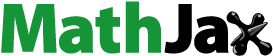
Abstract
Metal-matrix composites (MMCs) and hybrid metal-matrix composites (HMMCs) are novel materials that possess distinctive mechanical characteristics, making them well-suited for industrial applications. This research implements an experimental study of the mechanical properties of HMMCs, where weight percentages (2, 3, and 4 wt. %) of zirconium dioxide (ZrO2) and graphite powder (Gr) are used as reinforcements and the aluminum alloy 7475 (AA7475) is used as a matrix. In this investigation, the stir casting method was used to prepare the composite by using AA7475 alloy with pre-heated reinforced particles of ZrO2 and graphite with various reinforcement weight percentages. Among these combinations, AA with 4 wt. % ZrO2 and 2 wt.% Gr showed enhanced mechanical properties. The mechanical properties of the ultimate tensile strength, yield strength, and hardness were evaluated. A Scanning Electron Microscope analysis was conducted to study the reinforcement distribution in the matrix. The input parameters for wire-cut electrical discharge machining (WCEDM) are pulse-on time (TON), pulse peak current (IP), and flushing pressure (PF). The material removal rate and surface roughness are the output responses. Determining the ideal set of process parameters in WCEDM for HMMC also involved the use of the Taguchi design technique known as the L9 orthogonal array. The peak current for the MRR and flushing pressure for the SR are the most prominent parameters.
1. Introduction
By optimizing their composition, processing, and manufacturing, metal-matrix composites (MMCs) and hybrid metal-matrix composites (HMMCs) can demonstrate enhanced mechanical properties. MMCs and HMMCs offer unique mechanical properties; however, their processing and production are challenging. However, the present MMC and HMMC processing and manufacturing technologies have expensive production costs, and poor mechanical characteristics. These materials can be strengthened with ceramic materials in various ways to improve their mechanical and frictional properties. Reinforcing materials such as alumina, silicon carbide, boron carbide, and aluminum oxide are commonly utilized (Gill et al., Citation2022). The nanocomposites were fabricated by incorporating n-B4C and n-B4C/MoS2 particulates into an Al2219 alloy, matrix using the stir casting technique. To evaluate the even distribution of reinforcement, scanning electron microscopy (SEM) and energy-dispersive X-ray spectroscopy (EDS) are used (Siddesh Kumar et al., Citation2017). Various mechanical properties like yield strength, ultimate strength, hardness, % elongation and compressive strength were assessed in accordance with ASTM standards to assess the attributes of Al-based MMCs (Boppana et al., Citation2020; Karthikeyan et al., Citation2021; Nagaral et al., Citation2019).
An unconventional manufacturing technique known as electrical discharge machining (EDM) is used to produce precise and complicated shapes from materials that are too difficult to manufacture conventionally. EDM produces intricate designs with precise tolerances using electrical discharges to remove material from workpieces (Ming et al., Citation2020). Sinker EDM, sometimes referred to as Ram EDM or conventional EDM, employs electrodes that are similar to the ultimate shape intended for the item to generate an electrical discharge that strikes the workpiece and erodes the material. This process is ideal for creating small, intricate parts with complex shapes. Wire-cut electrical discharge machining (WCEDM), also known as wire EDM, is a type of EDM process that uses a thin electrically charged wire to cut through the workpiece (Gangil et al., Citation2017). The wire is constantly fed through the workpiece, while a spark is generated between the wire and the workpiece, eroding the material. The wire used in wire EDM is typically made of brass or tungsten and is held taut between a spool and a worktable. The power supply generated an electrical discharge that eroded the workpiece as the wire passed through it. WCEDM is a highly precise process that can achieve tolerances up to ±0.0001 in. It can cut through very thick workpieces of up to 20 inches in most cases. WCEDM is often used to create small, intricate parts with tight tolerances, such as gears, nozzles, and medical implants. One of the main advantages of WCEDM is that it does not require direct contact between the cutting tool and the workpiece, which reduces the risk of tool wear and breakage (Hakami et al., Citation2017). This makes it a more efficient process for cutting hard materials, such as titanium and tungsten carbide.
