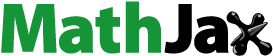
Abstract
The widespread use of steel in concrete has become a challenge as the emissions of carbon monoxide during steel production pollute the environment. The use of a conventional method of reinforcement has many limitations that still affect the total arrest of the concrete issue. This research aims to identify ways in which concrete strength can be improved without the conventional method. The objective of this research was to investigate the compressive strength (), slump value, water absorption, and density of basalt fiber hybridized concrete. Experimental methods were used where a total number of 36 concrete cube specimens were prepared. It was discovered that hybrid fiber concrete (B4) obtained the highest
on days 7, 14, and 28 of curing at 14.473, 17.844, and 24.632 N/mm2, respectively while on day 28, the
of concrete mixes B1 was 9.330 N/mm2, B2 was 16.780 N/mm2, B3 was 13.991 N/mm2. Hybrid fiber concrete (B4) obtained a slump value of 50 mm which is higher than the other concrete mix with B1 obtaining 25 mm which is the lowest slump value. The concrete B3 had the lowest density of 1931 kg/m3 followed by B4 concrete with 2047 kg/m3 while B2 had the highest density of 2099 kg/m3. Concrete mix B1 had the highest water absorption rate of 3.5% while B4 had the lowest water absorption rate of 1.4%. In general, B4 proved to offer better advantages over the other concrete mixes and can for flooring and crack resistance and curing.
Reviewing editor:
1. Introduction
Concrete is reinforced to improve the strength. It is a common practice to use reinforcement in the form of continuous rebars or short discrete fibers to improve the brittle behavior of cementitious materials because they have a limited tensile strength and strain capacity. To combat the problem of concrete’s brittleness and insufficient resistance to fracture initiation and propagation, short, randomly arranged fibers are frequently used (Bentur & Mindess, Citation2022) but some studies have presented studies on the use of basalt fiber-reinforced polymer sheets to retrofit concrete structural members (Shen et al., Citation2022). For materials that perform better in terms of tensile strength, ductility, toughness, and durability, fibers may be added to cement-based materials (Jamshidi & Karimi, Citation2010; Vandewalle, Citation2007) as well as rebars like basalt fiber rebar (Shi et al., Citation2023). An improvement is made by halting or delaying fracture initiation, growth, or coalescence (Yao et al., Citation2003). Numerous fiber types, including metallic, polymeric, and natural fibers, are frequently used in concrete engineering. Fiber-reinforced concrete (FRC), which is defined by ACI Committee 544 as concrete made of hydraulic cement comprising of fine or fine and coarse aggregates and discontinuous discrete fibers, will be the corresponding material when short discrete fibers are used.
FRC is frequently thought of as a composite material that consists of two phases, with concrete serving as the matrix phase and fiber as the inclusion phase. Although the volume fractions and properties of the individual phases influence the behavior of the composite, the volume fraction, geometry, and mechanical characteristics of the fibers are frequently more significant. The properties of the composite will only be slightly improved by reinforcing concrete with a single type of fiber, depending on these variables. In all conventional fiber-reinforced concrete products, a single type of fiber is utilized (SulphiaBeevi et al., Citation2020). The single fiber has a limited range of strain and effectiveness in opening fractures. Therefore, a specific type of fiber can only enhance the strength or ductility of cementitious composites (Sharma et al., Citation2009). Although some fibers with high modulus and high strength, such as carbon, polyvinyl alcohol, steel, asbestos, and glass, can significantly increase concrete’s strength, their inherent brittleness prevents concrete from becoming more ductile (Halvaei et al., Citation2014). The ductility and cracking resistance of low-strength fibers like nylon, acrylic, and polypropylene are improved (Halvaei et al., Citation2015). Reinforcement corrosion and seismic damage considerably affect the seismic performance of reinforced concrete (RC) members (Li et al., Citation2022). However, using the concept of hybridization, a logical combination of two or more types of fibers, more desirable engineering properties of the composite could be achieved from a potential synergetic response of the various fibers.
