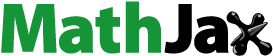
Abstract
Hemp fibers are becoming increasingly popular as a versatile reinforcement in natural fiber-reinforced composites, which have emerged as a sustainable alternative to traditional materials. With an emphasis on maximizing wear resistance, this study examines the wear properties of potassium permanganate (KMnO4)-treated Hemp fiber powder-reinforced epoxy composite specimens. The experiments on Pin-on-disc were conducted by varying the process parameters, such as time, load and speed to understand the wear damage. This study shed light on the influence of process parameters on the wear mechanism under various circumstances, by analyzing the mass loss of the specimens. The statistical analysis of the obtained results was performed using the Taguchi method at 95% confidence level. The lowest probability value (p values) was obtained for Time (0.018) followed by Speed (0.043). The study predicted that the mass loss was significantly influenced by time and speed. The surface morphology study of the tested specimens, performed using scanning electron microscopy (SEM) depicted that the mass loss of the specimens during the wear test is a combined effect of the formation of micro-cracks, micro ploughing, delamination and crater. The integration of Taguchi analysis and surface morphology study yield a thorough comprehension of the wear properties of natural fiber-reinforced composites, enabling their optimization for an extensive array of technical uses.
1. Introduction
Composites are materials that consist of two or more distinct components, each contributing its characteristic properties to create a final product with enhanced performance. These components, known as the matrix and the reinforcement, work together synergistically to produce desirable mechanical, thermal and physical properties. Composites have found widespread applications in various industries, including aerospace, automotive and construction, due to their lightweight nature, high strength-to-weight ratio, corrosion resistance and design flexibility. The versatile nature of composites makes them a better substitute for metals. In recent past, the synthetic fibres were even replaced by natural counterparts. These fibers can be seamlessly integrated into the composite matrix to enhance the material’s performance. In industries like automotive, where lightweight and eco-friendly materials are crucial, composites reinforced with natural fibers align perfectly with efficiency, emissions reduction and sustainability goals (Vassilopoulos, Citation2019; Padmaraj et al., Citation2020, Citation2021; Sukur et al., Citation2021). The use of natural fiber-reinforced composites in the automotive industry will reduce the weight by 40–50% as compared to the conventional metal structures. Also, the replacement of plastics by natural fiber-reinforced composites will reduce Carbon Dioxide emissions by about 60% (Sahu & Gupta, Citation2020).
Natural fibers, derived from renewable sources, such as plants and animals, have garnered significant attention as reinforcement materials in composites. One such recognized natural fiber is Hemp fiber, which possesses several desirable properties including high tensile strength, low density, biodegradability and excellent vibration-damping characteristics (Elfaleh et al., Citation2023). Moreover, Hemp cultivation requires minimal pesticides and fertilizers, making it an environmentally sustainable alternative to synthetic fibers. Composites, particularly those reinforced with natural fibers like Hemp, offer a compelling solution to light-weight automobile parts. These composites provide comparable mechanical properties to conventional materials while significantly reducing the weight of automotive components. Furthermore, they contribute to a more sustainable manufacturing process by reducing the dependence on non-renewable resources (Gholampour & Ozbakkaloglu, Citation2020; Maiti et al., Citation2022).
Wear characteristics are crucial in determining the durability and longevity of materials and composites. The study of wear characteristics of a material will help in understanding the mechanisms and modes of material degradation under various conditions, such as friction, abrasion and fatigue (Mahesha et al., Citation2018). Wear phenomena can manifest in various forms, with the most common type being the adhesive wear, the abrasive wear and the fatigue wear. Adhesive wear occurs when two contacting surfaces experience localized welding and subsequent tearing, resulting in material transfer and surface damage. On the other hand, abrasive wear involves material removal due to hard particles or asperities on the counter-surface. The fatigue wear typically arise from cyclic loading, which causes crack initiation, propagation and eventual failure of material (Elfaleh et al., Citation2023; Krishnasamy et al., Citation2022).