WCEDM is a non-conventional machining process used to machine a wide range of materials, including MMCs and HMMCs. MMCs are composite materials made up of a metal matrix, such as aluminum, reinforced with ceramic or other materials, whereas HMMCs combine a metal matrix with organic or inorganic fibers (Doreswamy et al., Citation2021). The need to produce extremely precise and intricate parts that are impossible to manufacture using conventional machining processes drives the use of WCEDM in MMCs and HMMCs. WCEDM can produce complex geometries and tight tolerances, making it an ideal process for producing MMCs and HMMCs (Kavimani et al., Citation2019). The main difference between MMCs and HMMCs is the nature of the reinforcing materials. MMCs are reinforced with ceramics, whereas HMMCs are reinforced with fibers, such as carbon or glass. The addition of fibers to the metal matrix in HMMCs results in a composite material that is stronger and more resistant to deformation than MMCs (Dar et al., Citation2022). This makes HMMCs ideal for high-performance applications such as aerospace, automotive, and defense. However, the use of WCEDM in HMMCs presents several challenges (Gavisiddesha et al., Citation2022). Mathematical regression models were used to estimate the ultimate tensile strength and tensile elongation of custom-welded joints (Battina et al., Citation2021). The stir casting method was used to manufacture the HMMC AA6061 matrix, which is reinforced with nano ZrO2, graphene and graphite of particulates. Reinforced nanoparticles improved mechanical properties like yield strength, ultimate tensile strength, and hardness when compared to the base metal (Pandiyarajan et al., Citation2022). Owing to the presence of fibers, composite materials are brittle and prone to fracture when cut, making the workpiece brittle, and difficult to handle. Furthermore, fibers have a higher propensity to harm the wire electrodes. Several strategies can be used to overcome these challenges. One approach is to use a wire electrode made of a harder material, such as tungsten carbide, which can withstand the increased wear of machined HMMCs. Another approach is to use a combination of WCEDM and other machining processes, such as grinding or milling, to reduce the amount of material that must be removed by WCEDM.
1.1. Working principle of WCEDM
The workpiece was placed close to a wire electrode, which was used to flush away the cutting waste using dielectric fluid (typically designated E). Sparks that melt and vaporize the material are produced when the wire electrode meets the workpiece, thereby creating a discharge. According to HMMC, because there are fibers in the composite material, the workpiece is fragile and prone to cracking when cut. Furthermore, fibers have a higher propensity to harm wire electrodes, which would result in a costlier downtime. To overcome these challenges, the working parameters of WCEDM must be optimized for HMMCs. This involves adjusting the electrical discharge parameters, such as the pulse duration and current intensity, to ensure that the wire electrode does not break owing to the presence of fibers in the workpiece. Additionally, the use of a specialized wire electrode made of a harder material, such as tungsten carbide, can help reduce wear and breakage during the cutting process. The use of a suitable wire electrode is also important for WCEDM of HMMCs. They should be able to flush away the debris generated during the cutting process and provide sufficient insulation to prevent arcing between the wire electrode and workpiece. The sketch below illustrates the working principle of WCEDM, as shown in .
The components of the WCEDM system include a power supply, wire electrode, workpiece, and control unit. The power supply generates a high voltage that is used to create an electrical discharge between the wire electrode and the workpiece. The wire electrode is fed through the workpiece to create a narrow cut or kerf in the material. Dielectric fluid was used to flush away the debris generated during the cutting process and prevent the formation of cracks in the workpiece. The control unit regulates the speed and direction of the wire electrode and the intensity and duration of the electrical discharge, and the flow rate of these parameters can be adjusted to produce highly accurate cuts with complex shapes and features. The power supply was connected to the wire electrode and the workpiece through a series of cables and connectors. A wire electrode is typically made of a thin wire of metal, such as brass or tungsten, and is fed through the workpiece using a wire guide. The workpiece was clamped in place using a special fixture, which allowed precise positioning and alignment. It was circulated through the system using a pump and a series of tubes and nozzles. Fluid is typically a specialized type of oil or water that has been used to improve its insulating properties and prevent corrosion of the components.
1.2. Input process parameters
The WCEDM input process parameters have a significant impact on how well the process runs and how well the surface of the object is machined. Below are the major input process parameters for WCEDM and their significance in the machining process.
1.2.1. Pulse duration
Pulse duration is the time interval at which electrical discharge occurs between the wire electrode and workpiece. This affects the size and shape of the craters produced on the workpiece surface. According to a study (Almeida et al., Citation2021), shorter pulse durations result in smaller craters and smoother surface finishes, but at the cost of reduced cutting speeds. Conversely, longer pulse durations resulted in larger craters, rougher surface finishes, and higher cutting speeds.
