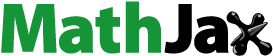
Abstract
This article calculates and studies the oil flow distribution, temperature distribution and electric field distribution of vegetable and mineral Insulating oil in the low-voltage winding of the transformer with finite element method (FEM). The results show that the maximum flow rates of vegetable Insulating oil and mineral oil are located on the side wall of low-voltage winding at 0.1, 0.3 and 0.6 m/s inlet velocities, and the maximum flow rate of mineral oil is higher. In addition, the increase of inlet flow rate will also cause the temperature inside the low voltage (LV) winding to drop. Due to the low viscosity of mineral oil, its heat transfer efficiency is higher. In addition, at the same flow rate, the pressure of mineral oil in the low-voltage winding is smaller, which is also due to the low viscosity and strong fluidity of mineral oil.
Reviewing editor:
1. Introduction
Power transformer is the core for transforming voltage and transmitting current, and also the core electrical equipment of distribution network. Therefore, the safety and reliability of the transformer directly affect the normal operation of the distribution network (Rapp & Luksich, Citation2011). Since the no-load loss (Mehta et al., Citation2016) and short-circuit loss (Martins, Citation2010) of the transformer account for the majority of the transformer loss, only these two parts of loss are considered in the conventional calculation of transformer loss. However, losses can also occur on leads, shells and other structural metal parts. Such losses can be classified as stray losses (Jeong et al., Citation2012). With the increase of transformer capacity, the leakage flux is increasing, which will inevitably increase the stray loss of power transformer structural components. In large power transformers, the leakage flux generated by winding current will inevitably cause load loss, and the loss distribution is uneven, which often leads to local overheating (Shao et al., Citation2014). Therefore, it is particularly important to study the leakage flux and stray loss thoroughly and accurately.
With the development of simulation calculation represented by finite element method (FEM), simulation analysis of temperature rise characteristics and fluid field distribution of electrical equipment is widely used at home and abroad (Joo et al., Citation2006). At present, the temperature and flow field distribution of insulating oil in the transformer is mostly studied by means of temperature-fluid field coupling simulation. Chen Mina (Chen et al., Citation2020) calculated the leakage flux and stray loss of power transformer structural components using three-dimensional nonlinear time-harmonic FEM. The results show that stray loss can be effectively eliminated and local overheating can be reduced by adding magnetic shielding. Jin Hong et al. (Citation2007) and others studied the characteristics of the electric field distribution of the high voltage (HV) winding of dry-type transformer based on ANSYS, and the results showed that the most uneven position of the electric field distribution is the junction of wire turns, spacers and air gaps. Based on the FEM, Li Jianxun et al. (Citation2022) and others explored the influence of oil flow speed and internal structure on the temperature rise of converter transformer, and analyzed the influence mechanism of different factors on the temperature field of converter transformer.
Mineral oil as insulating oil for transformers accounts for 99% of the total amount of oil-immersed transformers. It has excellent insulation performance, good stability and low cost, but its degradation time is long and its environmental performance is poor. Relevant research shows that the biodegradation rate of mineral-insulating oil is only about 30% (Wang, Citation2018). In case of leakage, mineral insulating oil will remain in the soil for a long time, causing changes in soil structure, causing irreversible damage to the surrounding environment and with the excessive development of oil resources, it will eventually face energy shortage and depletion, which will restrict the development of oil-immersed transformers in the future. Natural ester oil is derived from vegetable oil. Its raw material can be regenerated repeatedly, its output can be adjusted manually, its natural degradation time is short, and its biodegradation rate is high (Thirugnanam, Siluvairaj, & Karthik, et al., Citation2017; Thirugnanam, Siluvairaj, & Radha, et al., Citation2017; Samikannu et al., Citation2022). Compared with mineral oil transformer, natural ester oil has longer service life and stronger overload capacity. It is an ideal iterative product of mineral-insulating oil. However, natural ester oil has higher thermal conductivity and viscosity than traditional mineral insulating oil, directly replacing mineral insulating oil with natural ester insulating oil will have a significant impact on the heat dissipation of the entire insulation system of the transformer (Thirugnanam, Siluvairaj, & Karthik, et al., Citation2017; Thirugnanam, Siluvairaj, & Radha, et al., Citation2017).
