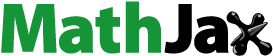
Abstract
Waste is considered a significant environmental issue in modern times thus the purpose of this paper is to compare the use of different waste materials in replacing aggregates in concrete. This study investigates the potential of incorporating plastic waste (PW), textile sludge (TS), and construction and demolition waste (CDW) into concrete as sustainable alternatives to traditional construction materials, by evaluating its mechanical, durability characteristics as well as the cost related to different process of the project. The study focuses on the use of PW and TS as a replacement for fine aggregate, and CDW as replacements for coarse aggregate. Thus, four concrete mixes incorporating PW and TS were prepared, using 0% 10%, 25%, 40% replacement levels. The compressive strength of concrete with 10% plastic waste as fine aggregate was 32.49 N/mm2 showing higher results than that of TS. Furthermore, another four concrete mixes incorporating CDW were prepared, using 0%, 25%, 50%, 75% replacement levels. The compressive strength of concrete with 25% CDW was 30.90 N/mm2 indicating an increase of 2% than the control block. The study concluded that the best strategy is to incorporate construction and demolition waste as a replacement for coarse aggregate in concrete. This is because it resulted in an increase in compressive strength as well as a decrease in cost.
This article is discussing the validation of the usage of various waste materials in place of aggregates in concrete, as waste is now considered as a major environmental issue. This study examines the viability of using construction and demolition waste (CDW), textile sludge (TS), and plastic waste (PW) in concrete as sustainable substitutes for conventional building materials, which will be a milestone in reaching the concept of sustainable housing. This will be reached by assessing the material's durability and mechanical properties as well as the associated costs of various project processes. The study focuses on replacing the coarse aggregate which is the dolomite with construction and demolition and replacing fine aggregate which is the sand in this case, with Plastic waste and Textile Sludge.
Public Interest Statement
Reviewing Editor:
1. Introduction
Concrete is considered the most used material in construction industry (Gagg, Citation2014). Concrete is composed of cement, aggregates, and water. The cement production is considered a great contributor to CO2 emissions. The expected annual usage of concrete is 11 billion metric tonnes because it is the most used construction material worldwide (Parveen et al., Citation2013). Aggregates are natural resources that are derived from natural deposits of gravel, sand, or rocks (Parmar, Citation2023). With the increased consumption of concrete, this natural resource will be depleted and scarce, thus requiring another environmentally friendly replacement. Applying the concept of green buildings to the concrete industry, different alternatives could be used to reduce cement’s content or natural aggregates’ content and replaced by more sustainable environmentally friendly constituents.
Industrial waste includes any waste generated by industrial activities, such as manufacturing, mining, construction, and agriculture. Manufacturing and construction in Egypt are associated with significant amounts of non-biodegradable solid waste (Labaran et al., Citation2022). The productive use of waste material represents a mean of alleviating some of the problems of solid waste management (Davis & Cornwell, Citation1998). Reusing of wastes is considered essential and important from multiple perspectives. It helps preserve and sustain non-renewable natural resources, decreases the pollution of the environment, and helps to conserve and recycle energy production processes. Wastes and industrial by-products should be viewed as potentially valuable assets awaiting appropriate treatment and application. This study investigates the potential of incorporating plastic waste (PW), textile sludge (TS), and construction and demolition waste (CDW) into concrete as sustainable alternatives to traditional construction materials, reducing the reliance on natural resources and minimizing waste sent to landfills.
In recent years, extensive research has focused on the properties and applications of geopolymer composites, which offer promising solutions for the construction industry. In a comprehensive review study by Ahmed Saeed et al. (Citation2022) the mechanical and microstructural properties of geopolymer composites were thoroughly investigated. In this comprehensive review study, the authors conducted an in-depth analysis of the mechanical and microstructural properties of geopolymer composites. By investigating the properties and behaviour of these composites, the study sheds light on their suitability as sustainable alternatives in the construction industry. Geopolymer concrete has been investigated as a feasible green construction material that can reduce CO2 emissions because it uses industrial wastes as raw materials.
The incorporation of industrial waste such as waste glass as aggregate in concrete has gained significant interest in recent years. In their review, Qaidi et al. (Citation2022) examine the mechanical characteristics of concrete containing waste glass. The findings reveal that incorporating waste glass as a partial replacement for natural aggregates can improve the workability properties of concrete. Moreover, the inclusion of waste glass in concrete can enhance the mechanical properties, such as compressive strength and flexural strength. This approach not only helps reduce the environmental impact of waste glass disposal but also contributes to the sustainable development of the construction industry. In this paper, A comparison is held to demonstrate the difference between incorporating other industrial wastes that demonstrate a significant environmental issue such as plastic waste (PW), textile sludge (TS), and construction and demolition waste (CDW). They are investigated as a potential replacement, in partial quantities, to natural aggregates. Using waste/recycled materials in concrete can be a reasonable option with apparent ecological and environmental advantages.