Significant research efforts have been devoted to examining the wear characteristics of various natural fiber-reinforced composite materials. Inbakumar and Ramesh (Citation2018) performed experiments on Hemp fiber and eggshell-reinforced composite materials. Mechanical tests were conducted to evaluate their performance including hardness, tensile, impact, and flexural strength. The abrasive wear behavior of the specimens was studied using a pin-on-disc machine. The mechanical test results revealed that by introducing the fiber, the load-bearing characteristics of the composites were significantly enhanced. De Fazio et al. (Citation2019) studied the tribological behavior of Hemp, glass and carbon/epoxy hybrid composites by conducting pin-on-disc tests under various conditions, primarily varying the applied load (10, 20, 50 and 70 N). The results indicated a notable improvement in the wear resistance of the Hemp composite, particularly under high-load conditions. S. Kumar et al. (Citation2019) studied the effect of the addition of rice husk fillers on physical, mechanical and sliding wear properties of hybrid Bauhinia-vahlii-weight (BVW) and Bauhinia-vahlii-weight/sisal (BVWS) fiber-reinforced hybrid composites. The influence of selected control factors, sliding velocity, rice husk content, average load and sliding distance on the specific wear rate (SWR) of the composites was investigated using the Taguchi experimental design. This research established that the addition of 4 wt.% rice husk filler reflected in the improvement in mechanical and wear performance of natural fiber-based epoxy composites. Shivamurthy et al. (Citation2009) prepared E-glass woven fabric-Epoxy (LY 556) composites with varying amounts of Silicon dioxide (SiO2). The sliding wear test was carried out at a velocity of 5 m/s and a constant abrading distance of 1200 m with different loads, viz., 30, 60 and 90 N, on a pin-on-disc machine. The 3 and 6 wt.% of SiO2 particulate-filled composites showed better performance in sliding wear resistance. A further increase in the filler loading was not beneficial to flexural and sliding wear performance. Gupta (Citation2022) studied the erosion behavior of short bamboo fiber and Cenosphere fly ash-reinforced polymer composites. An air jet-type test rig was used for the erosion study following the design of the experiment’s approach using Taguchi’s orthogonal array. A significant improvement in the erosion behavior of the specimens was reported with the addition of the Cenosphere at 10 wt.% and 20 wt.%. Sampathkumaran et al. (Citation2000) studied the sliding wear characteristics of a Glass–Epoxy composite filled with either rubber or oxide particles using a block-on-roller test configuration. The worn surfaces were also examined by scanning electron microscopy (SEM). The oxide-filled composite had lower wear at low loads, while the rubber-filled composite had lower wear at high loads. The work also showed that higher loads and sliding velocities changed the worn surface features. Omrani et al. (Citation2016) performed experiments to demonstrate the tribological behavior of natural fiber-reinforced composites for various applications. The findings indicated that the natural fiber-reinforced composites exhibit enhanced tribological properties comparable to synthetic fiber-reinforced composites. Moonart and Utara (Citation2019) studied the effect of surface treatment methods, such as silane and permanganate treatment on curing characteristics, and mechanical and thermal properties of Hemp fiber-filled natural rubber composites. The morphological properties of the untreated and treated Hemp fiber specimens were then investigated by attenuated total reflectance-Fourier transform infrared spectroscopy (FTIR), X-ray diffraction (XRD) and SEM. The work by Sumesh et al. (Citation2022) focuses on the wear characteristics of hybrid composites prepared using Sisal fiber (SF)/Pineapple fiber (PF) and Pineapple fly ash (PA) in various wt.%. A linear reciprocating tribometer was used to determine the SWR and coefficient of friction (CoF) of the hybrid composite. SEM observations revealed that the surface deformations of the specimens depended on filler content, loading conditions, and percentage of reinforcement. Suthan et al. (Citation2020) presented the wear and morphological analysis of treated and untreated bio-fillers reinforced polymer composites. Four different bio-fillers, viz. rice husk, groundnut shell powder, coconut shell powder and hybrid filler were used to prepare the composites. The tests were conducted on pin-on-disc apparatus to evaluate the wear characteristics of treated and untreated bio-filler reinforced epoxy composites. The test results exhibited a significant improvement in the wear resistance of the treated hybrid bio-filler composite.
The literature study shows that the wear rate of natural fiber-reinforced composites is significantly influenced by several key parameters, including load, sliding velocity, contact pressure, temperature, material properties and environmental conditions. Higher applied loads and sliding speeds generally increase the wear rate due to the increased contact forces and higher material removal rate. The contact pressure is critical in affecting the extent of deformation and surface damage. Temperature can also influence wear behavior by altering the material’s mechanical properties, thermal expansion and chemical reactions at the interface. Furthermore, material properties, such as hardness, toughness and lubrication significantly impact wear resistance. Environmental factors, such as humidity and exposure to aggressive media can also accelerate the wear damage. Understanding these parameters is crucial for optimizing the design and performance of composite materials in wear-prone applications.
