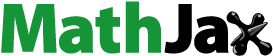
Abstract
Laminated bamboo presents potential physical and mechanical properties for being used as a construction material. However, this material presents low capacity if being used as a beam with a bending moment load due to its low flexural rigidity. The low flexural rigidity enlarges the beam deflection and lowers the service load, leading to the non-optimum usage of laminated bamboo with excellent strength. To increase the flexural rigidity in this study, laminated bamboo beams were given additional Carbon Fiber Reinforcement Polymer (CFRP) on the tension section, compression section, as well as the tension and compression section. The CFRP was also applied with various lengths of 0.2, 0.5, and 0.7 L, where L represents the beam span length. The results of the four-point bending test showed that the addition of CFRP to laminated bamboo beams significantly increases the flexural rigidity. The flexural rigidity level is directly proportional to the length of the CFRP used, according to the equation y = 0.608 x + 1.192, where y (multiplied by 1010) is the flexural rigidity of the beam and x is the ratio of the CFRP length toward the length of the beam span. The increase of flexural rigidity for the CFRP with length variations of 0.2, 0.5, and 0.7 L are 15.36, 24.90, and 38.86% calculated toward the control beam. Further, the placement of CFRP also increases flexural rigidity. The lowest increase in flexural rigidity occurred in beams given CFRP in compression was 24.25%, followed by beams treated with CFRP in tension of 28.79%, and the highest flexural rigidity was observed in beams that were given CFRP in tension and compression, of 38.86%, each calculated in comparison to the control beam.
Reviewing Editor:
Introduction
Bamboo is a construction material that offers great potential to be enhanced as a sustainable construction material. Therefore, the investigation on laminated bamboo becomes a thought-provoking research topic. Studies conducted by Dauletbek et al. (Citation2022), Kamarudin et al. (Citation2016), and Sulastiningsih et al. (Citation2021) reported the physical and mechanical properties of laminated bamboo as a building material. Those studies accentuate the suitability of laminated bamboo as a construction material. On the other hand, laminated bamboo also carries a weakness, such as having a low flexural modulus of elasticity compared to other materials, such as steel. Li et al. (Citation2015) experimented on beams made of laminated Moso Bamboo slats with a cross-sectional height of 100 mm, a span length of 2100 mm, and variations in cross-sectional width (45, 50, 60, 70, and 80 mm). That study uncovered the average flexural elastic modulus from laminated bamboo of 10.912 MPa. Meanwhile, Rusch et al. (Citation2019) investigated panels from laminated Dendrocalamus asper bamboo and reported a flexural elastic modulus of 11.215 MPa. Gao et al. (Citation2022) examined beams made of laminated bamboo with a size of 60 × 120 × 2040 mm and obtained a flexural elastic modulus of 9.630 MPa. The low flexural modulus of elasticity reduces the flexure rigidity of the beam, leading to enlarged deflection and a smaller service load. Karyadi and Susanto (Citation2017) explored laminated bamboo beams with a flexural elastic modulus of 14.504 MPa and reported a service load of 8.64% from the maximum load withstood by the beam. Therefore, a higher flexure rigidity of laminated bamboo beams will enhance its service load, enabling the maximum usage of bamboo’s high flexural strength.
The research investigating the procedures for improving the flexure rigidity of laminated bamboo beams has not been extensively carried out, but similar studies have been carried out on timber.
Raftery and Whelan (Citation2014) amplify Low-Grade glued laminated timber beams using Graphite Fiber-Reinforced Polymer (GFRP) rods installed in the tensile and compressive sections of the beam, reporting that the use of 1.4% GFRP rods installed in the tensile section increases the flexural rigidity of the beam by 11.2%. Meanwhile, reinforcement with GFRP rods with a volume of 1.4% on each installed in the tensile and compressive sections increases the flexural rigidity by 22%.