Plastic wastes disposal has harmful effects on the environment due to their long biodegradation period, and therefore one of the logical methods for reduction of their negative effects is the application of these materials in other industries. Plastic consumption has increased over the years as a result of its low price, flexibility, durability, and global industrialization. The slow rate of degradation and the large number of plastic wastes produced by human activities results in increased number of required landfills. Due to the toxic gases emitted, incineration is not a viable disposal method because it is harmful to the environment and human health. Recently, research have suggested reusing plastic wastes in concrete as natural aggregate replacement to reduce the exploitation of natural resources, and it could minimize the negative environmental impacts by construction (Tahir et al., Citation2022). Research in the field of plastic waste evaluated both the mechanical and durability properties of eco-friendly concrete incorporating electronic plastic waste (EPW) as partial replacement of fine aggregate. Natural fine aggregates were substituted with plastic fine aggregates (PFA) using 0%, 10%, 15%, and 20% ratios. The detrimental effect of PFA on the mechanical properties limits the application of EPW concrete in projects. However, its increased durability extends its usage in other applications such as coastal structures (Ullah et al., Citation2022). Another study was conducted to assess the possibility of utilizing recycled waste plastic, as a replacement of natural aggregates in concrete. Concrete samples were prepared utilizing three different types of recycled plastics (Granules, flakes, fibres) at varying proportions; 25%, 50%, 75%, 100% and they were evaluated to assess their mechanical and thermal properties. The highest compressive strength of 35 MPa was noted in concrete with 25% granule type RPA. This strength is about 25% less than that of the normal concrete. 25% was the only RPA percentage to satisfy the strength requirement criteria (Basha et al., Citation2020). Recycled Polyethylene Terephthalate (PET) was also studied in a concrete mix of variable water/cement ratio (0.50 and 0.60), PET content (10 and 20 vol%) and the particle size. Using PET implies defects in the internal structure of the concrete, reducing the strength and stiffness. This behaviour can be profitable when some ductility of the material is required, specifically at PET contents of 10% (Albano et al., Citation2009). Furthermore, Plastic waste is inexpensive because it is plentiful abundant in supply. Therefore, reusing plastic wastes as a substitution for natural aggregates is feasible because it is economic and environmentally friendly. Production of plastic has risen significantly in the last 60 years worldwide, and around 10% of this plastic is turned into solid waste. In the absence of appropriate recovery methods, this plastic becomes an environmental hazard. By incorporating plastic waste into concrete, the exploitation of natural resources can be reduced, while also mitigating the negative environmental impacts of construction activities.
Lately, great attention is directed to sludge treatment in Egypt to reduce its serious environmental and public health impact. In the past years, usually sludge management was focused on its disposal or discharging without any adequate treatment (Ghazy et al., Citation2009). Attempts have been made to incorporate TS sludge in the building materials. Sludge was stored, dried until the moisture content obtained was 36%. The experimental results indicated that it is not possible to reuse the textile effluent sludge as partial replacement for producing non-load bearing concrete blocks (Loganayagan et al., Citation2020). The results of this investigation showed that performance of concrete containing dry sludge depends on sludge composition, organic material content and volume of incorporation. Thus, different water to cement ratio (0.45) and sludge contents up to 20% were used to report the different effect of these parameters to the output (Muniraj et al., Citation2014). As well as, investigating partial replacement up to 30% for fine aggregate (Sakthivel et al., Citation2019). Further studies were conducted to report the effect of using dry sludge on compressive strength with the pre-treatment of TS, a lime-based pre-treatment process to remove ammonia showed better results at the TS replacement level yet consuming more energy ion the treatment process (Zhan & Poon, Citation2015). Meanwhile, other studies replaced cement with powdered textile sludge, with a maximum of 10% substitution. It was found that replacing the cement with sludge is not a proper option as it resulted in significant decrement in compressive strength of concrete as 5% replacement level caused a decrease in permeability of the mixture (Goyal et al., Citation2019). Therefore, the use of Textile sludge in construction materials could serve as an alternative solution to disposal and reduce pollution as Textile industry is considered the world’s second largest industry. 10% of the world’s productive energies are used in this activity and a huge part of the global population earns it living by working in the industry (Hall et al., Citation1972). It is growing at an average rate of 6.5% annually and have a great importance to Egyptian economy. It employs more than half a million Egyptians (Ibrahim et al., Citation2008). The textile dyeing industry demands large quantities of water, and results in large amounts of wastewater streams of complex contaminant matrix from the different steps of dyeing process (Sójka-Ledakowicz et al., Citation1998). The textile industry’s economic importance and employment opportunities further highlight the potential of incorporating textile sludge in sustainable construction practices.
Construction and Demolishing waste (CDW) waste consists of the residual materials that remain after the construction finishing or the demolition of a building. Construction and demolition debris are the materials generated from the modifications, construction, demolishing, renovation, or repair of any manmade building structures including houses, administration buildings, industrial or commercial facilities, and roads (Agency, XXXX). Over 85% of construction waste are ineffectual and are known as public fill. Public fill includes debris, rubble, soil, and concrete which is suitable for land reclamation and site formation. In Egypt, the estimated daily quantity of construction and demolition waste (CDW) is 10,000 tons (Al-Ansary, Citation2004). With the increase in construction due to development of countries as well as the over population, this problem became a major issue affecting the environment and the economy of countries globally. Instead of disposal of the construction waste, a waste management plan can reduce the negative environmental impact and improve the economy. Over 85% of construction and demolition waste are ineffectual and are known as public fill. In previous studies building rubbles were collected from demolished structures. It included used concrete, tiles, brickwork, steel, wood, plastic, and paper, among other materials. After appropriate treatment, only waste concrete, bricks, tiles, and a small number of impurities remained in the building materials (Chen et al., Citation2003). A study of using recycled concrete aggregates from precast block to produce new building blocks were also conducted. The concrete blocks produced with 30% and 100% of RCA met the requirements for strength, capillary water absorption, drying shrinkage, and freeze-thaw resistance (Zhao et al., Citation2020). The minimizing of construction and demolishing waste will lead to saving money, reducing negative environmental impacts, conserving virgin materials and reducing the wasted landfill spaces. The construction and demolishing waste minimization began by reducing, reusing, recycling, recovering the materials and finished by disposal to land fill sites.
There are several reasons why plastic waste, textile sludge, and construction & demolition waste are being incorporated in concrete. One main benefit is the environmental benefit of reducing the amount of waste that can be sent to landfills, and by turn reduces environmental pollution. This benefit also helps conserve natural resources such as sand and gravel that is soon to demolish. Additionally, these different types of waste are one of the top sources of wastes that can be incorporated in concrete with no processing and minimal pre-treating. Furthermore, this method has a lower energy consumption compared to other waste disposal methods. As well as being the cheapest and easiest way to dispose them. As a result, plastic waste, textile sludge, and construction and demolition waste are among the top waste materials being used to create sustainable concrete solutions.