1.2.2. Pulse frequency
Pulse frequency is the number of electrical discharges per unit of time. This affects the material removal rate and the surface finish. Although surface polishing is compromised, higher pulse frequencies accelerate material removal. Lower pulse frequencies lead to a smoother surface finish but decrease the material removal rate. A study by Ming et al. (Citation2021) found that the optimal pulse frequency varied depending on the workpiece material, with higher pulse frequencies producing better surface finishes on stainless steel, and lower pulse frequencies producing better surface finishes on titanium alloy.
1.2.3. Peak current
The peak current is the maximum current flowing between the wire electrode and workpiece during electrical discharge. This affected the material removal rate, surface finish, and electrode wear. A higher peak current improves the surface finish but decreases the material removal rate and electrode degradation. A study by Slătineanu et al. (Citation2020) found that higher peak currents resulted in faster material removal rates but lower surface finishes, whereas lower peak currents resulted in slower material removal rates but higher surface finishes.
1.2.4. Wire tension
The wire tension is the tension applied to the wire electrode during the machining process. This affects the straightness and stability of the wire electrode and the surface finish. A higher wire tension leads to higher material removal rates and a rougher surface finish. A lower wire tension leads to a smoother surface finish but a lower material removal rate. A study by Gupta et al. (Citation2020) found that higher wire tensions resulted in higher material removal rates but poorer surface finishes on a titanium alloy workpiece.
1.2.5. Wire speed
The wire speed is the speed at which the wire electrode moves through the workpiece during machining. This affects the MRR and the surface finish. Higher wire speeds led to higher MRR and rougher surface finishes. Lower wire speeds led to a smoother surface finish but lower material removal rates. A study by Prakash et al. (Citation2019) found that lower wire speeds resulted in a better surface finish on a copper workpiece, while higher wire speeds resulted in faster material removal rates.
1.2.6. Dielectric fluid (

Dielectric fluid is used to cool and flush away the debris generated during the machining process. This affects electrode wear, surface finish, and cutting speed. The type and flow rate of the dielectric fluid affects the quality of the machined surface and electrode wear. According to the findings of Gore and Patil (Citation2018), the best surface finish on titanium alloy workpieces was obtained when a deionized water dielectric fluid was used at a flow rate of 7 L/min.
1.3. Contributions
The main contributions of this research are:
Aluminum alloy 7475 (AA7475) is a prominent alloy that can be heat-treated and used as a matrix, and zirconia (ZrO2) and graphite powder (Gr) were used as reinforcements in this study.
Identification of Process Inputs and Output Characteristics: This study identifies process inputs such as TON, PF, Ip, wire material, and workpiece material that affect the machining process of HMMCs. Output characteristics, such as yield strength, surface roughness, ultimate tensile strength, and hardness, were used to evaluate the quality of the machined components.
In this study, the ideal combination of process parameters in WCEDM for HMMC was determined using the Taguchi design technique, L9 orthogonal array (OA). The number of experiments required to find the ideal set of process parameters is decreased using this method, which also aids in the optimization of the machining process.
This article’s body is what follows. The latest literature review on WCEDM, as well as its drawbacks and knowledge gaps, are discussed in Section 2. In addition, the problem statement has been described. Section 3 explains the materials and methods and their significance in WCEDM, along with the methodologies employed for sample preparation and process optimization. Sections 4 and 5 describe the preparation of the composites and the experimental setup. Section 6 illustrates the results obtained from the WCEDM and Taguchi methods, followed by Section 7, which provides the overall conclusions of the research.
2. Literature survey
To create B4C- and SiC-reinforced plates in AZ61 magnesium alloy by stir casting, Sathish et al. (Citation2021) used various ratios of the materials. The enrichment ratio, time, speed of stirring, and set temperature were among varied process parameters. To obtain the material removal rate (MRR) and surface roughness (SR) values, samples from composites cast with AZ61 alloy were obtained and processed using WCEDM. In this study, the MRR and SR were calculated using L16 OA, and the maximum UTS and minimum hardness were estimated using Taguchi’s approach. Chockalingam et al. (Citation2019) used grey connections and statistical analysis to construct an empirical relationship that explains the connection between processability and control factors. The proven empirical links are advantageous for both users and machine-makers. In their study, Divya et al. (Citation2020) focused on the optimization of WCEDM processing parameters using a variety of methods, including the Taguchi method, ANOVA, grey relation analysis (GRA), artificial neural network (ANN), and principal component analysis (PCA). To attain the highest MRR and lowest SR, they employed the Taguchi method to optimize parameters such as TOFF, TON, VS, IP, and kerf width.