Therefore, it is of great engineering significance to carry out simulation research on electric field, temperature and fluid field of vegetable oil and mineral oil transformer, analyze and compare the difference of electric field distribution, temperature distribution and oil flow rate between mineral oil and natural ester oil in the transformer, which can provide basis for the design and improvement of transformer and the improvement of physical and chemical properties of natural ester oil.
2. Model construction and simulation methodology
2.1. Simulation method
Scalar potential function φ simplifies the electric field calculation. In an electrostatic field, the electric field strength E satisfies ∇ × E = 0. Therefore, when conducting electric field simulation for this problem, the law of charge conservation can be used for calculation:
(1)
(1)
(2)
(2)
The sum of no-load loss and load loss generated during operation of a transformer is the residual heat of the transformer. The physical process of residual heat removal mainly involves solving the thermal field and flow field. To simulate the heat flow field of a transformer winding, it is necessary to determine the form of the oil flow of the insulating oil, which can be represented by the Reynolds number (Re), as shown in EquationEquation (3)(3)
(3) . When the Reynolds number is small, the influence of viscous force on the flow field is dominant. Due to the existence of viscous force, the disturbance of flow velocity in the flow field gradually attenuates, and the fluid flow is relatively stable, so it is laminar flow. When Reynolds number is large, the influence of inertial convection field dominates, and fluid flow is unstable, leading to the formation of irregular turbulent flow fields.
(3)
(3)
where Re is the Reynolds number, ρ Is the density, v is the flow rate, d is the characteristic length, and μ is the viscosity coefficient. According to the calculation, the flow form of the transformer oil is laminar flow, which is calculated using the Navier-Stokes equation and heat conduction equation:
(4)
(4)
(5)
(5)
(6)
(6)
(7)
(7)
(8)
(8)
2.2. Model boundary condition settings
In this article, a power transformer with a rated capacity of 25,000 kVA is used as a prototype to build a simulation model. The rated voltage of the HV coil of the transformer is 110 kV, the rated voltage of the medium voltage (MV) coil is 35 kV, and the rated voltage of the low voltage (LV) coil is 10.5 kV. Because the phase current of the LV coil of the AC transformer is the largest, and the hot spot generally appears on the LV coil, this article takes the LV coil as the research object of the transformer thermal field.
The high and LV conductors are set as the second homogeneous boundary condition. Because the electric field is solved by the potential divergence, the electric field intensity at the tip (such as the four corners of a rectangle) can be calculated as infinity. This phenomenon is reflected in the finite element solution that the finer the grid, the greater the electric field intensity. Therefore, the details of chamfer (0.2 mm chamfer) are considered for key parts, such as winding conductor in this investigation to solve the above phenomenon. The curvature factor of the mesh set at the chamfer is 0.15, which meets the requirements of simulation accuracy. The specific grid division settings are as follows: the maximum grid element at the conductor part is 0.5 mm, the minimum is 0.002 mm, the cell growth rate is 1.1 and the curvature factor is 0.15. In other parts, the maximum grid cell is 4.3 mm, the minimum is 0.0086 mm, the cell growth rate is 1.1 and the curvature factor is 0.2. The final number of grid cells is 302,533, and the statistics of grid cell quality are as follows: the grid quality is generally good, and the average grid quality is 0.8182, to meet the electric field simulation of the 2D axisymmetric model (). The mesh division is shown in .
Figure 1. The structure of winding structure of power transformer and grid generation of two-dimensional finite element model.

Table 1. Simulation parameter setting of oil-impregnated paper and copper (Gao, Citation2022).