2. Research significance
Different researchers incorporated one type of waste replacing portions of aggregates whether fine or coarse. However, to the researcher knowledge, no research has investigated incorporating different types of waste and compare their properties. Therefore, in this paper, plastic waste, textile sludge, and construction and demolition waste are added to the concrete mix to investigate their behaviour and to be a step towards greener buildings by reducing amount consumed of natural aggregates and by using efficiently wastes. We included assessing the potential impact on concrete strength and durability by conducting experimental tests, such as compression tests and fire resistance tests. This research started with incorporating different percentages depending on the usage of fine aggregate and coarse aggregates.
The research also examines the processing methods required for each waste material, such as the melting and crushing of plastic waste and the dewatering and pre-treatment of textile sludge.
Furthermore, the research adds value by conducting a comprehensive cost analysis of incorporating waste materials into concrete. While many studies have explored the technical feasibility of using waste materials in concrete production, the cost implications are often overlooked. By conducting a detailed cost analysis, the research provides insights into the financial feasibility of incorporating plastic waste, textile sludge, and construction and demolition waste into concrete mixes. The cost analysis considers various components, including sourcing costs, transport costs, and processing costs. It considers the expenses associated with purchasing and collecting waste materials, as well as the transportation costs involved in bringing the waste materials to the manufacturing facility.
Additionally, the research considers the environmental costs and social costs associated with incorporating waste materials into concrete. It acknowledges the reduction in environmental costs related to waste disposal and the use of virgin materials. However, it also highlights the energy consumption and emissions associated with the production and processing of waste materials. The social costs are assessed in terms of potential negative impacts on workers’ health and safety during the collection and processing of waste materials.
By including a comprehensive cost analysis that encompasses financial costs, environmental costs, and social costs, the research contributes to a more holistic understanding of the feasibility and implications of using waste materials in concrete production. This information is valuable for decision-makers in the construction industry who need to assess the economic viability and sustainability of adopting these alternative materials.
3. Methodology
3.1. Materials
Ordinary Portland cement (OPC), natural coarse aggregate (Dolomite), natural fine aggregate (sand), and different types of waste materials; recycled plastic (RP), textile sludge (TS), and construction& demolition waste (CDW). These materials were used in the preparation of the concrete specimens. The Portland cement used is Assiut Portland pozzolanic cement, an Eco-friendly product which reduces CO2 emissions by 15 to 20%. It is produced in Assiut Cement Company – CEMEX. The chemical and physical characteristics of cement are shown in and , respectively. The recycled plastic was collected from Cairo’s garbage city, Egypt. Its processing starts by the collection of waste plastic from all over Cairo, separation based on their nature followed by melting to form large solid blocks, which are again crushed to small pieces (5–10 mm) by using varying-sized cutting blades. They are produced in the shape of granules. The textile sludge used in the study was the coagulant sludge withdrawn from a bleaching and dyeing factory located in Giza, Egypt. The sludge collected was generated from the wastewater treatment process of the factory. The rubbles were transferred directly from a construction site without any separation.
Table 1. Chemical characteristics of cement.
Table 2. Physical and mechanical characteristics of cement.
3.2. Experimental tests
The effect of partial substitution of aggregates with waste was evaluated by measuring the mechanical properties, such as compressive strength, water absorption, and fire resistance. To measure the above properties, three laboratory tests were carried out on the concrete specimens to provide comparison points between the different materials incorporated in concrete. The experiments conducted in this study strictly adhere to the guidelines and regulations set forth by the Egyptian Building Code (EBC). The EBC serves as the fundamental reference for the design, construction, and safety of buildings in Egypt. The compression strength test and water absorption test are both performed on the three waste materials as shown in . As, they are important indicators of the quality, durability, and strength of the concrete, and are also used to determine the suitability of the concrete for construction purposes. Meanwhile, Fire resistance is performed only on plastic waste as it can release flammable gases when exposed to high temperatures, which can increase the risk of fire (Siddique et al., Citation2008). It’s important to evaluate the fire resistance of PW concrete to ensure its safety in real-world applications.
Compression strength test: the test was performed by compressing the cubes by an axial load till failure as shown in . Take the result of the failure load (P) then dividing the load by the cube surface area (A) to find the compressive strength (σ) in N/mm2.
Water absorption test: The test was performed by putting the cube samples into an oven with 105 °C for 24 hours then weight the sample (W1) as shown in . The next step was to immerse the sample in water for another 24 hours to absorb water then weight the sample again (W2). The water absorption ratio (WA) was calculated by dividing the difference between the two weights (W1-W2) by the weight of the original dried sample (W2) times 100%.
Fire resistance test: the test was performed after firing the samples at 3000 for 3 hours, by compressing the cubes by an axial load till failure as shown in . Take the result of the failure load (P) then dividing the load by the cube surface area (A) to find the compressive strength (σ) in N/mm2.
Figure 2. Procedures of compression test in the laboratory.

4. Experimental mix proportions
4.1. Fine aggregate replacement
Four concrete mixtures with varying proportions of waste (0, 10, 25 and 40%), cement content of (400 kg/m3), Coarse aggregate of (1320 Kg/m3) and (160 L) of water per one cubic meter of concrete. A dry mixture was prepared by using water to cement ratio of (0.4). Sand was replaced with plastic waste/textile sludge varying its weight in every mixture, as shown in . The samples are 100*100*100 mm cubes. Each sample of control concrete cubes contains 0.4 kg of normal Portland cement, 0.66 kg sand, 1.32 kg coarse aggregate and 0.2 Liter of water. All the samples were tested after 28 days of preparing at the faculty of engineering, Cairo University, materials tests laboratory. The types of concrete samples are prepared as follows:
Table 3. Mix proportion of concrete incorporating Plastic waste or textile sludge.