The application of Hemp-based composites in the automotive industry has increased at a rapid rate and is replacing the conventional materials by about 7%. These materials are subjected to different loading conditions in their service life and will result in mass loss or surface wear. The present research concentrates on developing a strategy to optimize the wear resistance of composites reinforced with Hemp powder at different operating conditions. To improve the compatibility of the Hemp fiber with epoxy resin, the fibers were treated with Potassium Permanganate (KMnO4) solution. The pin-on-disc wear test was performed on the Hemp powder filled epoxy composites by varying the applied load, speed and duration. The experiments were performed based on Taguchi’s experimental design to identify the effects of process parameters on wear characteristics. SEM was used to carefully investigate the wear surface, which gave important information on the structural alterations and wear mechanisms of the material.
2. Materials and methods
2.1. Fiber treatment
Natural fibers are highly sought after for composite materials due to their abundance and biodegradability. In this study, the Hemp was procured from the supplier Go Green Products, Chennai, India. Epoxy (Lapox L12) was used as a matrix material, and K6 Hardener was used as a curing agent to fabricate composites. It was procured from Yuje Enterprises, Bangalore, India. As part of the fiber treatment process, the fibers were initially soaked in a solution of 5 L of water and 50 g of KMnO4 for approximately 3 min to improve the surface interaction with the epoxy matrix, as shown in . The fibers were then rinsed with fresh water and subsequently dried at ambient temperature for 24 h. The treated fibers were placed in a hot air oven at 50 °C for 1 h to ensure complete drying. The treatment transformed the fiber color from yellowish-white to deep brown. The treatment also helped to optimize the performance of the Hemp fiber, resulting in a composite material with improved strength and durability.
2.2. Composite preparation
In this study, the Hemp fiber is reinforced with Epoxy in the form of powder. The treated hemp fiber was finely chopped into powder form to facilitate mixing with the matrix material. The microscopic analysis of the filler revealed that it exhibits a rough texture with cylindrical and irregular shapes of sizes ranging from 0.1 to 0.2 mm as shown in . The void content and hardness of the filler-based composites depend on the percentage of filler content. Literatures suggest that a 5–10% filler content in composites can provide an optimum void content and hardness value (Reddy et al., Citation2022; Saravanakumaar et al., Citation2021). Based on this, the composite specimen was prepared by reinforcing 5% (by weight) of Hemp powder with Epoxy matrix. The mixture was then poured into a wooden mould and left to cure for 24 h at room temperature, as shown in . The specimens from the were cut into small cuboid pieces as per the ASTM G99 standards (25 × 25 × 8 mm) using waterjet machining (Krishnasamy et al., Citation2022; Khan et al., Citation2022). The experimental density of the prepared specimens was calculated using the Archimedes principle. A total of 27 specimens were weighed in a digital weighing machine with an accuracy of 0.001 g. The average experimental density of the laminate was found to be 1.14 ± 0.003 g/cm3. The average micro Vickers hardness test of the prepared specimens measured using MATSUZAWA make hardness tester was found to be 45.4 ± 5.4 HV.
2.3. X-ray diffraction (XRD)
XRD examination, a quick and non-destructive diagnostic technique, was used to evaluate the Hemp fibers’ degree of crystallinity and crystalline size. The anode used in the XRD was made of copper, which produced monochromatic Cu Kα radiation with a wavelength of 0.154 nm when operated at 30 mA current and 30 kV voltage. With a step size of 0.02° at 25 °C, a continuous scanning mode was used to cover a 2θ range from 5° to 60°. The crystallinity and crystallite size levels were determined using Scherer’s equation and Segal’s empirical technique (EquationEquations (1)(1)
(1) and Equation(2)
(2)
(2) ) (Moussaoui et al., Citation2021; Ighalo et al., Citation2021).
(1)
(1)
(2)
(2)
In EquationEquation (1)(1)
(1) , Ic stands for the peak’s maximum crystalline intensity, and Iam for the peak’s maximum amorphous phase intensity of the treated fiber. In EquationEquation (2)
(2)
(2) , the Scherer’s constant (K = 0.89), β is the peak’s full width at half-maximum, λ is the wavelength of X-ray radiation and θ is the Bragg angle.