Andor et al. (Citation2015) examined a beam from Norway Spruce wood 95 × 95 × 1800 mm in size using Carbon Fiber Reinforced Polymer (CFRP) by strengthening at the tensile part. That study discovered a 16% flexure rigidity increase in the beam given no treatment. Meanwhile, Corradi et al. (Citation2018) investigated hardwood beams being strengthened using GFRP at the tensile part, with 145 × 145 × 2310 mm beam dimension and 8 × 80 × 2000 mm GFRP. That study reported higher flexural rigidity from 19.7% GFRP than the control.
Liu et al. (Citation2020) conducted a study on the use of BFRP strips with variations of lengths to improve the flexure behavior of poplar timber beams. Further, the study concluded that the addition of Basalt Fiber Reinforced Polymer (BFRP) with a length equal to the beam length would provide the most significant increase in the flexural rigidity of the beam. However, this study did not report the magnitude of the increase in beam flexural rigidity.
Corradi et al. (Citation2021) reviewed the addition of CFRP to enhance the flexural rigidity of defects in local wooden beams. That study concluded that the addition of CFRP increases the beam’s flexure rigidity by 22.7%, as well as lowering the defects, preventing the local fracture, and reducing the deformation.
Al‑Katib et al. (Citation2022) reinforced timber beams with CFRP at varying lengths and widths, and then placed them on the tensile part of the beam. The results indicate that the variation in CFRP length presents a significant effect on increasing the flexural rigidity of the beam, but the variation in CFRP width has no significant effect.
Meanwhile, Wdowiak-Postulak et al. (Citation2023) examined the use of various reinforcement elements in improving the flexural performance of Scots Pine beams. The reinforcement elements include steel plates, steel or Fiber Reinforced Polymer (FRP) bars, CFRP, Aramid Fiber Reinforced Polymer (AFRP), and GFRP. The results showed that the highest flexural rigidity occurred for beams with CFRP mats and the lowest for beams with AFRP, with an increase in flexural rigidity of around 30% with respect to the unreinforced beams.
Improvement of flexural rigidity using FRP on laminated bamboo beams has been carried out previously by Wei et al. (Citation2015), who enhanced flexural reinforcement of laminated bamboo beams by placing steel bars in the tensile section. The steel bars were 12 and 16 mm in diameter, with a configuration of one steel bar and two steel bars. The results implied that an increase in flexural rigidity had been indicated by an increase in the ratio between service load and service deflection by 20.2–36.9% against the control beam.
Wei et al. (Citation2017) placed FRP on the tensile area, with a variation of FRP thickness. The results signified 16.4–40.3% higher flexural rigidity on the laminated bamboo beams treated with FRP.
Zhao et al. (Citation2021) investigated the flexural strengthening of laminated bamboo beams using steel bars placed in the tensile section using various ratios between the cross-sectional area of steel bars and the beam area. The results indicate an increase in the flexure rigidity of the beam following the increase in the ratio between the cross-sectional area of the steel bar and the cross-sectional area of the beam, ranging from 3.81 to 36.80% against the control beam.
Those previous studies focused on the laminated bamboo position as the strengthening agent on the tensile zone of the beams. Meanwhile, the contributing parts for the beam flexural rigidity are not only the tensile parts but also the compression parts. Therefore, in this study, we placed the material on both the tensile and compression parts. Besides, in previous studies, the treatment was completed at the same length as the beam. Their results are not optimum since the beam’s bending moment is not spread evenly. Thus, in this study, we also examined the different lengths of treatment, prioritizing the part of the beam offering the highest bending moment. Different from the available previous studies, in this study, we applied the CFRP on the tensile and compression with various lengths. This study aims to investigate the flexural rigidity of the beams added with CFRP on the tensile part, compression part, as well as tensile and compression part with various lengths.
The flexure rigidity on the elastic border can be computed using Formula (1) (Gere, Citation2004) with a four-point bending method, as illustrated in .