4.2. Coarse aggregate replacement
Sludge and plastic granules are a fine-grained material, while construction and demolition waste are a larger, coarse-grained material. The specific properties of the materials, such as particle size, shape, and texture, affected its suitability to be used as fine aggregate. Furthermore, the use of construction waste as a coarse aggregate replacement has been shown to improve the workability and reduce the water demand of concrete mixtures. 4 types of concrete samples are prepared with 0, 25, 50, 75% mixed waste respectively.
The samples are 10*10*10 cm cubes while the aggregate and the mixed waste (Concrete + Bricks with the same ratio) are with 15 mm maximum nominal size. The concrete mixture consists the same concrete mix of 400 kg cement, 0.66 kg sand, 1.32 kg coarse aggregate and 0.2 liter of water per one cubic meter of concrete as shown in . All the samples were tested after 7 days of preparing at the faculty of engineering, Cairo University, materials tests laboratory.
Table 4. Mix proportion of concrete incorporating construction and demolition waste.
The concrete mixtures were prepared in a long process indicated in . By mixing the dry materials, fine aggregate, coarse aggregates, and cement in a concrete mixer for about 2 mins then waste was added. The ingredients were fully mixed again for another 2–3 min. The water was divided in two halves. The first half was added to the ingredients in the mixer, and they were mixed for 3 mins and then the remaining water was added in the process of mixing. All the ingredients were then mixed for additional 2–3 min. The concrete was then poured in the molds and compacted using compaction rod. The molds were placed on a vibrator for consolidation. The specimens were labelled with the plastic percentage, cured by leaving them for 24 h under laboratory conditions followed by curing under wet conditions as shown in .
5. Experimental findings and discussion
5.1. Compressive strength
Concrete cube specimens were prepared to determine the compressive strength. A 3000 KN capacity digital compression testing machine was used to apply an axial compressive load on the specimen until failure. The maximum compressive load was divided by the cross-sectional area of the specimen to calculate the compressive strength. To give a quantitative measure of the compression S test result of the specimens, the compressive strength (σ) is defined as:
(1)
(1)
where σ is 28-day compressive strength of cement bricks prepared with waste material and the control block (N/mm2), while P is the Crushing Load in kilo newton (KN), and A is the specimens Area in millimetres square (mm2).
5.1.1. Fine aggregates
Based on previous studies conducted on textile sludge, they observed 80% reduction at the replacement level of 10% when pre-treated by over drying and grinding. Meanwhile, when using lime treatment and leaving the mixture to age for 24 h in the laboratory environment, 17% reduction was obtained when incorporating the same replacement level (Zhan & Poon, Citation2015). Another study obtained with water/cement ratio of 0.432, showed a 1% increase of 5% replacement. Yet, it showed a 10% reduction of 15% replacement (Muniraj et al., Citation2014). As shown in , the test results from 28 days compressive strength of 0% replacement of textile sludge was 32.49 N/mm2. A decrease in the compressive strength was noted with an increase in the quantity of TS. The highest compressive strength of was 26.811 N/mm2 noted in concrete with 10% TS. This strength is 17.5% less than that of the normal concrete. Meanwhile, there was a great reduction in compressive strength of concrete with other percentages. Mixes of 25%, and 40% was 19.1 and 16.6 N/mm2, which shows a reduction of 41, and 49% respectively.
Table 5. Compression test results.
Whereas previous studies conducted on plastic waste, showed that the variations of compressive strength values are due to the differences in the type of plastic wastes used and the differences in the workability behaviour of concrete mix. As upon using they observed a 72% reduction at the replacement level of 20% (Saikia & De Brito, Citation2012). And when E-waste was used, the compressive strength was reduced by 52.98% when coarse aggregate is replaced by 20% (Manjunath, Citation2016). Considering the cement content to be 400 kg/m3 with 160 L of water, and mixture prepared with plastic waste substitution, a decrease in the compressive strength was noted with an increase in the quantity of plastic. The reduction in strength may be attributed to the low strength of plastic compared to that of the natural coarse aggregates. The highest compressive strength of 26.27 N/mm2 was noted in concrete with 10% plastic. This strength is about 19.1% less than that of the normal concrete. Nevertheless, the compressive strength of concrete with 25% and 40% plastic was 25.34 and 23.97 N/mm2, respectively, which satisfied the strength requirement criteria of 20 MPa for medium strength concrete. provides an insightful visual comparison between the two waste materials under consideration.
5.1.2. Coarse aggregates
The results are indicating an increase in compressive strength (σ) with 2% for sample (2), a reduction in compressive strength of 17.5% for sample (3) and 17.8% for sample (4) in reference to sample (1) (Control Sample), as shown in . The increase in compressive strength for sample 2%, which incorporate 25% waste, can be attributed to the irregular shapes and sizes of construction and demolition waste particles filling the voids between larger aggregates, reducing the overall porosity of the concrete. This reduced porosity can improve the density and overall strength of the concrete.
5.2. Water absorption test
The specimens were weighed before being submerged in water. The samples were weighed after one day. The difference between the two weights was divided by the dried sample gives the result of water absorption ratio. To give a quantitative measure of the water absorption ratio test result of the specimens, the water absorption (WA) is defined as:
(2)
(2)
Where, WA is 28-day water absorption percentage of cement bricks prepared with waste material and the control block (N/mm2), while W2 is the dried sample weight in gram (gm), and W1 is the wet sample weight in gram (gm).