2.4. Wear test
The main objective of the present research work is to understand the wear characteristics of the Hemp powder-filled epoxy composites. Wear characteristics of the specimens were investigated using the Ducom make Pin on Disc wear test apparatus, and the schematic representation of the setup is shown in . The wear tests were performed by varying the operating parameters, such as Load, Speed and Time, at different levels. The wear characteristics of the specimens (25 × 25 × 8 mm) were evaluated in the form of mass loss. The initial mass and final mass of the specimen (after the wear test) were measured using a digital weighing scale with an accuracy of 0.001 g.
2.5. Taguchi analysis
A series of experiments were diligently executed utilizing a three-factor, three-level Taguchi methodology to delve into the nuanced influence of individual parameters and their potential interaction effects on the wear performance of Hemp powder reinforced epoxy composites (Khan et al., Citation2022).
The experimental procedures were meticulously conducted under ambient conditions, maintaining a constant room temperature. The experimental setup followed the Taguchi L-27 full factorial design, where three distinct factors were meticulously manipulated across three levels: high, medium and low, as shown in . The controlled variation of applied load spanned a range from 10 to 30 N. This enabled a systematic investigation into the direct relationship between applied load and the resulting wear patterns exhibited by the composite material. Additionally, the test speed, a pivotal determinant in the wear process, was scrutinized. The range of speed levels selected, ranging between 500 and 1000 RPM, was judiciously determined, considering the operational limitations of the machinery involved. Specimens were tested for durations of 10, 20 and 30 min to have a better understanding on the effect of wear on the changing time duration (Reddy et al., Citation2022; Davim & Cardoso, Citation2009; Keerthiveettil Ramakrishnan et al., Citation2022).
Table 1. Parameters and their different levels.
2.6. Scanning electron microscope (SEM)
A SEM is an effective scientific tool that employs concentrated electron beams to create high-resolution, 3D images of the surface structure and composition of materials at the nanoscale, enabling in-depth analysis in various scientific and industrial fields. Three specimens with low, medium, and high wear were chosen for SEM examination. The Quorum gold sputtering equipment was used to gold sputter the specimens as they were manufactured from Hemp and needed improved conductivity and picture contrast. The specimens were analyzed using the Zeiss EVO 18 from Oxford Industries at the Central Instrumentation Facility Manipal.
3. Results and discussions
3.1. Effect of treatment on fiber content
represents the XRD spectra of treated and untreated Hemp fibers. The pattern elucidates the presence of semi-crystalline amorphous peaks of cellulose. The characteristic peaks of 2θ angles at around 16° and 22° are depicted by (110) planes and (200) planes, respectively, as per previously published results (Gholampour & Ozbakkaloglu, Citation2020; Shahzad, Citation2012; Vigneshwaran et al., Citation2020). The crystal plane (200) peak at 2θ is obtained at around 22° for treated and untreated Hemp fibers. In contrast, the amorphous contribution is at 18.5°, which is evident in the treated and untreated fibers. shows that the intensity of treated fiber is higher than that of its counterpart, indicating that the treated fiber’s increased degree of crystallinity is higher than that of untreated fiber. This can be attributed to removing the gum base and impurities from the untreated fiber during the KMnO4 treatment, leading to exposure of higher cellulose content in the specimens, providing better diffraction of X-rays resulting in higher intensity peaks. Treatment of fiber with KMnO4 removes the disoriented cellulose and low molecular weight amorphous components from the fiber surface. The treated fiber is mainly composed of ordered cellulose with a rough surface. The treatment also removes the microvoids from the fiber surface and reduces the water affinity of the fiber surface. The formation of a rough fiber surface due to KMnO4 treatment increases the fiber/matrix adhesion and provides better wear characteristics at different loading scenarios (Pereira et al., Citation2021; Krishnaiah et al., Citation2017).
One of the critical characteristics of nano cellulose that drives spatial organization and symmetry and significantly impacts its mechanical, chemical and physical properties is its crystallinity. The crystallinity index ranges from 70% to 88% for cellulose nanocrystals, depending on the source material and pretreatment techniques.
3.2. Experimental results
The wear assessment of Hemp powder/Epoxy specimens was performed using the Pin-on-Disc apparatus. Two sets of experiments were conducted to understand the effect of process parameters on the wear characteristics. The average mass loss of specimens in each experiment is shown in .
Table 2. Taguchi L27 experimental design and wear response of specimens.