3. Results and discussion
3.1. Flow velocity distribution of vegetable and mineral oil power transformer
The transformer adopts forced oil circulation for cooling. The internal heat source of the transformer mainly includes core loss and winding loss. The core loss is calculated using an analytical formula, and it is assumed that the core loss distribution is uniform. Using the winding loss per pie obtained in the magnetic field as the heat source of the winding can more accurately reflect the non-uniformity of the distribution of the heat source along the axial direction of the winding, thereby more accurately obtaining the temperature rise and location of the winding hot spot.
The flow velocity distribution of FR3 vegetable oil in the low-voltage winding at the inlet velocity of 0.1, 0.3 and 0.6 m/s is shown in . It can be seen that the increase of the initial flow rate will lead to the increase of the flow rate of the insulating oil in the low-voltage winding, which indicates that the structural design of the low-voltage winding can meet the flow requirements of the insulating oil under various flow rates. In addition, because the insulating oil in the DC channel is easier to flow, the flow rate of the insulating oil in the DC channel is higher after it enters the low-voltage winding from the bottom inlet, and the flow rate of the insulating oil in the oil flow channel on the left side of the inlet is relatively low, which makes the temperature of the oil flow channel on the right side of the low-voltage winding higher than the temperature on the left side. However, because the oil flow channel inside the low-voltage winding is similar to the S-shaped distribution, the temperature of the insulating oil on the side wall of the low-voltage winding is higher than that of other parts. In addition, because the insulating oil is an incompressible liquid, the flow rate of the insulating oil in the upper and lower structures of the low-voltage winding is not significantly different. shows the velocity distribution of mineral oil in the low-voltage winding at the inlet velocity of 0.1, 0.3 and 0.6 m/s. It can be seen that the flow rate of mineral oil is greater than that of vegetable oil at the same flow rate, because the viscosity of mineral oil is smaller. In addition, the flow velocity distribution in mineral oil is roughly the same as that of vegetable oil, and its flow velocity on the side wall of low-voltage winding is the largest. Our calculation results are consistent with Zhang’s work (Thirugnanam, Siluvairaj, & Karthik, et al., Citation2017; Thirugnanam, Siluvairaj, & Radha, et al., Citation2017), the presence of guide baffles can better cool the heat inside the windings, but also limits the flow rate of insulating oil to a certain extent.
3.2. Temperature distribution of vegetable and mineral oil power transformer
The temperature distribution of FR3 vegetable oil in the low-voltage winding at the inlet velocity of 0.1, 0.3 and 0.6 m/s is shown in . shows that the higher the oil flow rate, the lower the maximum temperature of the insulating oil in the low-voltage winding, which indicates that the insulating oil in the low-voltage winding can effectively reduce the heat generated by the transformer. In addition, . It can be seen that the temperature of the upper part of the LV winding is higher than that of the lower part of the LV winding. This is because the insulating oil has less heat from the metal wall after entering the oil flow channel. With the increasing of the flow stroke of the insulating oil, the energy absorbed from the metal wall increases, and the temperature of the insulating oil rises. This also shows that the structural design of the LV winding can effectively reduce the temperature of the LV winding. shows the temperature distribution of mineral insulating oil in the low-voltage winding at the inlet velocity of 0.1, 0.3 and 0.6 m/s. The results show that the maximum temperature of mineral oil at the initial flow rate of 0.1 m/s is 420 K, and the temperature at the upper end is much higher than that of the winding at the lower end. This is because the mineral oil continuously absorbs its internal temperature after entering the winding, resulting in the highest temperature of mineral oil at the winding outlet. The maximum temperature in the winding decreases with the increase of the initial flow rate, and the maximum temperature of the winding at the same flow rate is smaller, which indicates that the heat transfer capacity of mineral oil is better. shows the temperature distribution curve of the low-voltage winding in the Z-axis direction under different flow rates. The results show that the temperature of vegetable oil is higher than that of mineral oil at different flow rates, mainly due to the higher viscosity of vegetable oil. In addition, the trend of high temperature at both ends of the low-voltage winding and low temperature at the middle is consistent with the temperature distribution in and .