Cementitious composites offer a variety of cracking reactions at different loading stages because they use two or more mixed fibers. The resultant fiber-reinforced concrete that contains a mixture of two or more fibers is known as hybrid FRC. As a result, different kinds of fibers are added to hybrid fiber-reinforced concretes, which result in hybrid performances that are superior to the sum of those of the individual fibers (Banthia & Soleimani, Citation2005). The hybrid composite exhibits a synergistic reaction and gains advantages from each of its constituent fibers (Shu et al., Citation2015). Studies show that combining two or more types of fibers to create hybrids produces cementitious composites with greater ultimate strengths, strain capacities, and strain-hardening behavior (Ahmed & Mihashi, Citation2011; Banthia & Sappakittipakorn, Citation2007). One of the numerous hybridization strategies involves combining different fiber lengths, diameters, moduli, and tensile strengths (Ahmed & Maalej, Citation2009; Silva et al., Citation2013).
By acting as a bridge, the fibers can stop surface cracking, increasing the concrete’s ability to withstand impacts. Combining two or more different fibers (different fiber types and/or geometries) is becoming more popular to improve the behavior of the entire system (Vairagade et al., Citation2012). The goal is for these hybrid systems’ performance to be better than what each fiber type could produce on its own. In other words, there would be a synergy. According to the mechanisms involved, the study (Banthia & Gupta, Citation2004) divided these synergies into three groups:
Hybrids are based on the fiber constitutive response, in which one fiber is more ductile and provides toughness at high strains while the other is stronger, stiffer, and provides strength (Banthia & Gupta, Citation2004).
Fiber-based hybrids in which one fiber is very small and controls microcracks during the initial stages of loading while the other is larger and acts as a bridging mechanism across macrocracks.
Fiber-based hybrids, in which one type of fiber contributes to the strength or toughness of the hardened composite and the other type contributes processing-friendly fresh mix properties.
In contrast to long fibers, which are more frequently used to increase the mechanical properties of concrete, short fiber types greatly increase the number of fibers used in concrete and are used to decrease cracking and increase durability depending on the properties of the materials used. Hybrid fiber addition improved the concrete’s overall performance and led to similar notable improvements in mono-fiber reinforced concrete with a higher total content (Qian & Stroeven, Citation2000).
Yao et al. (Citation2003) investigated the effects of fiber hybridization on the mechanical characteristics of concrete and concluded that a composite with superior mechanical properties can be obtained through the combination of fibers with different properties. The benefits of hybrid fiber systems were discussed by Bentur and Mindess (Citation2006), who also explained how the presence of a durable fiber can increase strength and/or toughness after aging. Khalil (Citation2013) investigated the mechanical characteristics of concrete reinforced with high-performance fibers. As the fiber volume fraction increased, the addition of steel fibers led to a slight increase in high-performance concrete’s (HPC's) compressive strength (). High-performance carbon fiber concrete’s mechanical properties were investigated by Khalil and Abdulrazaq (Citation2011). The outcomes demonstrated that the mechanical qualities of HPC were enhanced by the addition of carbon fibers.
Mohankar (Citation2016) used steel and polypropylene fibers in various proportions (0.1, 0.5, 0.7, and 1%) to study the mechanical effectiveness of hybrid fiber-reinforced concrete (HFRC). The role of the crack resistor would be played by the addition of small, evenly distributed, and closely spaced fibers to the concrete, greatly improving its properties (Mohankar, Citation2016). A volume fraction of carbon fiber of 0.10, 0.20, and 0.30% and steel fiber of 0.50, 1.0, and 1.50% were studied for the and impact toughness of steel fiber reinforced concrete (SFRC), HFRC, and carbon fiber reinforced concrete (CFRC). According to the results, adding steel and carbon fibers may increase
Compressive strength may be significantly improved by CF, SF, and hybridization between them. Results of impact tests have demonstrated that as fiber volume increases, the impact number of the first visible crack and ultimate failure also rises (Song & Yin, Citation2016).
According to their size (length and diameter), the most common fibers used in the construction of concrete structures are divided into micro and macro fibers. According to the material, micro-fibers are divided into three categories: carbon fiber (CF), glass fiber (GF), and basalt fiber (BF) (Chiadighikaobi et al., Citation2022; Ivorra et al., Citation2010; Ralegaonkar et al., Citation2018; Tassew & Lubell, Citation2014). Micro-fibers have a length of 5–10 mm and a diameter of 7–30 micrometers. However, macro-fibers, which include steel fiber (SF) and polyvinyl alcohol fiber (PVAF), have a length of 25–60 mm and a diameter of 0.2–0.8 mm (Abbas Ashour Alaraza et al., Citation2022; Atahan et al., Citation2013; Pakravan & Ozbakkaloglu, Citation2019).
depicts the behavior of the hybrid fibers concerning crack bridging that occurs in the cement matrix. The micro-cracks are crosslinked at the micro-crack stage (), and macro-cracks are crosslinked at the macro-crack stage (), which is expected to improve flexural performance and impact resistance. To put it another way, relatively long macro-fibers are better at controlling large cracks than short micro-fibers are at controlling micro-cracks (Markovic, Citation2006; Pakravan et al., Citation2017; Vandewalle, 2007).