The MRR and SR of the INCONEL 625 alloy were examined using the Taguchi technique by Subrahmanyam and Nancharaiah (Citation2020) in their analysis of the impact of the WCEDM process parameters. To obtain the highest MRR and lowest SR, the authors optimized several parameters using MINITAB 17, including TOFF, TON, and servo voltage (SV). The WCEDM technique has been used to manufacture materials such as MMC, ceramic composites, and superalloys, according to a review by Praveen et al. (Citation2020). Using various methodologies, they attempted to improve the WCEDM processing parameters and replies. Kannan et al. (Citation2022) To determine the best parameter settings for enhancing two processing features, we used the TOPSIS approach for ideal solutions and a similarity-first method. The TOPSIS method was used to determine the best settings: 4 A spark current, 20 s pulse width, 72 s pulse interval, and an 18 mL/min mixing water flow rate.
Titanium nitride (TiN), generated by cutting microwave sintering and reinforced with chromium composite, was the subject of a study by Girisa et al. (2021). A teaching-learning-based optimization strategy was suggested by Devarasiddappa and Chandrasekaran (Citation2020) for preference-based multi-objective optimization. The MRR increased by 40.51% because of the optimization, whereas the SR decreased by 15.66%. On the MRR of stainless steel 304 material utilizing the WCEDM process, Seshaiah et al. (Citation2022) examined the impacts of several operational variables, including wire speed, wire tension, discharge current, dielectric current rate, pulse on time, and pulse off time. They employed RSM to optimize the process factors to maximize the MRR and produce a good surface finish. Similarly, Karthikeyan et al. (Citation2022) created a method using artificial intelligence to optimize network weights for maximal MRR and minimal SR. Taguchi’s design was employed by Babu et al. (Citation2021) to identify the critical process variables, particularly the material removal rate and surface finish, that affect the performance of WCEDM. To examine the connection between the input treatment parameters and MRR, they employed ANOVA findings and plots of the main and interaction effects. Battina et al. (Citation2022) carried out various combinations of different sheets utilized for experimental and finite element simulation tests to evaluate the maximum dome height. Chekuri et al. (Citation2022) conducted a study to evaluate the precision of results derived from numerical analysis. The theoretically calculated material removal rate (T-MRR) was compared to the experimentally determined material removal rate (E-MRR) considering input process variables like gap voltage, peak current, and pulse on time. Dayakar et al. (Citation2019) The Taguchi optimization approach was suggested for wire electrical discharge machining (WEDM) of maraging steel 350, with material removal rate (MRR) and surface roughness (Ra) as the output answers. The researchers Naidu et al. (Citation2021) and Palanisamy et al. (Citation2020) investigated the wire-cut electrical discharge machining of aluminum-metal matrix composite (Al-MMC) consisting of Al2024 alloy and Al2O3. The input process parameters analyzed in this study are the pulse on time “Pon” (measured in seconds), the pulse off time 'Poff’ (also measured in seconds), and the peak current (measured in amperes). An investigation was conducted utilizing Taguchi’s analysis to assess the impact of process parameters on desired outputs, namely material removal rate (MRR) and surface roughness (SR). Molybdenum was used as the wire material (electrode).
2.1. Research gap
The processability of various materials has been examined in earlier studies about the process parameters; however, HMMC as a working material has not received sufficient attention. Furthermore, there is limited research on the optimization of multiple response characteristics, including the YS, UTS, hardness, SR, and MRR of HMMCs using the Taguchi method.
2.2. Problem statement
The research gap mentioned above emphasizes the need for experimental investigations to examine the impact of process parameters on various WCEDM and HMMC response qualities. To attain the highest possible YS, UTS, hardness, lowest possible SR, and highest possible MRR, this study sought to optimize the process parameters, including the TON, PF, IP, wire, and workpiece material. The challenge is to use Taguchi’s method to identify the ideal set of WCEDM process parameters in HMMC to enhance processing efficiency.