5.2.1. Fine aggregates
Based on previous studies conducted on textile sludge, Partial replacement of fine aggregate had a significant increase in 14-day water absorption ratio of the specimens. All the samples were beyond the maximum allowable values of 0.060%. The value even reached 0.1142% when 30% of fine aggregate was replaced (Zhan & Poon, Citation2015). The degree of porosity and absorption percentage increased with the increase of TS percentage. This may lead to decrease in the strength of the concrete (Maierdan et al., Citation2020). An increase in the absorption water ratio was noted with an increase in the quantity of Textile sludge. It can be seen in and illustrated in that the absorption ratio increase is 4.70%, 8.1%, and 10.90% for P10, P25 and P40, of TS concrete respectively.
Figure 9. Water absorption ratio comparison between PW and TS concrete compressive strengths.

Table 6. Water absorption test results.
An increase in the absorption water ratio was also noted with an increase in the quantity of plastic. It can be seen in that the results are about 4.63%, 5.09%, 5.18%, and 5.72% for P0, P10, P25, and P40 of PW concrete respectively. According to Albano et al. (Citation2009), their experimental work showed that water absorption percentage for 10% PW is lower than the ones with 20% due to the lower porosity.
5.2.2. Coarse aggregates
For the water absorption (WA), the results are indicating that the water absorption percentage is lower for samples (2), (3) by 15.3% and 2.9% for sample (4) in reference to sample (1) (Control Sample), as shown in . The decrease in sample 2 and 3 can be attributed to the inclusion of construction and demolition waste in the concrete mix helping to fill the gaps and voids between larger aggregates, resulting in a denser concrete matrix. A denser matrix with reduced porosity limits the penetration and absorption of water, leading to lower water absorption percentages. Meanwhile the regain of higher percentage in sample 4 identify that the Construction and demolition waste can have a diverse composition, and different waste materials may exhibit varying water absorption characteristics. It is possible that the specific construction and demolition waste material used in sample 4 had higher porosity or water absorption tendencies, leading to the observed increase.
5.3. Fire resistance test
The fire resistance test was performed on the same concrete cube specimens measuring 100 mm × 100 mm × 100 mm, three for each plastic percentage. The specimens were fired by 300º for 3 hours then a 3000 KN capacity digital compression testing machine was used to apply an axial compressive load on the specimen until failure. The maximum compressive load was divided by the cross-sectional area of the specimen to calculate the compressive strength. The compressive strength (σ) is defined as:
(3)
(3)
Ullah et al. (Citation2022) observed 37.27–44.4% reduction in compressive strength was experienced for EPW concrete containing 0%–20% at 300 ◦C for 1 hour. In our experimental work, a decrease in the compressive strength was greatly noted with an increase in the quantity of plastic, as shown in . The reduction in strength may be attributed to the melting of plastic inside the specimens. The highest compressive strength of 24.43 N/mm2 was noted in concrete with 10% plastic. This strength is about 16% less than that of the normal concrete, which satisfied the strength requirement criteria of 20 MPa for medium strength concrete. The compressive strength of concrete with 25% and 40% plastic was 20.42 and 17.7 N/mm2 less than that of control specimens with 29.6% and 39% respectively, which can be classified as a low strength concrete. Yet, the PW concrete is prone to strength degradation upon exposure to high temperatures.
6. Cost analysis
Preforming a cost analysis is crucial when comparing the use of different waste materials in replacing aggregates in concrete, such as textile sludge, plastic waste, and construction and demolition waste. The usage of waste materials can have a positive effect on environmental aspects, lowering the carbon footprint and reducing the quantity of waste ending up in landfills. Yet, utilizing these waste materials can have cost implications, that need to be carefully investigated (Tam, Citation2008). A cost analysis helps to calculate the cost related to different process of the project such as, the costs of sourcing, processing, transporting, and storing materials. In addition to the traditional costs, there are costs associated with the long-term benefits if using these improved materials (Singh et al., Citation2017).
True cost accounting is a concept that include the full costs of goods and services, such as financial costs as well as social and environmental costs that are not often investigated in traditional costs analysis. These costs can be broken down into several key components such as; sourcing costs, performance costs and the cost of any decrease in concrete strength and durability, environmental costs, and social costs (Gemmill-Herren et al., Citation2021).
6.1. Sourcing costs
This is considered the direct and most important component of our cost analysis, as it refers to cost related to purchasing, collecting, transporting, and processing &treating waste material to be incorporated in concrete.
6.1.1. Purchasing and collecting materials
This component describes the cost of both purchasing raw materials, such as cement, sand, and coarse aggregate as well as the collection of waste materials from their source. Collecting of materials depend mainly on the type and amount of waste materials. Materials are assessed according to the price rate (USD/m3) in correspondence to the amount of waste percentage, as shown in and .
Table 7. PW & TS concrete cost analysis.
Table 8. CDW concrete cost analysis.
6.1.2. Transporting materials
This component describes the cost of transportation of waste materials from their origin to the manufacturing facility. It depends on the distance travelled while carrying the waste materials, so it’s an important factor if the waste source was within the city’s boundaries. Transportation costs also depends on the volume and weight of the material being transported. The recycled plastic was collected from Cairo’s garbage city, Egypt. The textile sludge was withdrawn from a bleaching and dyeing factory located in Giza, Egypt. The rubbles were transferred directly from a construction site without any separation. The three sites are inside the city boundaries which decreases the transporting costs to minimum.
6.1.3. Processing materials
Plastic processing starts by the collection of waste plastic from all over Cairo, separation based on their nature followed by melting to form large solid blocks, which are again crushed to small pieces (5–10 mm) by using varying-sized cutting blades. They are produced in the shape of granules. On the other hand, sludge was dewatered to obtain a concentration of suspended particles in sludge of at least 20 percent by filtration through a specialized filter. The following step involved collecting the suspended soils in a mixture from the filter, spreading them out, and exposing them to air and direct sunlight for at least 14 days until they were air-dried. Using a pestle and mortar, the dried sludge was pulverized. Following the extraction of the powder, it was sieved to remove impurities and sand particles of significant size. As sludge has a comparatively high loss on ignition (L.O.I.) value, the final stage of the sludge preparation procedure involved the removal of the organic content. This was accomplished by burning the pulverized sludge dust at 350 C0 for one hour, which resulted in a 25% reduction in sludge weight, which is considered acceptable. Meanwhile, the rubbles were collected with no separation nor treatment, thus there is no cost of processing.