(1)
(1)
Description:
EI: flexural rigidity from a multiplication between E and I (Nmm2)
E: elastic modulus (N/mm2)
I: moment of inertia of the beam cross-section (mm4)
F: change of load on the elastic zone (N)
change of deflection in the middle of the beam on the elastic zone (mm)
L: beam span (mm)
a: distance between the nearest support and load point (mm)
Materials and methods
The 3–5-year-old and ∼150 mm diameter of Dendrocalamus asper bamboo from Malang Regency, East Java, Indonesia, was used to prepare the laminated bamboo. The bamboo was made into strips with a cross-section size of 5 × 20 mm and a length of 1500 mm ( until ). Then, the strips of bamboo were dried until it has <12% moisture content and then glued on using the Urea Formaldehyde of 268 g/m2 with 2 MPa cold forging for 4 h. First, 10 bamboo strips were glued together ( to construct three boards with a cross-sectional size of 20 × 50 mm () to construct the beam test specimens (). Further, the three boards were glued to each other to produce a beam (). Finally, the cross-sectional size and length were adjusted to construct a beam measuring 50 × 50 × 1500 mm ().
Figure 1. Preparation of laminated bamboo beam (a) bamboo stem, (b) bamboo splitting, (c) bamboo splits, (d) bamboo slats, (e) bamboo slats glue, (f) bamboo laminated board, (g) bamboo laminated board gluing, (h) gluing result, and (i) laminated bamboo beam.

Installation of CFRP on beams using Sikadur 732 brand epoxy resin adhesive following the location of CFRP on the beam. Installation of CFRP in the tensile section was carried out by applying adhesive on the surface of laminated bamboo and on the surface of CFRP are each 0.5 mm thick (). Then, the two surfaces were attached to each other and pressed at 2 MPa for 4 h (). Installation of CFRP in the compressive section was carried out by first making holes in the beam with a cross-sectional size of 2 × 21 mm with lengths of 0.2, 0.5, and 0.7 L (). Then, the hole was filled with adhesive until a half of the hole volume (), and then the CFRP was inserted in the hole that had been filled with adhesive while keeping the CFRP right in the center of the hole (). presents the photograph of the laminated bamboo beam test specimens, consisting of beams without CFRP, beams with CFRP in the tensile zone, beams in the compressive zone, and beams in the tensile and compressive zone (not visible in the photo).
Figure 2. CFRP installation process on laminated bamboo beams, (a) CFRP gluing in the tensile zone, (b) CFRP forging in the tensile zone, (c) punching the beam in the compressive zone, (d) pouring the adhesive, (e) CFRP installation in the compressive zone, and (f) the photograph of the laminated bamboo beam test specimens.

The independent variables in this study were the length and positioning of CFRP on the laminated bamboo beam. The CFRP length variations were 0.2, 0.5, and 0.7 L, with L representing the beam span. Meanwhile, the positioning of CFRP was on the tensile zone, compression zone, as well as the tensile and compression zone. In this study, we used a laminated bamboo beam with 50 × 50 × 1500 mm dimensions. CFRP was placed on the tensile part of the bamboo with a cross-section of 25.4 × 0.8 mm, while the treatment on the compression part was carried out on the bamboo with a cross-section of 20 × 0.8 mm. summarizes the codes for the sample beams’ dimensions, CFRP size, and CFRP positioning. The beam with B-0.2L-TC code was strengthened with CFRP with 0.2 times the beam span, and the CFRP was applied at the compression and tensile parts of the beams. Further, illustrates the detail of the CFRP application and the size of the beam samples. In each sample variation, we prepared three samples, so we had 18 samples in total.
Figure 3. Beam configuration: (a) B-CTRL, (b) B-Lo-TC, Lo = 0.2 L; 0.5 L; 0.7 L, (c) B-0.7L-C, (d) B-0.7L-T, and (e) longitudinal section.

Table 1. Sample size and code.
The flexural rigidity test was carried out using the four-point bending method based on the ASTM D 198-15, as shown in . The laminated bamboo beam was placed on hinged and roller support with a span of 1400 mm and a spreader beam distance of 200 mm. The load was identified using a load cell with 100 kN capacity and 0.5 N accuracy. Deflection at mid-span was measured with LVDT at 200 mm capacity and 0.01 mm accuracy. The reduction of support was measured using a dial gauge with 50 mm capacity and 0.01 mm accuracy.