Figure 1. Bridging action of the hybrid with different sizes; (a) first phase of loading, and (b) second phase of loading (Park et al., Citation2022).

It has been identified that most of the hybrid fiber-reinforced concrete was prepared with the combination of two or more fibers of different materials. No research was identified on the use of micro and macro basalt fiber of different lengths and diameters as hybrid fiber for concrete reinforcement. Also, the use of black wood charcoal in basalt hybrid fiber as a concrete additive is a new feature in concrete construction though there is a series of research on the use of black wood charcoal in concrete. From the review of previous studies, it could be understood that that is no information on the possible use of macro basalt fiber and micro basalt fiber as hybrid fiber for the reinforcement of concrete. These two types of basalt fiber are in different shapes, lengths, and diameters and were incorporated into the concrete mix. This is a new method in concrete design and construction. Though both fibers are of the same origin their properties differ. This will give the concrete matrix better performance to produce better concrete properties. In the event of promoting the sustainability of concrete, the use of wood black charcoal powder as a concrete additive was implemented. This type of additive is an organic type that the production did not need the use of chemicals. To overcome the limitations associated with the production of sustainable concrete with good strength for construction, the hybridization of basalt fiber was used to improve the physical and mechanical properties of concrete. It is worth noting that several studies have stated that a high dosage of basalt fiber (micro basalt fiber) especially >1% leads to fiber clumping which produces unwanted cavities and pores in concrete matrix but this study presents hybrid basalt fiber of 1.5% (0.75% of macro and 0.75% of micro basalt fiber) by volume of cement. the optimum dose is important as the greater quantity unfavorably impacts the strength properties of concrete due to a deficiency of flowability. The typical range of the ideal amount of BFs fluctuates from 0.5 to 1.5% (Al-Kharabsheh et al., Citation2022). Macro basalt fiber which does not have the same texture and body surface as the micro basalt fiber will reduce the high unwanted cavities and pores caused by soft basalt fiber (micro basalt fiber).
The steps to achieving the objectives of this research are illustrated in of this study.
2. Experimental materials and methods
2.1. Materials for experiments
This research study used the below-listed materials to produce the concrete mixes:
2.1.1. Cement
Dangote Portland cement was used as the binder in the concrete. This cement was obtained from a local cement dealer in Ado-Ekiti, Ekiti State. The chemical composition of Dangote cement used in this study and compared with the BS limit is illustrated in .
Table 1. Chemical composition of Dangote cement, %.
River sand of sizes 0.4–1 mm was used as the concrete aggregate which accommodated fine aggregate and coarse aggregate. This sand was gotten from sand dealers in Lagos Nigeria.
2.1.2. Additive
Wood black charcoal powder () and Rice husk ash () were used as the additive to produce concrete with enhanced properties. The chemical composition of black wood charcoal powder is stated in while the composition of the rice husk ash is stated in .
Table 2. Chemical composition of wood charcoal (Laosombatthawee et al., Citation2015).
Table 3. The composition of the rice husk ash (Korotkova et al., Citation2016).
Superplasticizer was used as the water-reducing agent. The superplasticizer used was Sika visco-create 2000. It was obtained from Sika Manufacturing Nigeria. Sika ViscoFlow®-2000 (GB) is a liquid admixture for concrete based on unique polycarboxylate polymer technologies. Sika ViscoFlow®-2000 (GB) is designed as a high-range water reducer or superplasticizer. It is particularly suited for use in concretes that require low water/cement ratios and/or high-water reductions with excellent workability retention properties of up to 3 h. Sika ViscoFlow®-2000 (GB) meets the requirements of BS EN 934-2 Table (BS EN 934-2:2009 + A1, Citation2012).
For the concrete dispersed reinforcement, macro basalt fiber (Basalt minibars) () and micro basalt fiber (chopped soft basalt fiber) () were used. The basalt minibar and micro basalt fiber were purchased from EccoTexx company, Lipetsk, Russia. The characteristics and properties of this basalt microfiber and basalt minibars are stated in .