3.3. Effect of control parameters on wear behavior
Within the framework of the Taguchi design of experiments, the signal-to-noise (S-N) ratio assumed significance as a chosen quality metric for the analysis. The adoption of the S-N ratio stems from its capacity to provide a quantifiable value, circumventing the limitations of the standard deviation. This approach avoids the confounding effect that changes in the mean can have on the standard deviation and vice versa. Notably, the S-N ratio finds applicability in diverse scenarios, including quality enhancement via variance reduction and the refinement of measurement precision. Given the objective of minimizing wear, a ‘smaller-the-better’ approach, inherent in Taguchi analysis, has been employed in this study as per Equation (3) (Khan et al., Citation2022; Kumar et al., Citation2021).
Smaller is the best characteristic, S/N = −10 log1/n (Σy2) (3)
where ‘y’ represents the collected data, and ‘n’ denotes the count of observations. The outcomes of the S-N ratio analysis are summarized in , presenting the S-N ratios corresponding to various process parameters and their respective levels. The dominant influencer on wear is time, followed by speed and load.
Table 3. Response table for S-N ratios.
illustrates the distinct impact of individual parameters on wear. Notably, wear escalates the most with an increase in time. The experiments were conducted with different load conditions. Consequently, elevated loads amplify the force at any given point on the surface, leading to a heightened interaction with particles and more pronounced wear. An increase in speed initially led to a notable rise in wear, but this effect diminished with a further increase in speed. Particularly, specimens tested at 500 RPM exhibited the lowest wear, while wear remains relatively consistent at 750 and 1000 RPM, with only a minor increase observed at 1000 RPM. This indicates an enhanced resistance of the material to wear at 500 RPM. The effect of speed diminished below 750 RPM. The experiments conducted above 750 RPM increased the temperature between the specimen surface and the disc interface. The formation of a temperature gradient between two surfaces can induce thermal stresses in the target surface. The thermal stress will initiate debonding between the fiber/matrix interphase (Reddy et al., Citation2022). The mass loss of the specimen is directly proportional to the bonding strength between the fiber/matrix. Due to this, the experiments performed at higher RPM (750 and 1000 RPM) results in a higher amount of material removal from the target surface.
An insightful analysis of variance, outlined in , offers a comprehensive view of the impact of the process parameters and their interactions on wear. The assessment of the significance of these factors in the wear test is facilitated through the p values. To be deemed significantly impactful at a 95% confidence level, a factor should possess a p value below 0.05. The analysis highlights that time and speed have notably lower p values, affirming their role as the most influential parameters on mass loss.
Table 4. Analysis of variance for mass loss of the composite.
delves into the interaction effects of various factors on the wear of Hemp powder-reinforced composite. Notably, the absence of parallel lines suggests intersecting effects. Regarding the interaction analysis of load and speed, it is evident that there is no noticeable interaction at low speed but a subtle interaction potential surface at high speeds. The speed vs. load and speed vs. time exhibit no significant interactions. An interaction plot reveals a significant interaction between time and load, suggesting that wear depends on both time and load. While there is no interaction at low speed, a considerable interaction emerges at high speed. The temperature gradient between the specimen and the disc surface will be high at higher speeds. The application of higher axial thrust force (20 and 30 N) at high speed (750 and 1000 RPM) will increase the thermal stresses on the target surface. This inturn softens the material, and increases the mass loss, and reduces the wear resistance of the material (Reddy et al., Citation2022; Davim & Cardoso, Citation2009). These findings provide valuable insights to the wear behavior of Hemp powder-reinforced composites, highlighting the nuanced effects of load, speed and time on wear resistance.
3.4. Wear surface morphology analysis
The surface morphology of the specimens was studied using SEM. The specimens for the study were selected based on the mass loss, and classified as high (30 N, 750 RPM and 30 min), medium (30 N, 500 rpm and 30 min) and low mass loss (10 N, 500 RPM and 10 min) and are shown in , respectively.
As the majority of the stress is centered on the leading edge, this is where the specimen surface shows the highest wear. The highest amount of wear is observed at the leading edge of the specimen surface, where the majority of stress is concentrated. Hence, this study focuses on the leading edge to evaluate the specimen’s wear mechanism. (a) shows the surface morphology of the specimens with lower mass loss. The surface is composed of wear debris, most likely from adhesive wear, which has resulted in filler pullouts and apparent microcracks. The composite has microcracks due to mechanical forces, including compression and shear stresses. The addition of Hemp powder in matrix material reduced the crack propagation rate in the specimens (Wang et al., Citation2020). (b) shows the surface morphology of the composite with moderate wear throughout the experiment. Application of high load and testing speeds resulted in multiple surface cracks and delamination. These occurrences are explained by the composite’s brittleness, which resulted in surface fatigue failure. (c) shows the surface morphology of the specimen with significant wear. As the load and speed increased, the target surface was subjected to higher thermal stresses. The combined effect of thermal stress and operating parameters softened the target surface. This resulted in severe delamination, widespread crater development and total particle separation from the surface. Compression, fatigue and shear stress also contribute to excessive mechanical stress, which causes delamination in the specimen. Furthermore, as (c) illustrates, the wear rate has resulted in surface smearing.