Results and discussion
Results of material test
The results of the physical and mechanical properties of laminated bamboo, as well as the result of the tensile test on CFRP, are presented in .
Table 2. Physical and mechanical property.
Following ASTM D 2395-14, we obtained density test results on the laminated bamboo was 690 kg/m3 at 12.39% moisture content. This test result is linear with the study reported by Karyadi et al. (Citation2020) of 0.687 g/cm3 density. Besides, the mechanical properties obtained in this study, consisting of the tensile strength, compression strength, and elasticity, based on ASTM D 143, are in the same range as the results reported by Dauletbek et al. (Citation2022). The tensile strength test of CFRP was carried out referring to ASTM D 3039/D 3039 M–00, ‘Standard Test Method for Tensile Properties of Polymer Matrix Composite Materials’ by using a gauge length of 50 mm and a head displacement rate of 2.61 mm/min. Besides, we also identified CFRP’s tensile strength of 659.61 MPa and elasticity modulus of 31.58 GPa. In this study, we used CFRP with low quality, therefore, we gained a lower elasticity modulus than the elasticity modulus from CFRP with higher quality, such as the one adopted in a study by D’Ambrisi et al. (Citation2014), reporting an elastic modulus of 150 GPa. However, the CFRP in this study can serve as the reference for improving the laminated bamboo beam’s flexural rigidity due to the higher CFRP elasticity modulus than the elasticity modulus of the laminated bamboo. In this study, we examined the physical and mechanical properties of laminated bamboo using ASTM for the wood’s physical and mechanical properties due to the absence of code for laminated bamboo, as well as the similar physical and mechanical properties of bamboo and wood.
Relationship between load and deflection
The amount of load and deflection were two primary data garnered in this study. The loading process was carried out in stages, resulting in failure experienced by the laminated bamboo beam. The beam loading failure is caused by the maximum load on the beam. The relationship between the load and deflection is illustrated in . describes the maximum load detained by the beam (not being discussed in this study) and beam flexural rigidity. Generally, the flexural rigidity of reinforced beams is higher than that of unreinforced beams. The flexural rigidity of the beam with strengthening treatment (lines in color other than black) is represented in a more vertical line than the line of the beam with no strengthening treatment (black line).
Flexure rigidity of beams
The flexural rigidity of the beams was calculated using Formula (1), with data of the load (F) and the deflection in the midspan of the beam (δ) on the elastic-linear areas, as presented in . The elastic-linear area represents the relationship between load (F) and deflection in the midspan of the beam (δ) forms a straight line. The elastic-linear region was determined by placing a straight line on the curve of the relationship between load (F) and deflection at mid-span (δ). The points that coincide with the straight line were selected as linear regression. If the coefficient of determination (R2) obtained from the linear regression was ≥0.95, the selected points were in the elastic-linear region. If the obtained R2 was <0.95, then some reduction was made at the upper end of the selected points until an R2 reached at least 0.95. Further, at the bottom end of that line, we observed a pair of F-δ, which is further referred to as bottom F elastic-linear and bottom δ elastic-linear. Meanwhile, on its top end, we secured an F-δ pair, referred to as the top F elastic-linear and top δ elastic-linear. The calculated flexural rigidity on each beam sample is summarized in .
Table 3. Flexural rigidity of beams.
shows a graphical presentation of the flexure rigidity of each test beam and also the percentage increase in flexure rigidity of the beam with CFRP reinforcement compared to the beam without CFRP reinforcement. The flexural rigidity increases following the higher CFRP length. The more significant flexural rigidity increased from the beam added with CFRP than the control beam is also in accordance with the greater CFRP length. Therefore, the CFRP length accelerates the laminated bamboo’s flexural rigidity. From the obtained increase results from every greater CFRP length, then the plan for CFRP length addition for the expected flexural rigidity. Besides, the generated graphic also presents the effects of CFRP position toward the beam flexural rigidity. The beam treated with CFRP on its compression part only attains the lowest increase than the beams applied with CFRP on their tensile part only, as well as on the tensile and compression parts, simultaneously.