Table 4. Characteristics and properties of the basalt microfiber (Abbas Ashour Alaraza et al., Citation2022; Cemmix, Citationn.d.; Fiber is basalt, Citationn.d.).
2.1.3. Water
Clean tap water for mixing the concrete aggregates was obtained from ABUAD water.
The components of the concrete mix are illustrated in , where the materials used in this research and their rates are indicated.
Table 5. Fabrication of concrete mix.
2.2. Laboratory experimental procedures
To achieve the aims and objectives of this research study, 4 (four) concrete mix series were prepared bearing individually dispersed reinforcement as 0% basalt fiber, 1.5% micro basalt fiber, 1.5% macro basalt fiber, and 1.5% basalt fiber (0.75% micro basalt fiber and 0.75% macro basalt fiber). This is expressed in with the concrete mix series acronyms. Since the objective of this research study is not the selection or identification of the optimum basalt fiber percentage that produces the best strength, this study selected this specific percentage of 1.5% basalt fiber since it falls within the optimum basalt fiber range according to a series of research where the optimum basalt fiber percentage ranges from 0.5 to 1.5% (Al-Kharabsheh et al., Citation2022) and based on the research study (Ding et al., Citation2023; Ramesh & Eswari, Citation2021) 1.5% BF obtained the best compressive strength at day 28.
Table 6. Basalt fiber content per concrete mix series.
To mix the concrete, all of the aggregates, basalt fibers, and water were successively added to the mixer and thoroughly mixed for 1 min for each additional ingredient shown. To ensure that the basalt fibers in the concrete were distributed evenly, the addition of fiber was not poured into the mixer in lumps, then the time for mixing the concrete was set at 3 min. Also, the type of superplasticizer used in the concrete mix helped to improve the concrete workability.
After preparing each concrete mix series, a slump test was conducted on it to ascertain its workability. Then the concrete pastes were cast in the concrete molds of dimensions 100 × 100 × 100 mm. After casting the pastes in the mold, they were covered in the laboratory under room temperature °C for 24 h then the hardened concrete cubes were demolded and cured for 7, 14, and 28 days before testing for compression.
18 (Eighteen) concrete cube specimens () were prepared from each concrete mix series and three specimens per each testing day to obtain their compressive strength (). Before crushing the concrete cubes, they were weighed to obtain their weight for density. In like manner, 18 concrete cube specimens were prepared for water absorption tests. After each test on the three specimens per each testing day, the average data was taken for analysis. This research investigated for
test, density (
) test, water absorption (WA) test. The slump test was done following BS 1881-102: 1983 (British Standard Institution, Citation1983a). The water absorption test was done following BS 1881-122: 1983 (British Standard Institution, Citation1983b).
3. Research study results
3.1. Slump value
The procedure for the slump test is shown in . The slump values measured for each of the concrete mixes are illustrated in and . The slump value was measured to the nearest 5 mm.
Table 7. Slump values at each concrete mix series.
According to and , concrete mix B1 had the lowest slump value of 25 mm, and mix B4 had the highest slump value of 50 mm. Low workability for mixes having slumps 10–40 mm is typically used for foundations with light reinforcement. Medium workability mix with slump 50–90 mm is typically used for normal reinforced concrete placed with vibration, high workability concrete with slump above 100 mm is typically used for closely spaced rebars and/or used when concrete needs to flow over long distances (Concrete slump test, Citationn.d.). It was observed that the workability of a concrete mix increased with the addition of Macro basalt fiber and Micro Basalt Fiber.
3.2. Water absorption
The water absorption property of the three categories of fiber-reinforced concrete considered in this research is presented in and . At 28 days, total water absorption of concretes was between 1.4 and 3.5%. The concrete with a volume of macro fiber and micro basalt fiber shows higher water absorption. More specifically, it was noted that the micro basalt fiber reinforced concrete had better resistivity to water penetration than the macro fiber reinforced concrete as well as the Hybrid fiber (macro basalt fiber and micro basalt fiber) reinforced concrete. For the conventional concrete mix (B1), the absorption rate measured was 3.5% which is the highest absorption rate. While the lowest absorption rate was B3 (1.4%). The water absorption test has also demonstrated the positive effect of hybridization in fiber-reinforced concrete. The results of the research have indicated that concrete reinforced with macro fiber and micro basalt fiber (Hybrid fiber) or higher volume fractions of hybrid fiber is likely to absorb more water.