4. Conclusion
Applying Hemp-based composites in the automotive sector presents a viable approach for manufacturing door, floor, and dashboard panels due to the low density and better compatibility of Hemp with the matrix material. The study focused on the types of wear found during the service life of the aforementioned automotive body. This study used the Taguchi statistical approach to understand wear on Hemp-based composites. The XRD analysis showed that the crystallinity of Hemp fiber improved on treatment with KMnO4 solution due to the removal of gum-based impurities from the untreated fiber surface. A thorough specimen level pin on disc wear test was conducted on hemp-filled epoxy composites using the Taguchi experimental method, emphasizing mass loss. The analysis of process parameters showed that the duration of the test or time (p value = 0.018) highly impacts the mass loss, followed by the speed (p value = 0.043). The results showed that the applied load was the least significant influencing parameter. The high test speed induced thermal stresses at different loading conditions and induced matrix softening and resulted in higher mass loss. Surface morphology examination confirmed the different wear patterns that complemented these findings. Specimens with high wear showed micro-cracks, debonding with large debris/flakes. Specimens with medium wear revealed wear debris and spalling, and specimens with low wear showed micro-crack initiation. These revelations aid in comprehending wear properties and direct the industry’s strategic application of Hemp-filled epoxy composites.
5. Future work
There are several ways to analyze wear characteristics to comprehensively analyze the performance of hemp-filled epoxy composites. This entails modifying variables, such as fiber length, surface treatment and weight percentages of Hemp filler to comprehend their influence on wear behavior and specific wear rate. Comparing various matrix alternatives is made possible by experimenting with matrix materials, such as polyester and polypropylene, which provide insights into wear behavior. Enhancing composite flexibility by hybridization with fillers including glass fibers, carbon fibers and bio-based fibers like coir, sisal and rice husk permits studies into how various combinations affect wear behavior.
Author contributions
Pruthviraj Hemanth Patil, Rahul K., Pranam Shetty, Vineeth Jeevan Dias – Experimental work, Formal Analysis, Data Curation and Writing – Original Draft and Writing; Thara Reshma I. V. – Data Curation and Review and Editing; Manjunath Shetty–Formal Analysis, Writing – Review and Editing; Padmaraj N. H. – Conceptualization, Methodology, Formal Analysis and Supervision.
Acknowledgments
The authors were greatly thankful to Manipal Institute of Technology, Manipal Academy of Higher Education, Manipal and N.M.A.M. Institute of Technology, Nitte, Karkala, Karnataka, India for providing the composite manufacturing and testing facility.
Disclosure statement
The authors declare that they have no known competing financial interests or personal relationships that could have appeared to influence the work reported in this article.
Data availability
The data will be made available on request.
References
- Davim, J. P., & Cardoso, R. (2009). Effect of the reinforcement (carbon or glass fibres) on friction and wear behaviour of the PEEK against steel surface at long dry sliding. Wear, 266(7–8), 795–799. https://doi.org/10.1016/j.wear.2008.11.003
- De Fazio, D., Boccarusso, L., & Durante, M. (2019). Tribological behaviour of hemp, glass and carbon fibre composites. Biotribology, 21, 100113. https://doi.org/10.1016/j.biotri.2019.100113
- Elfaleh, I., Abbassi, F., Habibi, M., Ahmad, F., Guedri, M., Nasri, M., & Garnier, C. (2023). A comprehensive review of natural fibers and their composites: An eco-friendly alternative to conventional materials. Results Engineering, 19, 101271. https://doi.org/10.1016/j.rineng.2023.101271
- Gholampour, A., & Ozbakkaloglu, T. (2020). A review of natural fiber composites: Properties, modification and processing techniques, characterization, applications (Vol. 55). Springer US.