Effects of CFRP length on the beam flexural rigidity
The influence of CFRP length on the flexure rigidity of beams can be observed from the regression of the CFRP length data and the beam flexural rigidity, as shown in . In this study, we selected linear regression due to its higher coefficient of determination (R2) than the non-linear regression. Accordingly, the enhancement of flexural rigidity is linearly proportional to the CFRP length, with the Formula of y = 0.608x + 1.192, the y multiplied by 1010 representing the beam flexure rigidity and x is the ratio of CFRP length toward the beam span. That Formula has a coefficient of determination of R2 = 0.6522. The finding from this Formula was further used in determining CFRP length, following the expected flexural rigidity.
The trend of CFRP impact in enhancing the beam flexural rigidity can also be identified from the regression between the CFRP length and the average increased percentage of flexural rigidity compared to the control beam, as presented in . The linear regression was chosen as it shows the highest determination coefficient in comparison to the other regressions. The regression with Formula y = 51.835x + 1.6394, with y representing the average increase percentage of flexural rigidity and x showing the CFRP length ratio toward the beam span and R2 = 0.9727, can be used in preparing particular the flexural rigidity level.
The findings observed in this study are within the same ranges as the results discovered in previous studies. presents the B-CTRL or beam sample without treatment, at 50 × 50 × 1400 mm dimension and rigidity of 1.17 × 1010 N.mm2. Nadir et al. (Citation2016) conducted a similar study using a timber beam at 40 × 60 × 830 mm dimension, reporting flexural rigidity of 7.19 × 109 N.mm2. Meanwhile, Bhat (Citation2021) discovered that a timber beam with no treatment at 70 × 120 × 1524 mm dimension had 1.01 × 1010 N.mm2 flexural rigidity. In this study, we found slightly different flexural rigidity than those studies that is possibly caused by different dimensions and materials. Bhat (Citation2021) adopted timber with an elastic modulus of 10 GPa, Nadir et al. (Citation2016) used timber with an elastic modulus of 8.68 GPa, and in this study, we used laminated bamboo with an elastic modulus of 13.02 GPa.
The increase in beam rigidity identified in this study is directly proportional to the length of CFRP. The greatest improvement of flexural rigidity of 38.68% was observed on the beam with a CFRP of 0.7 times the beam span length. With the length of CFRP being the same as the length of the beam, as illustrated in , the predicted beam flexural rigidity reaches 53.47%. This finding is also similar to the result from previous studies. Vahedian et al. (Citation2019) conducted research using a wooden beam at 45 × 90 × 1620 mm dimension and 245 GPa elasticity modulus with FRP on its tensile area. The FRP length of 1000 and 1300 mm produced 31 and 45% flexural rigidity in comparison to the beam with no FRP. Nadir et al. (Citation2016) also conducted a study on laminated bamboo at 40 × 60 × 830 mm dimension with FRP strengthening on the tensile area along with the beam span and variation of thickness of one and two layers. That study reported a rigidity increase of 36.19 and 64.12%, respectively, for one and two layers of FRP.
Effects of CFRP position on the beam flexural rigidity
The CFRP being applied on the tensile area (B-0,7L-T), compression area (B-0,7L-C), as well as on tensile and compression area simultaneously (B-0,7L-TC) enhance the laminated bamboo beam flexural rigidity. In comparison to the control (B-CTR), the beam with CFRP in the compression area presented the lowest flexural rigidity increase of 24.25%, followed by the beam with CFRP in the tensile area (28.79%), and the highest flexural rigidity was recorded from the sample being applied with CFRP in both of its tensile and compression areas (38.86%). The beam with CFRP only on the compression area presents a lower increase in flexural rigidity due to the early buckling of the CFRP in that section (Yang et al., Citation2016). presents the detailed results of the CFRP position’s effect on flexural rigidity.
The different levels of flexural rigidity between the beams given CFRP on the compression areas only and tensile areas only, with the ones applied with CFRP on both compression and tensile areas, are 14.61 and 10.07%, respectively. Meanwhile, between the samples given CFRP on their tensile areas only and compression areas only is 4.56%.