Table 8. Water absorption at different concrete mix.
3.3. Concrete density
The concrete with densities of 2083, 2099, and 2047 kg/m3, exhibited a relatively moderate density ( and ). It appeared to have good workability during the mixing process and was easy to handle. The resulting concrete had a balanced weight while still providing sufficient strength for typical construction applications. However, the concrete with a density of 1931 kg/m3 displayed a noticeably lower density than the previous samples. This suggests that lightweight aggregates may have been used in the mixture to reduce the weight. As a result, this concrete may offer advantages, such as improved thermal insulation properties and reduced dead load on the structure.
Table 9. Density at different concrete mix series.
3.4. Concrete compressive strength
The most crucial aspect of concrete is its strength, but other qualities may also be crucial and must not be overlooked. Because strength is directly connected to the structure of hardened cement paste, strength is a crucial measure of quality. Strength has a high correlation with the water-to-cement ratio of the concrete, which in turn affects durability, dimensional stability, and other qualities of concrete, even though strength is not a direct indicator of durability or dimensional stability. As illustrated in and , the compressive strength of all concrete mixes increased with an increase in concrete age. Concrete mix B4 got the highest compressive strength on day 28 of curing with 24.632 N/mm2. The lowest compressive strength obtained was concrete mix B1 at 7.674 N/mm2. The result shows that there is an increase in compressive strength due to the hybridization of basalt fiber. Comparing the compressive strength difference by percentage between the three concrete mix series (B2, B3, and B4) bearing basalt fiber percentages about B1, it is seen that on day 28, B4 obtained a 62.12% increase, B3 obtained a 33.31% increase, B2 obtained a 44.4% increase. Checking the rate at which the strength of each concrete mix increased by the 28th day in comparison with Day 7, the strength of B1 grew by 17.75% while the strength of B2 grew by 21.57%, B3 grew by 26.03% while the strength of B4 grew with 41.24%.
Table 10. Concrete compressive strength of the hardened concrete mix cubes.
4. Conclusions
The investigations of the effect of hybrid fiber on concrete reinforcement have provided valuable insights into the potential benefits and limitations of using hybrid fiber in concrete structures. The study aimed to evaluate the mechanical properties and performance of concrete reinforced with hybrid fibers compared to traditional concrete reinforcement methods. Through comprehensive testing and analysis, several key conclusions can be drawn.
The incorporation of hybrid fiber in concrete has shown a significant improvement in the mechanical properties of the material. The hybrid fiber system combines the advantages of different sizes and texture fiber types to enhance the overall performance of the concrete. The hybridization resulted in improved compressive strength of the concrete, which is a crucial factor for the durability and longevity of structures.
Concrete with hybrid fiber proved to enhance the speedy growth of the compressive strength where it grew 41.24% at day 28 about day 7 while B1, B2, and B3 grew 17.75, 21.57, and 26.03%, respectively.
Concrete with dispersed hybrid fiber had the highest slump value of 50 mm while the concrete without fiber had the lowest slump value of 25 mm.
A water absorption rate of 3.5% was obtained in the concrete without fiber followed by hybrid fiber concrete with a water absorption rate of 3.2%. Concrete with macro fiber had the lowest rate of 1.2%.
Incorporation of macro basalt fiber in the concrete mix (B3) gave the lowest density of 1931 kg/m3 while B2 had the highest density of 2099 kg/m3.
The use of micro and macro basalt fiber has provided many answers to the challenging questions found in concrete construction and usage. The findings of this investigation will contribute to the existing body of knowledge on fiber-reinforced concrete and provide insights into the effectiveness of hybrid fiber reinforcement.
This type of concrete has numerous practical uses in the construction industry. The results will be valuable for engineers and researchers in the construction industry to design and optimize concrete structures with improved durability and enhanced resistance to cracking and deformation. This concrete type can be implemented in structures to repair structures with cracks, for ground flooring because of the water resistance of this concrete type.
This study can be enhanced further by researching the economic impact of this hybridization type. Also, to check the effect of this fiber type on the flexure and ductility of concrete beams.
Disclosure statement
No potential conflict of interest was reported by the author(s).