6.2. Performance costs
The cost of any potential impact on the performance of the concrete, such as decreased durability or strength. These costs are evaluated based on the experimental tests conducted such as, compression test and fire resistance test. One of the primal costs is the potential decrease in strength as reduction in strength can compromise the safety and durability of the structure. Incorporating 10% plastic resulted in 19.1% less strength than that of the normal concrete. Also, it was noted in concrete with 10% TS that the strength is 17.5% less than that of the normal concrete. Both waste materials incorporation in concrete still satisfies the strength requirement criteria of 30 MPa for high strength concrete. Meanwhile, replacing coarse aggregate with construction and demolition waste had a lower impact on the strength of concrete where a minor reduction in compressive strength 17.8% for 75% C&D waste and 2% increase for 20%.
6.3. Environmental costs
Incorporating the waste material used such as plastic waste, textile sludge, and construction & demolition waste into concrete reduces the environmental costs associated with waste disposal and waste management. We also reduce the amount of virgin materials needed for manufacturing such as sand and gravel. Yet, the environmental costs also include the production of the waste materials, the cost of emissions and other pollutants associated with their production as well as the energy used in processing of materials (Sandanayake, Citation2022). Our goal was to use materials with the least processing procedures to reduce energy. Construction and demolition waste produces no energy in collection as its not separated nor treated. As for plastic waste, the energy used is only produced in the shredding machine to produce the small particles that can be used. Meanwhile, textile sludge is the highest among the three materials to consume energy and emit greenhouse gases, as the sludge is dewatered, filtered, and pretreated before being used.
6.4. Social costs
Social costs are associated with any negative impact that can result from our product on local communities and the cost of mitigating those impacts. Construction and demolition waste have minimal social costs associated only with the collection of materials such as concrete, bricks, and wood. Furthermore, plastic waste has higher social costs due to its additional processing required after collection. This process includes sorting, cleaning, and shredding into small pieces before it can be incorporated into concrete. This additional process increases the social costs of potentially exposing workers to harmful activities. Meanwhile, textile sludge has much higher social cost due to its impact on the health and safety of field workers. Textile sludge is processed, dewatered, and pre-treated before being incorporated. This pretreatment process exposes workers to harmful chemicals and pollutants. It’s important to consider the social costs associated with these practices.
7. Conclusion
The usage of waste materials such as construction& demolition waste as a coarse aggregate substitution and plastic waste as well as textile waste as fine aggregate substitution in concrete has the potential to offer environmental benefits. However, careful consideration must be given to the strength, water absorption, fire resistance, and overall costs associated with each type of waste material.
Based on the test results, it can be concluded that
The best option is to incorporate construction and demolition waste as a replacement for coarse aggregate in concrete. This is because it resulted in an increase in compressive strength in some samples and a decrease in cost.
However, when it comes to fine aggregates, plastic waste is a better option than textile waste due to its higher compressive strength and lower water absorption. The recommended percentage of plastic waste is 10% to ensure the compressive strength of the concrete satisfies the criteria for medium-strength concrete.
It is important to note that while incorporating waste materials into concrete can offer environmental benefits, the social costs associated with these practices must also be considered. Proper waste management practices and the use of environmentally friendly processing methods can help minimize negative impacts and ensure that these practices are sustainable in the long run.
The use of construction and demolition waste as a replacement for coarse aggregate in concrete and the incorporation of 10% plastic waste as fine aggregate in concrete are recommended options based on the test results and cost analysis. However, further research is needed to investigate the long-term durability and environmental impacts of these practices.
Based on the cost analysis, it can be concluded that
Purchasing and Collecting Materials cost: Plastic Waste (PW) and Textile Sludge (TS) costs increase with higher waste percentages with TS showing slightly lower costs. However, Collection costs for Construction and Demolition Waste (CDW) decrease with higher waste percentages, as it is directly transferred without separation, indicating much more cost savings.
Transporting Materials: Waste materials collected within Cairo’s city boundaries minimize transporting costs as it reduces the distance travelled. Localized transportation aligns with sustainable practices, enhancing cost efficiency and reducing environmental impact.
Processing Materials: Processing methods vary, with each contributing to the overall cost of incorporating waste materials into concrete. Textile sludge processing includes dewatering, filtration, air-drying, pulverizing, sieving, and burning to remove organic content, which contributes to its overall cost. Meanwhile, plastic processing involves collection, separation, melting, and crushing, decreasing its cost that (TS). Remarkably, construction and demolition waste (CDW) stand out as the most efficient in this category. CDW requires no processing, as it is collected without prior separation or treatment.
Performance Costs: Despite strength reductions in concrete incorporating waste materials, all compositions meet high-strength concrete criteria. Incorporating 10% plastic waste (PW) resulted in a 19.1% reduction in strength compared to normal concrete. Concrete with 10% textile sludge (TS) showed a 17.5% strength reduction compared to normal concrete. Both waste materials still met the strength requirement criteria of 30 MPa for high-strength concrete. Replacing coarse aggregate with construction and demolition waste (CDW) had a lower impact on strength, with a 17.8% reduction for 75% CDW and a 2% increase for 20%. This strength analysis underscores the resilience of concrete incorporating diverse waste materials. While reductions in strength are evident, all compositions align with the high-strength concrete standards, affirming the feasibility and effectiveness of integrating waste materials into concrete structures.
Environmental Costs: Incorporating waste materials into concrete reduces environmental costs associated with disposal and waste management. Construction and demolition waste, being untreated, has minimal energy consumption during collection. Meanwhile, plastic waste requires energy primarily in the shredding process, and textile sludge exhibits the highest energy consumption due to dewatering, filtering, and pretreatment.