Wei et al. (Citation2017), investigating the strengthening of scrimber bamboo beam using one layer CFRP on the tensile area, reported the increase results of 23.2% (2.65 × 108 kNmm2) from the control, with the rigidity of 2.15 × 108 kNmm2.
Further, Yang et al. (Citation2016), using a GFRP sheet on the tensile area, reported an increase of beam flexural rigidity of 4.9% (2.16 × 1012 N.mm2), while the application of GFRP on the tensile and compression area resulted in 6.6% (2.19 × 1012 N.mm2) from the control beam with the rigidity of 2.06 × 1012 N.mm2. The flexural rigidity found on the sample given treatment on the tensile and compression area is 1.7% higher than the flexural rigidity from the sample provided treatment on its tensile area only.
By using FRP in the tensile section only, as well as in the tensile and compressive sections, Yeboah and Gkantou (Citation2021) reported an increase in the flexural rigidity of timber beams by 22–33% compared to beams without reinforcement. Furthermore, it also reported that the increase in flexural rigidity between beams reinforced with FRP in the tensile and compressive sections against beams reinforced with FRP in the tensile section only amounted to 2%. Thus, the addition of FRP in the compressive section only contributes slightly to the increase in flexural stiffness.
Kliger et al. (Citation2007) constructed a non-linear model to identify the effects of strengthening in the beam’s tensile area and or the compression area of the glued laminated timber with different treatment percentages. The modeling results suggest the highest rigidity from the same treatment applied to the tensile and compression area.
The results from this study indicate that the addition of CFRP to laminated bamboo beams increases flexural rigidity, thereby, enhancing the amount of service load. For this reason, the addition of CFRP on laminated bamboo beams for building structures is highly recommended. Further, this study also suggests the addition of CFRP to repair installed laminated bamboo beams to improve their flexural rigidity.
Conclusion
The addition of CFRP at different lengths on the laminated bamboo beam at the tensile and compression zone improves its flexural rigidity significantly. The increase in flexural rigidity of beams with CFRP lengths of 0.2, 0.5, and 0.7 L are 15.36, 24.90, and 38.86%, respectively, calculated against the control beam. The amount of treatment is directly proportional to the CFRP length, following the formula y = 0.608x + 1.192, with y multiplied by 1010, showing the beam flexural rigidity and x being the CFRP length ratio toward the beam span. Placement of CFRP in the tensile zone, compressive zone, and in both tensile and compressive zone has been observed to significantly increase the flexural rigidity of the beams. The lowest increase in flexural rigidity occurred in beams given CFRP in the compression zone by 24.25%, followed by beams treated with CFRP in the tension zone of 28.79%, and the highest flexural rigidity was observed in beams that were given CFRP in the tension and compression zone of 38.86%, respectively, calculated against the control beam. The flexural rigidity of control beams and those with CFRP added to the tensile and compressive sections with lengths of 0.2, 0.5, and 0.7 L are 1.17 × 1010, 1.35 × 1010, 1.47 × 1010, dan 1.63 × 1010 Nmm2, respectively. The magnitude of the flexure rigidity of the beam with the addition of CFRP in the compressive zone only, in the tensile zone only, and in the tensile and compressive zone are 1.46 × 1010, 1.51 × 1010, and 1.63 × 1010 Nmm2, respectively.
Disclosure statement
No potential conflict of interest was reported by the author(s).
Additional information
Funding
Notes on contributors

Karyadi
Karyadi is a lecturer at the Department of Civil Engineering and Planning, Faculty of Engineering, Universitas Negeri Malang, with the Building Structure study group.

Natasya Aqnes Sindi Safira
Natasya Aqnes Sindi Safira is a student in the same department writing her thesis.

Roro Sulaksitaningrum
Roro Sulaksitaningrum is a lecturer at the Department of Civil Engineering and Planning, Faculty of Engineering, Universitas Negeri Malang, with the Building Structure study group.