Additional information
Funding
Notes on contributors
Paschal Chimeremeze Chiadighikaobi
Paschal Chimeremeze Chiadighikaobi (Ph.D.) is a Nigerian. He acquired his Ph.D., M.Sc., and B.Eng and Tech. from Peoples Friendship University of Russia (RUDN University), Moscow, Russia. His research interests are in; strength and durability concrete, structural materials, composite materials, structural design and analysis, computational civil engineering, steel frame stability analysis, computational mechanics of structural systems, concrete reinforcement, nano-concrete, fiber dispersed concrete reinforcement, lightweight concrete, basalt fiber, finite element analysis, green city, design of structural timbers, machine learning in structural and construction engineering, artificial intelligence in structural and construction engineering, data mining in structural and construction engineering.
Andersen Rishammah Zakka
Andersen Rishammah Zakka is a Nigerian. He holds a Bachelor’s degree in Civil Engineering from Afe Babalola University, Ado-Ekiti, Nigeria.
Kebba Camara
Kebba Camara is from The Gambia. He is an Engineer at Gambian Port Authority. He obtained his Bachelor’s and Master’s degrees in Civil Engineering from Peoples Friendship University of Russia, Moscow Russia.
Vladimir Jean Paul
Vladimir Jean Paul (PhD) obtained his Bachelor’s degree, Master’s degree and Ph.D in Civil Engineering, from Peoples Friendship University of Russia. He is a lecturer in the department of civil engineering, RUDN University.
References
- Abbas Ashour Alaraza, H., Kharun, M., & Chiadighikaobi, P. C. (2022). The effect of minibars basalt fiber fraction on mechanical properties of high-performance concrete. Cogent Engineering, 9(1), 1–21. https://doi.org/10.1080/23311916.2022.2136603
- Ahmed, S. F. U., & Maalej, M. (2009). Tensile strain hardening behaviour of hybrid steel-polyethylene fibre reinforced cementitious composites. Construction and Building Materials, 23(1), 96–106. https://doi.org/10.1016/j.conbuildmat.2008.01.009
- Ahmed, S. F. U., & Mihashi, H. (2011). Strain hardening behavior of lightweight hybrid polyvinyl alcohol (PVA) fiber reinforced cement composites. Materials and Structures, 44(6), 1179–1191. https://doi.org/10.1617/s11527-010-9691-8
- Al-Kharabsheh, B. N., Arbili, M. M., Majdi, A., Alogla, S. M., Hakamy, A., Ahmad, J., & Deifalla, A. F. (2022). Basalt fibers reinforced concrete: Strength and failure modes. Materials, 15(20), 7350–7350. https://doi.org/10.3390/ma15207350
- Atahan, H. N., Pekmezci, B. Y., & Tuncel, E. Y. (2013). Behavior of PVA fiber-reinforced cementitious composites under static and impact flexural effects. Journal of Materials in Civil Engineering, 25(10), 1438–1445. https://doi.org/10.1061/(ASCE)MT.1943-5533.0000691
- Banthia, N., & Gupta, R. (2004). Hybrid fiber reinforced concrete (HFRC): Fiber synergy in high strength matrices. Materials and Structures, 37(10), 707–716. https://doi.org/10.1007/BF02480516
- Banthia, N., & Sappakittipakorn, M. (2007). Toughness enhancement in steel fiber reinforced concrete through fiber hybridization. Cement and Concrete Research, 37(9), 1366–1372. https://doi.org/10.1016/j.cemconres.2007.05.005
- Banthia, N., & Soleimani, S. M. (2005). Soleimani flexural response of hybrid fiber-reinforced cementitious composites. ACI Materials Journal, 102, 382–389.
- Bentur, A., & Mindess, S. (2006). Fibre reinforced cementitious composites. CRC Press.
- Bentur, S., & Mindess, A. (2022). Fibre reinforced cementitious composites. Routledge; CRC Press.
- British Standard Institution (1983a). BS 1881: Method for determination of slump. Part 102.
- British Standard Institution (1983b). BS 1881: Testing concrete. Method for determination of water absorption. Part 122. https://doi.org/10.3403/00049286
- BS EN 934-2:2009 + A1 (2012). Admixtures for concrete, mortar and grout concrete admixtures. Definitions, requirements, conformity, marking and labelling.