- Gupta, A. (2022). Influence of filler content on tribological behavior of cenopshere flyash filled bamboo fiber reinforced composites. Journal of Natural Fibers, 19(15), 12051–12067. https://doi.org/10.1080/15440478.2022.2051666
- Ighalo, J. O., Adeyanju, C. A., Ogunniyi, S., Adeniyi, A. G., & Abdulkareem, S. A. (2021). An empirical review of the recent advances in treatment of natural fibers for reinforced plastic composites. Composite Interfaces. 28(9), 925–960. https://doi.org/10.1080/09276440.2020.1826274
- Inbakumar, J. P., & Ramesh, S. (2018). Mechanical, wear and thermal behaviour of hemp fibre/egg shell particle reinforced epoxy resin bio composite. Transactions of the Canadian Society for Mechanical Engineering, 42(3), 280–285. https://doi.org/10.1139/tcsme-2017-0079
- Keerthiveettil Ramakrishnan, S., Vijayananth, K., Pudhupalayam Muthukutti, G., Spatenka, P., Arivendan, A., & Ganesan, S. P. (2022). The effect of various composite and operating parameters in wear properties of epoxy-based natural fiber composites. Journal of Material Cycles and Waste Management, 24(2), 667–679. https://doi.org/10.1007/s10163-022-01357-1
- Khan, M. M., Dey, A., & Hajam, M. I. (2022). Experimental investigation and optimization of dry sliding wear test parameters of aluminum based composites. Silicon, 14(8), 4009–4026. https://doi.org/10.1007/s12633-021-01158-5
- Krishnaiah, P., Ratnam, C. T., & Manickam, S. (2017). Enhancements in crystallinity, thermal stability, tensile modulus and strength of sisal fibres and their PP composites induced by the synergistic effects of alkali and high intensity ultrasound (HIU) treatments. Ultrasonics Sonochemistry, 34, 729–742. https://doi.org/10.1016/j.ultsonch.2016.07.008
- Krishnasamy, P., Rajamurugan, G., Aravindraj, S., & Sudhagar, P. E. (2022). Vibration and wear characteristics of aloevera/flax/hemp woven fiber epoxy composite reinforced with wire mesh and BaSO4. Journal of Natural Fibers, 19(8), 2885–2901. https://doi.org/10.1080/15440478.2020.1835782
- Kumar, S., Mer, K. K. S., Gangil, B., & Patel, V. K. (2019). Synergy of rice-husk filler on physico-mechanical and tribological properties of hybrid Bauhinia-vahlii/sisal fiber reinforced epoxy composites. Journal of Materials Research and Technology, 8(2), 2070–2082. https://doi.org/10.1016/j.jmrt.2018.12.021
- Kumar, S., Patel, V. K., Mer, K. K. S., Gangil, B., Singh, T., & Fekete, G. (2021). Himalayan natural fiber-reinforced epoxy composites: Effect of grewia optiva/bauhinia vahlii fibers on ohysico-mechanical and dry sliding wear behavior. Journal of Natural Fibers, 18(2), 192–202. https://doi.org/10.1080/15440478.2019.1612814
- Mahesha, G. T., Satish Shenoy, B., Vijaya Kini, M., & Padmaraj, N. H. (2018). Wear behaviour studies on Grewia Serrulata bast fibre reinforced polymer composites. Cogent Engineering, 5(1). article no 1517580. https://doi.org/10.1080/23311916.2018.1517580
- Maiti, S., Islam, M. R., Uddin, M. A., Afroj, S., Eichhorn, S. J., & Karim, N. (2022). Sustainable fiber-reinforced composites: A review. Advanced Sustainable Systems, 6(11), 2200258.
