.2022.e01257
- Verma, N., Sharma, V., Badar, M. A., Choubey, N., & Parihar, R. S. (2022). Optimization of Zinc Coating Thickness by Unreplicated Factorial Design of Experiments in Hot-Dip Galvanization Process. International Journal of Precision Engineering and Manufacturing, 23(10), 1173–1182. https://doi.org/10.1007/s12541-022-00695-2
- Xiong, T., Ren, J., Luo, C., & Zuo, R. (2022, December). Analysis of coarse stripes of alloyed hot-dip galvanized steel sheets. Proceedings of the Journal of Physics: Conference Series, Guangzhou, China (Vol. 2390, No. 1, pp. 012017). IOP Publishing.
- Yadav, S. (2021). Performance observation of hot dip galvanization for steel sheets. Proceedings of the Materials Today, Delhi Technical Campus, Greater Noida (Vol. 46, pp. 6700–6703).