- Cemmix (n.d.). Retrieved from https://cemmix.ru/clauses/kak-sdelat-beton-prochnee-bazaltovaya-fibra-armiru
- Chiadighikaobi, P. C., Emiri, D. A., Abu Mahadi, M. I., Camara, K., Al-Shaibani, F., Haidar, M. M., & Saad, L. A. (2022). Performance evaluation of dispersed basalt fiber on strength of lightweight expanded clay concrete. Cogent Engineering, 9(1), 2137007. https://doi.org/10.1080/23311916.2022.2137007
- Concrete slump test (n.d.). Retrieved from https://academic-accelerator.com/encyclopedia/concrete-slump-test#google_vignette
- Ding, C., Xue, K., & Yi, G. (2023). Research on fire resistance and economy of basalt fiber insulation mortar. Scientific Reports, 13(1), 17288. https://doi.org/10.1038/s41598-023-44591-9
- EN 197-1 (2000). Cement – Part 1: Composition, specifications and conformity criteria for common cements (pp. 1–20).
- Fiber is basalt (n.d.). Microfiber. Retrieved from https://snab-pol.ctlx.ru/products/b354202553-fibra-bazaltovaya-mikrovolokno
- Halvaei, M., Jamshidi, M., & Latifi, M. (2014). Investigation on pullout behavior of different polymeric fibers from fine aggregates concrete. Journal of Industrial Textiles, 45(5), 995–1008. https://doi.org/10.1177/1528083714551437
- Halvaei, M., Jamshidi, M., Pakravan, H. R., & Latifi, M. (2015). Interfacial bonding of fine aggregate concrete to low modulus fibers. Construction and Building Materials, 95, 117–123. https://doi.org/10.1016/j.conbuildmat.2015.07.024
- Ivorra, S., Garcés, P., Catalá, G., Andión, L. G., & Zornoza, E. (2010). Effect of silica fume particle size on mechanical properties of short carbon fiber reinforced concrete. Materials and Design, 31(3), 1553–1558. https://doi.org/10.1016/j.matdes.2009.09.050
- Jamshidi, M., & Karimi, M. (2010). Characterization of polymeric fibers as reinforcements of cement-based composites. Journal of Applied Polymer Science, 115(5), 2779–2785. https://doi.org/10.1002/app.30302
- Khalil, W. I. (2013). Mechanical properties of high performance concrete fibre reinforced concrete. Engineering and Technical Journal, 31, 411–416.
- Khalil, W. I., & Abdulrazaq, A. (2011). Mechanical properties of high performance carbon fibre concrete. Engineering and Technical Journal, 29(5), 906–924.
- Korotkova, T. G., Ksandopulo, S. J., Donenko, A. P., Bushumov, S. A., & Danilchenko, A. S. (2016). Physical properties and chemical composition of the rice husk and dust. Oriental Journal of Chemistry, 32(6), 3213–3219. https://doi.org/10.13005/ojc/320644
- Laosombatthawee, P., Wiwattanadate, D., & Kotchawattana, S. (2015). Recovery of metals from electronic waste by reduction melting method. Applied Environmental Research, 37(1), 49–55. https://doi.org/10.35762/AER.2015.37.1.5
- Li, M., Shen, D., Yang, Q., Cao, X., Liu, C., & Kang, J. (2022). Rehabilitation of seismic-damaged reinforced concrete beam-column joints with different corrosion rates using basalt fiber-reinforced polymer sheets. Composite Structures, 289, 115397. https://doi.org/10.1016/j.compstruct.2022.115397
- Markovic, I. (2006). High-performance hybrid-fibre concrete–development and utilisation [PhD thesis]. Technische University.
- Mohankar, R. H., Pidurkar, M. D., Thakre, P. V., & Pakhare, S. S. (2016). Hybrid fibre reinforced concrete. International Journal of Scientific & Technology Research, 5(1), 1–4.