Figure 4. Temperature distribution of FR3 vegetable oil in low-voltage winding at different flow rates.

3.3. Pressure distribution of vegetable and mineral oil power transformer
The pressure distribution of FR3 vegetable oil and mineral oil in the low-voltage winding at the inlet velocity of 0.1, 0.3 and 0.6 m/s is shown in . It can be seen from that the increase of FR3 insulating oil flow rate will lead to the increase of insulating oil pressure. In addition, the bottom pressure of the low-voltage winding is higher than the upper pressure of the low-voltage winding, which is caused by the fact that the bottom insulating oil needs to push the upper insulating oil to flow when the insulating oil is just injected into the low-voltage winding. In addition, by comparing the pressure distribution of FR3 vegetable oil and mineral oil, it is found that the pressure of vegetable oil is higher because the viscosity of mineral oil is lower and the fluidity is stronger. shows the pressure distribution of FR3 vegetable oil and mineral oil in low-voltage winding at 20 °C, 50 °C and 80 °C. The results show that the maximum pressure in FR3 insulating oil and mineral oil increases with the increase of temperature.
3.4. Electric field distribution of transformer winding
The distribution of electric field in LV, MV and HV windings of vegetable oil and mineral oil transformers is studied, as shown in . The HV winding is 110 kV AC voltage, the MV winding is 35 kV AC voltage and the LV winding is 10.5 kV AC voltage. The results show that the maximum electric field strength in the winding of vegetable oil transformer is 4.3 × 106 V/m, maximum electric field strength in mineral oil transformer winding is 4.4 × 106 V/m. In addition, the largest power plant in LV, MV and HV windings of vegetable oil transformer is 4.3 × 106 V/m, 3.5 × 106 V/m and 4.1 × 106 V/m, respectively, the maximum electric field in LV, MV and HV windings of mineral oil transformer is 4.3 × 106 V/m, 4.1 × 106 V/m and 4.4 × 106 V/m. Note that the maximum electric field strength of the LV winding in the vegetable oil transformer is in the middle of the LV winding, the maximum electric field strength of the LV winding in the mineral oil transformer is at its bottom, and the maximum electric field strength of the other windings is in the same position.
4. Conclusion
Based on the FEM, this article calculates and studies the oil flow distribution, temperature distribution and electric field distribution of FR3 vegetable oil and mineral oil in the low-voltage winding of the true-type transformer. The summary is as follows:
At the inlet velocity of 0.1, 0.3 and 0.6 m/s, the maximum flow velocity of FR3 vegetable oil and mineral oil are all on the side wall of low-voltage winding, and the maximum flow velocity of mineral oil is higher.
The increase of the inlet flow rate will also lead to the decrease of the temperature inside the low-voltage winding. Due to the lower viscosity of mineral oil, its heat transfer efficiency is higher.
At the same flow rate, the pressure of mineral oil in the low-voltage winding is smaller, which is also due to the low viscosity and strong fluidity of mineral oil.
The maximum electric field strength of vegetable oil transformers is higher than that of mineral oil transformers, and the maximum electric field strength of both vegetable oil and mineral oil transformers is in the low-voltage winding.
Authorship contribution statement
Suning Liang and Xianjun Shao: Conceptualization, methodology, data analysis, article writing. Zhi Yang: Methodology, data analysis. Lingfeng Jin: Conceptualization, article reviewing. Qiang Wang and Zhengyong Huang: Conceptualization, supervision of the project and article writing and reviewing.
Acknowledgments
The project’s title is High Voltage Large Capacity Natural Ester Vegetable Oil Transformer Key Technology Research.
Disclosure statement
No potential conflict of interest was reported by the author(s).
Additional information
Funding
Notes on contributors
Suning Liang
Suning Liang was born in Jiangsu province, China, in 1991. He received the Ph.D. degree in electrical engineering in 2021 from Chongqing university, Chongqing, China. He is currently an electrical engineer in Electric Power Research Institute of Zhejiang Electric Power Co., Ltd. His research field mainly includes pre-breakdown and breakdown phenomena in liquids, environment-friendly insulation materials, online monitoring and fault diagnosis of HV power equipment.