3. Materials and methods
3.1. Matrix material AA7475
AA7475 is a high-strength alloy consisting mostly of aluminum (Al), with the addition of elements like zinc, copper, magnesium, and chromium to improve its characteristics. The utilization of this alloy is frequent in aerospace applications due to its exceptional strength-to-weight ratio and remarkable fatigue resistance. aluminum alloy with the chemical formula AA7475 and the weight percentages of its constituent parts are listed in . AA7475 has 0.20% copper (Cu) by weight, which is a frequent addition to aluminum alloys that makes them stronger, harder, and more corrosion resistant. Furthermore, it helps to make the alloy more heat treatable. Additional elements added to aluminum alloys to improve workability and strength include manganese (Mn), which constitutes 0.30% of the weight of AA7475. This can help refine the grain structure and enhance the reaction of the alloy to heat treatment. There was 2.4% magnesium (Mg) by weight in AA7475. Magnesium is an essential alloying ingredient for aluminum alloys because of its substantial strength enhancement. The proportion of Si by weight in AA7475 was 0.40%. Silicon is a typical additive used to enhance the casting properties of aluminum alloys. This improved the castability, fluidity, and melting point of the alloy. On a weight basis, AA7475 contained 6% Zn. Zinc was added to the aluminum alloys as a reinforcing ingredient. However, chromium makes objects more resistant to wear and corrosion. The proportion of Ti in AA7475 by weight was 0.20%. The mechanical properties of the alloy can be improved by controlling its grain size and distribution. By weight, AA7475 contained 0.50% iron. Iron is often seen as an impurity in aluminum alloys because it can negatively impact their mechanical characteristics when present in high concentrations. The material is procured from alloy pipe impex Mumbai, Maharashtra.
Table 1. Composition of AA7475 by weight percentage Elements.
3.2. Reinforcement (Graphite and ZrO2)
3.2.1. Graphite powder
Graphite powder, which has an Irregular shape of 60 microns, is frequently utilized as a reinforcing agent for HMMCs purchased from Sigma-Aldrich. It possesses several distinctive characteristics that render it an exceptional option for this application. Graphite powder is characterized by its notable electrical conductivity with a density of 2.267 g/cm³. (a), shows the morphology of graphite powder. It has a high melting point and is chemically stable, making it suitable for use in high-temperature and corrosive environments.
3.2.2. ZrO2
Zirconium dioxide (ZrO2), commonly referred to as zirconia, is a ceramic substance with an irregular shape of 20–50 microns, as shown in (b). It is frequently employed as a reinforcing agent in HMMCs. It possesses many distinctive characteristics that makes it as a very efficient reinforcing material for HMMCs. The remarkable properties of ZrO2 includes its high strength and toughness, which make it an ideal substance for improving the mechanical properties of HMMCs. Zirconia has a density of 5.89 g/cm3. ZrO2-reinforced HMMCs have the capability to be employed in many high-temperature applications, such as aerospace, automotive, and other industrial sectors.
3.3. Stir casting procedure
Stir casting is a widely employed method for producing hybrid metal matrix composites (HMMCs) by adding reinforcing elements to a molten metal matrix. The reinforcing elements were incorporated into the molten metal by the use of a mechanical stirrer, and the resultant blend was subsequently poured into the required form and left to harden. AA 7475 is a widely utilized high-strength aluminum alloy in HMMCs, serving as a matrix material. Graphite powder and ZrO2 are commonly utilized as reinforcing elements alongside AA7475 in stir-casting methods, as seen in . Graphite powder is a kind of carbon that has strong electrical and thermal conductivity, a low friction coefficient, and exceptional resistance to wear. Incorporating AA7475 into the HMMC can enhance its mechanical characteristics, including strength, stiffness, and wear resistance. Moreover, the use of graphite powder can enhance the workability of HMMC. Conversely, ZrO2 is a ceramic substance characterized by its exceptional strength, hardness, and thermal stability. ZrO2, when included in AA7475, enhances the mechanical and thermal characteristics of HMMC, including tensile strength, hardness, and high-temperature resistance.
Three distinct hybrid metal matrix composites (HMMCs) were fabricated: HMMC 1 included 2 wt. % ZrO2 and 4 wt. % Gr; HMMC 2 contained 3 wt. % ZrO2 and 3 wt. % Gr; and HMMC 3 contained 4 wt. % ZrO2 and 2 wt. % Gr.