- Moonart, U., & Utara, S. (2019). Effect of surface treatments and filler loading on the properties of hemp fiber/natural rubber composites. Cellulose, 26(12), 7271–7295. https://doi.org/10.1007/s10570-019-02611-w
- Moussaoui, N., Rokbi, M., Osmani, H., Jawaid, M., Atiqah, A., Asim, M., & Benhamadouche, L. (2021). Extraction and characterization of fiber treatment inula viscosa fibers as potential polymer composite reinforcement. Journal of Polymers and the Environment, 29(11), 3779–3793. https://doi.org/10.1007/s10924-021-02147-w
- Omrani, E., Menezes, P. L., & Rohatgi, P. K. (2016). State of the art on tribological behavior of polymer matrix composites reinforced with natural fibers in the green materials world. Engineering Science and Technology, an International Journal, 19(2), 717–736. https://doi.org/10.1016/j.jestch.2015.10.007
- Padmaraj, N. H., Vijaya, K. M., & Dayananda, P. (2020). Experimental study on the tension-tension fatigue behaviour of glass/epoxy quasi-isotropic composites. Journal of King Saud University - Engineering Sciences, 32(6), 396–401. https://doi.org/10.1016/j.jksues.2019.04.007
- Padmaraj, N.H., Vijaya, K. M., Amritha, U., Dayananda, P., & Shreepannaga. (2021). Slurry erosion behaviour of carbon/epoxy quasi-isotropic laminates based on Taguchi’s optimization method. Engineering Failure Analysis, 123, 105274. https://doi.org/10.1016/j.engfailanal.2021.105274
- Pereira, P. H. F., Ornaghi, H. L., Arantes, V., & Cioffi, M. O. H. (2021). Effect of chemical treatment of pineapple crown fiber in the production, chemical composition, crystalline structure, thermal stability and thermal degradation kinetic properties of cellulosic materials. Carbohydrate Research, 499, 108227. https://doi.org/10.1016/j.carres.2020.108227
- Reddy, P. V., Reddy, R. V. S., Rajendra Prasad, P., Mohana Krishnudu, D., Reddy, R. M., & Rao, H. R. (2022). Evaluation of mechanical and wear performances of natural fiber reinforced epoxy composites. Journal of Natural Fibers, 19(6), 2218–2231. https://doi.org/10.1080/15440478.2020.1807441
- Sahu, P., & Gupta, M. K. (2020). A review on the properties of natural fibres and its bio-composites: Effect of alkali treatment. The Proceedings of the Institution of Mechanical Engineers, Part L: Journal of Materials: Design and Applications, 234(1), 198–217. https://doi.org/10.1177/1464420719875163
- Sampathkumaran, P., Seetharamu, S., Vynatheya, S., Murali, A., Kumar, R. K., & Kishore. (2000). SEM observations of the effects of velocity and load on the sliding wear characteristics of glass fabric-epoxy composites with different fillers. Wear, 237(1), 20–27. https://doi.org/10.1016/S0043-1648(99)00300-2
- Saravanakumaar, A., Senthilkumar, A., & Rajan, B. M. C. (2021). Effect of fillers on natural fiber-polymer composite: An overview of physical and mechanical properties. Mechanical and dynamic properties of biocomposites (pp. 207–233). Wiley.
- Shahzad, A. (2012). Hemp fiber and its composites - A review. Journal of Composite Materials. 46(8), 973–986. https://doi.org/10.1177/0021998311413623
- Shivamurthy, B., Siddaramaiah., & Prabhuswamyc, M. S. (2009). Influence of SiO2 fillers on sliding wear resistance and mechanical properties of compression moulded glass epoxy composites. Journal of Minerals and Materials Characterization and Engineering, 08(07), 513–530. https://doi.org/10.4236/jmmce.2009.87045
- Sukur, E. F., Kaybal, H. B., Ulus, H., & Avci, A. (2021). Tribological behavior of epoxy nanocomposites under corrosive environment: Effect of high-performance boron nitride nanoplatelet. Sigma Journal of Engineering and Natural Sciences, 41(2), 385–395.
- Sumesh, K. R., Kavimani, V., Rajeshkumar, G., Ravikumar, P., & Indran, S. (2022). An investigation into the mechanical and wear characteristics of hybrid composites: Influence of different types and content of biodegradable reinforcements. Journal of Natural Fibers, 19(8), 2823–2835. https://doi.org/10.1080/15440478.2020.1821297
- Suthan, R., Jayakumar, V., & Bharathiraja, G. (2020). Wear analysis of bio-fillers reinforced epoxy composites. Materials Today: Proceedings, 22, 793–798. https://doi.org/10.1016/j.matpr.2019.10.154
- Vassilopoulos, A. P. (2019). The history of fiber-reinforced polymer composite laminate fatigue. International Journal of Fatigue, 134, 105512. https://doi.org/10.1016/j.ijfatigue.2020.105512
- Vigneshwaran, S., Sundarakannan, R., John, K. M., Joel Johnson, R. D., Prasath, K. A., Ajith, S., Arumugaprabu, V., & Uthayakumar, M. (2020). Recent advancement in the natural fiber polymer composites: A comprehensive review. Journal of Cleaner Production, 277, 124109. https://doi.org/10.1016/j.jclepro.2020.124109
- Wang, X., Tang, F., Cao, Q., Qi, X., Pan, H., Lin, Z., & Battocchi, D. (2020). Nano-modified functional composite coatings for metallic structures: Part II—Mechanical and damage tolerance. Surface and Coatings Technology, 401, 126274. https://doi.org/10.1016/j.surfcoat.2020.126274