Social Costs: Construction and demolition waste has minimal social costs related to material collection. Plastic waste incurs higher social costs due to additional processing steps, potentially exposing workers to harmful activities. However, Textile sludge has the highest social costs, impacting the health and safety of field workers through its dewatering, filtering, and pretreatment processes. Considering social costs is crucial for evaluating the overall impact on local communities and ensuring responsible waste utilization practices.
The comprehensive cost analysis provides valuable insights for decision-making, considering both economic and sustainability factors.
7.1. Future scope
Optimization of Waste Material Ratios: Further investigation is needed to determine the optimal percentage of construction and demolition waste as a replacement for coarse aggregate in concrete. The study established positive outcomes in terms of compressive strength and cost reduction, but exploring different ratios may yield even better results.
Performance Enhancement: Future studies can focus on enhancing the strength and durability properties of concrete incorporating waste materials. This could involve the addition of supplementary cementitious materials or chemical admixtures to improve the overall performance of the concrete.
Evaluation of Long-term Durability: Long-term durability assessments are essential to ascertain the performance of concrete containing waste materials. Research should focus on evaluating the durability aspects such as resistance to chemical attack, freeze-thaw cycles, and carbonation over an extended period.
Environmental Impact Assessment: Conducting a comprehensive life cycle assessment (LCA) of concrete with waste material substitutions would provide a holistic understanding of its environmental impact. This analysis should consider factors such as greenhouse gas emissions, energy consumption, and waste generation during the entire life cycle of the concrete.
Standardization and Guidelines: Development of standardized guidelines and specifications for incorporating waste materials in concrete construction would facilitate their widespread adoption. These guidelines should encompass aspects such as material selection, processing methods, quality control, and structural design considerations.
Social and Economic Considerations: Future research should address the social and economic implications of using waste materials in concrete production. This includes evaluating the social acceptance of such practices, assessing the economic viability at a larger scale, and considering potential job creation and local industry development opportunities.
Data availability statement
Data available on request.
Disclosure statement
No potential conflict of interest was reported by the author(s).
Additional information
Funding
Notes on contributors

Hagar Hammad
Hagar Hammad, Architect and research assistant, Smart engineering systems Research centre (SESC), Nile University, Cairo, Egypt. In 2021 earned the BSC degree in Architecture Engineering, Ainshams University, Egypt.
Yasmin Elhakim
Yasmin Elhakim, Research Assistant, SESC, Nile University, PhD student at the AUC. involved in multiple research topics in the construction industry. won the Mohamed Bin Abdulkarim A. Allehedan Award in 2020 for demonstrating excellence in sciences and engineering studies from the AUC.
Tawfik Ismail
Tawfik Ismail, Associate Professor at NILES, Cairo University, Egypt. manages several types of research in implementing optical transceivers for FSO and PON.
Irene S. Fahim
Irene S. Fahim, the Director for Smart engineering systems Research centre, Nile University, Associate Professor, Industrial Engineering and Management department, Nile University Cairo, Egypt. the Director for SESC, Nile University. won the state encouragement award for women 2020, Hazem Ezzat Research excellence award, 2021 and Loreal UNESCO for Women in Science 2021 Egypt young talents program.
Mohamed Mahmoud
Mohamed Mahmoud, Assistant professor at the Architecture and Urban Design Program, School of Engineering and Applied Sciences, Nile University. 31 years of in-depth experience in Engineering firms, project Management and General management experience in consultant engineering offices and contracting companies. finished the BSc degree. in Civil Engineering, Cairo university, Egypt in 1991, then earned the master's and PhD. in Architectural Engineering, Cairo university, Cairo, Egypt.
References
- Agency, O. E. P. “Ohio Environmental Protection Agency.” www.epa.state.oh.us
- Al-Ansary, M. Sustainable guidelines for managing demolition waste in Egypt. Forum Barcelona International ‘RILEM’. (2004).
- Albano, C., Camacho, N., Hernández, M., Matheus, A., & Gutiérrez, A. (2009). Influence of content and particle size of waste pet bottles on concrete behavior at different w/c ratios. Waste Management (New York, N.Y.), 29(10), 2707–2716. https://doi.org/10.1016/j.wasman.2009.05.007
- Basha, S. I., Ali, M. R., Al-Dulaijan, S. U., & Maslehuddin, M. (2020). Mechanical and thermal properties of lightweight recycled plastic aggregate concrete. Journal of Building Engineering, 32, 101710. https://doi.org/10.1016/j.jobe.2020.101710
- Chen, H. J., Yen, T., & Chen, K. H. (2003). The use of building rubbles in concrete and mortar. Journal of the Chinese Institute of Engineers, Transactions of the Chinese Institute of Engineers, Series A/Chung-Kuo Kung Ch’eng Hsuch K’an, 26(2), 227–236. https://doi.org/10.1080/02533839.2003.9670773
- Davis, M. L., & Cornwell, D. A. (1998). “Introduction to environmental engineering.”
- Gagg, C. R. (2014). Cement and concrete as an engineering material: An historic appraisal and case study analysis. Engineering Failure Analysis, 40, 114–140. https://doi.org/10.1016/j.engfailanal.2014.02.004
- Gemmill-Herren, B., Baker, L. E., & Daniels, P. A. (2021). True cost accounting for food: Balancing the scale. Taylor & Francis.
- Ghazy, M., Dockhorn, T., & Dichtl, N. (2009). Sewage sludge management in Egypt: Current status and perspectives towards a sustainable agricultural use. World Academy of Science English and Technology, 33(9), 492–500.
- Goyal, S., Siddique, R., Jha, S., & Sharma, D. (2019). Utilization of textile sludge in cement mortar and paste. Construction and Building Materials, 214, 169–177. https://doi.org/10.1016/j.conbuildmat.2019.04.023
- Hall, P., Cliffs, E., & J, N. (1972). Encyclopedia of textiles.