References
- Chen, W., Zhang, Z. Q., & Zhang, W. (2020). Three dimensional finite element analysis of stray loss in structural parts of power transformers. Computer & Digital Engineering, 48 (11), 2783–2787.
- Gao, C. (2022). Analysis and research on the effect of replacing natural ester on thermal aging characteristics and life extension of oil-paper insulation [D]. Chongqing University.
- Hong, J., Heyun, L., & Zihong, X. (2007). Three-dimensional finite element analysis of end electric field of dry-type transformer. Journal of Guizhou University (Art), 11(02), 47–51.
- Jeong, J., An, J., & Huh, C. (2012). Accelerated aging effects of mineral and vegetable transformer oils on medium voltage power transformers. IEEE Transactions on Dielectrics and Electrical Insulation, 19(1), 156–161.
- Jianxun, L., Shuoming, G., Yinglong, W., Xin, Y., Hongbin, W., & Hongshun, L. (2022). Study on influencing factors of converter transformer temperature field based on finite element method. Electronic Test, (19), 76–78.
- Joo, H. W., Lee, C. H., Rho, J. S., & Jung, H. K. (2006). Analysis of temperature rise for piezoelectric transformer using finite-element method. IEEE Transactions on Ultrasonics, Ferroelectrics, and Frequency Control, 53(8), 1449–1457. https://doi.org/10.1109/tuffc.2006.1665102
- Martins, M. A. G. (2010). Vegetable oils, an alternative to mineral oil for power transformers-experimental study of paper aging in vegetable oil versus mineral oil. IEEE Electrical Insulation Magazine, 26(6), 7–13. https://doi.org/10.1109/MEI.2010.5599974
- Mehta, D. M., Kundu, P., Chowdhury, A., Lakhiani, V. K., & Jhala, A. S. (2016). A review on critical evaluation of natural ester vis-a-vis mineral oil insulating liquid for use in transformers: Part 1. IEEE Transactions on Dielectrics and Electrical Insulation, 23(2), 873–880. https://doi.org/10.1109/TDEI.2015.005370
- Rapp, K. J., & Luksich, J. (2011 Review of Kraft paper/natural ester fluid insulation system aging [Paper presentation]. 2011 IEEE International Conference on Dielectric Liquids, (pp 1–4), Trondheim.
- Samikannu, R., Raj, R. A., Murugesan, S., Venkatachary, S. K., & Stonier, A. A. (2022). Assessing the dielectric performance of Sclerocarya birrea (Marula oil) and mineral oil for eco-friendly power transformer applications. Alexandria Engineering Journal, Tamil Nadu, India, 61(1), 355–366. https://doi.org/10.1016/j.aej.2021.06.002
- Shao, W., Han, Z., Xiang, Y., Li, P., & Cui, Y. (2014). Temperature distribution of piezoelectric transformer with heat couple component using finite element method. 2014 IEEE International Ultrasonics Symposium (pp. 1340–1343).
- Thirugnanam, A. R. P. R., Siluvairaj, W. I. M., & Karthik, R. (2017). Performance studies on dielectric and physical properties of eco‐friendly based natural ester oils using semi‐conductive nanocomposites for power transformer application, Tamil Nadu, India. IET Science, Measurement & Technology, 12(3), 323–327. https://doi.org/10.1049/iet-smt.2017.0467
- Thirugnanam, A. R. P. R., Siluvairaj, W. I. M., & Radha, K. (2017). Retreatment of aged mineral oil using semiconductive nanocomposites for power transformer application. International Transactions on Electrical Energy Systems, 27(9), e2358. https://doi.org/10.1002/etep.2358
- Wang, Y. (2018). Thank you for your suggestion. The reference has been added into the revised manuscript: Study on the discharge-related parameters of triglyceride molecules and vegetable insulating oils based on density functional theory [D]. Chongqing University.