Nindyawati
Nindyawati is a lecturer at the Department of Civil Engineering and Planning, Faculty of Engineering, Universitas Negeri Malang, with the Building Structure study group. The four of us researched Improving the Flexural Rigidity of Laminated Bamboo Beams Using Fiber Reinforcement Polymer with funding from the State University of Malang. This research is part of our research project that examines the use of laminated bamboo for building structures. This project examines the Building Structure of laminated bamboo, including beams, columns, plates, walls, and connections between structural elements.
References
- Al‑Katib, H. A. A., Alkhudery, H. H., & Al‑Tameemi, H. A. (2022). Structural behavior of standard timber beams strengthened using CFRP Sheet. Asian Journal of Civil Engineering, 23(5), 727–739. https://doi.org/10.1007/s42107-022-00452-w
- Andor, K., Lengyel, A., Polgár, R., Fodor, T., & Karácsonyi, Z. (2015). Experimental and statistical analysis of spruce timber beams reinforced with CFRP fabric. Construction and Building Materials, 99(2015), 200–207. https://doi.org/10.1016/j.conbuildmat.2015.09.026
- ASTM (2000). D 3039/D 3039M-00, standard test method for tensile properties of polymer matrix composite materials. American Society for Testing Material Standard.
- ASTM (2014). D 143-standard test method for small clear specimen of timber. American Society for Testing Material Standard.
- ASTM (2014). D 2395-14, standard test methods for density and specific gravity (relative density) of wood and wood-based materials. American Society for Testing Material Standard.
- ASTM (2015). D 198-15-standard test methods of static tests of lumber in structural sizes. American Society for Testing Material Standard.
- Bhat, J. A. (2021). Effect of FRP-reinforcement variation on the strength parameters of different timber beams. Materials Today: Proceedings, 44, 2785–2791. https://doi.org/10.1016/j.matpr.2020.12.783
- Corradi, M., Mouli Vemury, C., Edmondson, V., Poologanathan, K., & Nagaratnam, B. (2021). Local FRP reinforcement of existing timber beams. Composite Structures, 258(August 2020), 113363. https://doi.org/10.1016/j.compstruct.2020.113363
- Corradi, M., Vo, T. P., Poologanathan, K., & Osofero, A. I. (2018). Flexural behaviour of hardwood and softwood beams with mechanically connected GFRP plates. Composite Structures, 206, 610–620. https://doi.org/10.1016/j.compstruct.2018.08.056
- D’Ambrisi, A., Focacci, F., & Luciano, R. (2014). Experimental investigation on flexural behavior of timber beams repaired with FRP plates. Composite Structures, 108(1), 720–728. https://doi.org/10.1016/j.compstruct.2013.10.005
- Dauletbek, A., Li, H., Lorenzo, R., Corbi, I., Corbi, O., & Ashraf, M. (2022). A review of basic mechanical behavior of laminated bamboo lumber. JRM, 10(2), 273–300.
- Gao, D., Chen, B., Wang, L., Tang, C., & Yuan, P. (2022). Comparative study on clear specimen strength and member strength of side-pressure laminated bamboo. Advances in Civil Engineering, 2022, 1–11. https://doi.org/10.1155/2022/2546792
- Gere, J. M. (2004). Mechanics of materials (6th ed.). Thomson Learning Singapore.
- Kamarudin, M. H., Saringat, M. S., & Sulaiman, N. H. (2016). The study of mechanical properties of laminated bamboo strip (LBS) from Gigantochloa Levis type mixed with epoxy. Jurnal Teknologi (Sciences & Engineering), 78(5–10), 59–64. https://doi.org/10.11113/jt.v78.8836
- Karyadi, & Susanto, P. B. (2017). Mechanical characteristics of box-section beam made of sliced-laminated Asian bamboo (Dendrocalamus asper) in bending failure mode under transversal load. AIP Conference Proceedings, 1887 (October), 020062. https://doi.org/10.1063/1.5003545
- Karyadi, K., Prayogo, B., Nindyawati, N., & Susanto, P. B. (2020). Pull-out resistance parallel to grain of threaded steel rod glued-in glubam with edge distance variation. Research Journal of Applied Sciences, Engineering and Technology, 17(1), 1–6. https://doi.org/10.19026/rjaset.17.6027
- Kliger, K., Al-Emrani, M., Johansson, M., & Crocett, R. (2007). Strengthening glulam beams with steel or CFRP plates. In Proceedings of the First Asia-Pacific Conference on FRP in Structures: APFIS 2007.