- Ibrahim, N. A., Abdel Moneim, N. M., Abdel Halim, E. S., & Hosni, M. M. (2008). Pollution prevention of cotton-cone reactive dyeing. Journal of Cleaner Production, 16(12), 1321–1326. https://doi.org/10.1016/j.jclepro.2007.07.002
- Labaran, Y. H., Mathur, V. S., Muhammad, S. U., & Musa, A. A. (2022). Carbon footprint management: A review of construction industry. Cleaner Engineering and Technology, 9(July), 100531. https://doi.org/10.1016/j.clet.2022.100531
- Loganayagan, S., Rajkumar, G., Pavthra, A., & Poonkundran, M. (2020). Experimental study on concrete by partial replacement of fine aggregate by textile effluent treatment plant sludge. IOP Conference Series: Materials Science and Engineering, 764(1), 012043. https://doi.org/10.1088/1757-899X/764/1/012043
- Maierdan, Y., Haque, M. A., Chen, B., Maimaitiyiming, M., & Ahmad, M. R. (2020). Recycling of waste river sludge into unfired green bricks stabilized by a combination of phosphogypsum, slag, and cement. Construction and Building Materials, 260, 120666. https://doi.org/10.1016/j.conbuildmat.2020.120666
- Manjunath, B. T. A. (2016). Partial replacement of e-plastic waste as coarse-aggregate in concrete. Procedia Environmental Sciences, 35, 731–739. https://doi.org/10.1016/j.proenv.2016.07.079
- Muniraj, K., Asha, B., & Raja Ramachandran, P. (2014). Application of fine aggregate by replacement of tannery dry sludge in concrete. International Journal of Applied Environmental Sciences, 9, 2805–2815.
- Parmar, N. (2023). Major aggregate sources: Which factors affect the choice of sources of aggregates? Civil Giant. https://www.civilgiant.com/aggregate-sources/
- Parveen, S., Rana, S., & Fangueiro, R. (2013). A review on nanomaterial dispersion, microstructure, and mechanical properties of carbon nanotube and nanofiber reinforced cementitious composites. Journal of Nanomaterials, 2013, 1–19. https://doi.org/10.1155/2013/710175
- Qaidi, S., Najm, H. M., Abed, S. M., Özkılıç, Y. O., Al Dughaishi, H., Alosta, M., Sabri, M. M. S., Alkhatib, F., & Milad, A. (2022). Concrete containing waste glass as an environmentally friendly aggregate: A review on fresh and mechanical characteristics. Materials, 15(18), 6222. https://doi.org/10.3390/ma15186222
- Saeed, A., Najm, H. M., Hassan, A., Sabri, M. M. S., Qaidi, S., Mashaan, N. S., & Ansari, K. (2022). Properties and applications of geopolymer composites: A review study of mechanical and microstructural properties. Materials, 15(22), 8250. https://doi.org/10.3390/ma15228250
- Saikia, N., & De Brito, J. (2012). Use of plastic waste as aggregate in cement mortar and concrete preparation: A review. Construction and Building Materials, 34, 385–401. https://doi.org/10.1016/j.conbuildmat.2012.02.066
- Sakthivel, S., Sarathi, M., Sathish, S., & Sivakumar, M. (2019). Experimental investigation on textile mill sludge with partial replacement of fine aggregate in concrete. International Research Journal of Engineering and Technology (IRJET), 06(03), 7404–7407.
- Sandanayake, M. S. (2022). Environmental impacts of construction in building industry—A review of knowledge advances, gaps and future directions. Knowledge, 2(1), 139–156. https://doi.org/10.3390/knowledge2010008
- Siddique, R., Khatib, J., & Kaur, I. (2008). Use of recycled plastic in concrete: A review. Waste Management (New York, N.Y.), 28(10), 1835–1852. https://doi.org/10.1016/j.wasman.2007.09.011
- Singh, M., Choudhary, K., Srivastava, A., Singh Sangwan, K., & Bhunia, D. (2017). A study on environmental and economic impacts of using waste marble powder in concrete. Journal of Building Engineering, 13(February), 87–95. https://doi.org/10.1016/j.jobe.2017.07.009
- Sójka-Ledakowicz, J., Koprowski, T., Machnowski, W., & Knudsen, H. H. (1998). Membrane filtration of textile dyehouse wastewater for technological water reuse. Desalination, 119(1–3), 1–9. https://doi.org/10.1016/S0011-9164(98)00078-2
- Tahir, F., Sbahieh, S., & Al-Ghamdi, S. G. (2022). Environmental impacts of using recycled plastics in concrete. Material Today: Proceedings, 62, 4013–4017. https://doi.org/10.1016/j.matpr.2022.04.593
- Tam, V. W. Y. (2008). Economic comparison of concrete recycling: A case study approach. Resources, Conservation and Recycling, 52(5), 821–828. https://doi.org/10.1016/j.resconrec.2007.12.001
- Ullah, K., Irshad Qureshi, M., Ahmad, A., & Ullah, Z. (2022). Substitution potential of plastic fine aggregate in concrete for sustainable production. Structures, 35, 622–637. https://doi.org/10.1016/j.istruc.2021.11.003
- Zhan, B. J., & Poon, C. S. (2015). Study on feasibility of reutilizing textile effluent sludge for producing concrete blocks. Journal of Cleaner Production, 101, 174–179. https://doi.org/10.1016/j.jclepro.2015.03.083
- Zhao, Z., Courard, L., Groslambert, S., Jehin, T., Léonard, A., & Xiao, J. (2020). Use of recycled concrete aggregates from precast block for the production of new building blocks: An industrial scale study. Resources, Conservation and Recycling, 157(March), 104786. https://doi.org/10.1016/j.resconrec.2020.104786