- Pakravan, H. R., & Ozbakkaloglu, T. (2019). Synthetic fibers for cementitious composites: A critical and in-depth review of recent advances. Construction and Building Materials, 207, 491–518. https://doi.org/10.1016/j.conbuildmat.2019.02.078
- Pakravan, H. R., Latifi, M., & Jamshidi, M. (2017). Hybrid short fiber reinforcement system in concrete: A review. Construction and Building Materials. 142, 280–294. https://doi.org/10.1016/j.conbuildmat.2017.03.059
- Park, J.-G., Seo, D.-J., & Heo, G.-H. (2022). Impact resistance and flexural performance properties of hybrid fiber-reinforced cement mortar containing steel and carbon fibers. Applied Sciences, 12(19), 9439. https://doi.org/10.3390/app12199439
- Qian, C. X., & Stroeven, P. (2000). Development of hybrid polypropylene-steel fibre reinforced concrete. Cement and Concrete Research, 30(1), 63–69. https://doi.org/10.1016/S0008-8846(99)00202-1
- Ralegaonkar, R., Gavali, H., Aswath, P., & Abolmaali, S. (2018). Application of chopped basalt fibers in reinforced mortar: A review Basalt based fiber-reinforced materials and structural applications in civil engineering. Construction and Building Materials, 164, 589–602. https://doi.org/10.1016/j.conbuildmat.2017.12.245
- Ramesh, B., & Eswari, S. (2021). Mechanical behaviour of basalt fibre reinforced concrete: An experimental study. Materials Today: Proceedings, 43, 2317–2322. https://doi.org/10.1016/j.matpr.2021.01.071
- Sharma, A., Reddy, G. R., Varshney, L., Bharathkumar, H., Vaze, K. K., Ghosh, A. K., Kushwaha, H. S., & Krishnamoorthy, T. S. (2009). Experimental investigations on mechanical and radiation shielding properties of hybrid lead–steel fiber reinforced concrete. Nuclear Engineering and Design. 239(7), 1180–1185. https://doi.org/10.1016/j.nucengdes.2009.02.017
- Shen, D., Li, M., Yang, Q., Wen, C., Liu, C., Kang, J., & Cao, X. (2022). Seismic performance of earthquake-damaged corroded reinforced concrete beam-column joints retrofitted with basalt fiber-reinforced polymer sheets. Structure and Infrastructure Engineering, 18, 1–17. https://doi.org/10.1080/15732479.2022.2147197
- Shi, J., Sun, S., Cao, X., & Wang, H. (2023). Pullout behaviors of basalt fiber-reinforced polymer bars with mechanical anchorages for concrete structures exposed to seawater. Construction and Building Materials, 373, 130866. https://doi.org/10.1016/j.conbuildmat.2023.130866
- Shu, X., Graham, R. K., Huang, B., & Burdette, E. G. (2015). Hybrid effects of carbon fibers on mechanical properties of Portland cement mortar. Materials and Design, 65, 1222–1228. https://doi.org/10.1016/j.matdes.2014.10.015
- Silva, E. R., Coelho, J. F. J., & Bordado, J. C. (2013). Strength improvement of mortar composites reinforced with newly hybrid-blended fibres: Influence of fibres geometry and morphology. Construction and Building Materials, 40, 473–480. https://doi.org/10.1016/j.conbuildmat.2012.11.017
- Song, W., & Yin, J. (2016). Hybrid effect evaluation of steel fiber and carbon fiber on the performance of the fiber reinforced concrete. Materials, 9(8), 704. https://doi.org/10.3390/ma9080704
- SulphiaBeevi, U., Joseph, A., & Nazeer, M. (2020). Effect of hybrid fibres on the mechanical properties of high-performance concrete. IOP Conference Series: Earth and Environmental Science, 491(1), 012035. https://doi.org/10.1088/1755-1315/491/1/012035
- Tassew, S. T., & Lubell, A. S. (2014). Mechanical properties of glass fiber reinforced ceramic concrete. Construction and Building Materials, 51, 215–224. https://doi.org/10.1016/j.conbuildmat.2013.10.046
- Vairagade, V. S., Kene, K. S., & Patil, T. R. (2012). Comparative study of steel fiber reinforced concrete over control concrete. International Journal of Scientific and Research Publications, 2(5), 1–3.
- Vandewalle, L. (2007). Postcracking behaviour of hybrid steel fiber reinforced concrete. In Proceeding of the 6th International Conference on Fracture Mechanics of Concrete and Concrete Structures (Vol. 3, pp. 1367–1375).
- Vandewalle, L. (2007, June 17–22). Postcracking behaviour of hybrid steel fiber reinforced concrete. In Proceedings of the 6th International Conference on Fracture Mechanics of Concrete and Concrete Structures.
- Yao, W., Li, J., & Wu, K. (2003). Mechanical properties of hybrid fiber-reinforced concrete at low fiber volume fraction. Cement and Concrete Research, 33(1), 27–30. https://doi.org/10.1016/S0008-8846(02)00913-4