- Li, H. T., Deeks, A. J., Zhang, Q. S., & Wu, G. (2015). Flexural performance of laminated bamboo lumber beams. BioResources, 11(1), 929–943. https://doi.org/10.15376/biores.11.1.929-943
- Liu, Q., Ma, S., & Han, X. (2020). Study on the flexural behavior of poplar beams externally strengthened by BFRP. Journal of Wood Science, 66(1), 40. https://doi.org/10.1186/s10086-020-01887-y
- Nadir, Y., Nagarajan, P., Ameen, M., & Arif M, M. (2016). Flexural stiffness and strength enhancement of horizontally glued laminated wood beams with GFRP and FRP composite sheets. Construction and Building Materials, 112, 547–555. https://doi.org/10.1016/j.conbuildmat.2016.02.133
- Raftery, G. M., & Whelan, C. (2014). Low-grade glued laminated timber beams reinforced using improved arrangements of bonded-in GFRP rods. Construction and Building Materials, 52(2014), 209–220. https://doi.org/10.1016/j.conbuildmat.2013.11.044
- Rusch, F., Trevisan, R., Hillig, E., & Mustefaga, E. C. (2019). Physical-mechanical properties of laminated bamboo panels. Pesquisa Agropecuária Tropical, 49, e53714.
- Sulastiningsih, I. M., Trisatya, D. R., Indrawan, D. A., Malik, J., & Pari, R. (2021). Physical and mechanical properties of glued laminated bamboo lumber. Journal of Tropical Forest Science, 33(3), 290–297. https://doi.org/10.26525/jtfs2021.33.3.290
- Vahedian, A., Shrestha, R., & Crews, K. (2019). Experimental and analytical investigation on FRP strengthened glulam laminated timber beams: Full-scale experiments. Composites Part B: Engineering, 164(August 2018), 377–389. https://doi.org/10.1016/j.compositesb.2018.12.007
- Wdowiak-Postulak, A., Wieruszewski, M., Bahleda, F., Prokop, J., & Brol, J. (2023). Fibre-reinforced polymers and steel for the reinforcement of wooden elements—Experimental and numerical analysis. Polymers, 15(9), 2062. https://doi.org/10.3390/polym15092062
- Wei, Y., Ji, X., Duan, M., & Li, G. (2017). Flexural performance of bamboo scrimber beams strengthened with fiber-reinforced polymer. Construction and Building Materials, 142, 66–82. https://doi.org/10.1016/j.conbuildmat.2017.03.054
- Wei, Y., Zhou, M. Q., & Chen, D. J. (2015). Flexural behaviour of glulam bamboo beams reinforced with near surface mounted steel bars. Materials Research Innovations, 19(sup1), S1-98–S1-103. https://doi.org/10.1179/1432891715Z.0000000001377
- Yang, H., Liu, W., Lu, W., Zhu, S., & Geng, Q. (2016). Flexural behavior of FRP and steel reinforced glulam beams: Experimental and theoretical evaluation. Construction and Building Materials, 106(2016), 550–563. https://doi.org/10.1016/j.conbuildmat.2015.12.135
- Yeboah, D., & Gkantou, M. (2021). Investigation of flexural behaviour of structural timber beams strengthened with NSM basalt and glass FRP bars. Structures, 33(6), 390–405. https://doi.org/10.1016/j.istruc.2021.04.044
- Zhao, K., Wei, Y., Yan, S., Chen, S., & Dong, F. (2021). Experimental and analytical investigations on flexural behavior of bamboo beams strengthened with steel bars. Advances in Structural Engineering, 24(14), 3338–3356. https://doi.org/10.1177/13694332211026230