3.4. Mechanical properties
YS, UTS, hardness, MRR, and SR are important mechanical properties commonly used to describe the strength and durability of materials. These properties can be measured using various testing methods, and can provide valuable information for the design, manufacturing, and selection of materials for various applications.
YS: This indicates the amount of stress that a material can bear before permanent deformation. the point at which a substance transitions from elastic to plastic deformation. In a tensile test, a sample of material is often subjected to escalating stress until it starts to irreversibly deform, which is the typical assessment of yield strength.
UTS: The highest stress a material can withstand prior to tensile failure is known as UTS, or simple tensile strength. Additionally, tensile testing is used, and the results are typically expressed in megapascals (MPa) or pounds per square inch (psi), which expresses the force per unit area.
Hardness refers to the resistance of a material to indentation, scratching, or deformation. This is a measure of how easily a material can be scratched, dented, or worn away. Hardness is typically measured using a hardness tester that applies a known amount of force to a small area of the material and measures the depth or size of the resulting indentation.
3.5. Taguchi method
The Taguchi method is a widely adopted technique for optimizing WCEDM process parameters to achieve optimal machining results with fewer experimental runs. This method involves the use of an OA to conduct a series of experiments with varying process parameters to identify the optimal combination of parameters that can minimize variability in the machining process. To optimize the TON, PF, and IP for the WCEDM process parameters using the Taguchi-based design of experiments, the following steps are typically taken:
Step 1: Determine the range of values for each of the three process parameters that need to be optimized.
Step 2: Develop an OA with an appropriate number of experimental runs, based on the number of levels and factors being investigated. For example, a standard L9 orthogonal array can be used to investigate three factors with three levels each, requiring nine experimental runs.
Step 3: Give each experimental run a unique set of TON, PF, and IP values.
Step 4: Conduct tests to gather information regarding the response, such as the rate of material removal, surface roughness, and tool wear.
Step 5: Determine the best configuration of process parameters to reduce process variability by analyzing the data using signal-to-noise (S/N) ratios.
Step 6: Verify the results obtained from the analysis by conducting confirmation experiments.
By adopting the Taguchi method to optimize the WCEDM process parameters, the number of experimental runs required to identify the optimal combination of parameters can be reduced. Additionally, this approach can lead to improvements in the machining performance and increased productivity.
4. Composite preparation
The stir casting process for AA7475 with graphite powder (Gr) and ZrO2 as reinforcement materials starts with the melting of the matrix material AA7475 in the induction furnace, and a temperature of 700 °C is maintained. Both the reinforcements were weighed and pre-heated separately before they were added to the matrix. The purpose of the preheating process is to remove the moisture content in reinforcement particles.
At a temperature of 700 °C, 4 wt. % of ZrO2 and 2 wt. % of Gr, is introduced into the induction furnace. Subsequently, a Mg 1.0 wt. % is added to facilitate the removal of oxides and other impurities from the metal. For generating a vortex, a four-blade stirrer was inserted into the crucible, and its rotational 500 rpm speed was finely tuned using a potentiometer. Before enhancing the vortex, the rotating speed of the crucible was stabilized. After the addition of all the reinforcements, the stirrer was left to rotate for 10 min to disperse the particles uniformly. The metal required approximately 2 min to cool and harden in the die cavity after being ladled from the crucible. Samples of the metal shards were collected from the crucible. ZrO2 and Gr samples with compositions of 2 wt. % ZrO2 and 4 wt.% Gr or 3 wt.% ZrO2 and 3 wt.% Gr are created according to specifications. The final samples were sorted, labeled, and sent to WCEDM for further processing.
5. Experimentation
show that the ELEKTRA SPRINTCUT 734 WCEDM machine was used in studies at Sree Datta CNC WCEDM in Hyderabad, India for determining the effect of process factors on surface smoothness during WCEDM. The goal of this project is to ascertain how TON, PF, IP, and wire affect the MRR and SR of stir casting composites with AA7475 as the matrix material and Gr and ZrO2 as reinforcements. As the process settings were changed, experiments were conducted, and the MRR and SR values of the machined surface were recorded.
shows the technical specifications of the machine tools and the pulse generator specifications. The primary objective of this investigation was to determine the optimal settings for each parameter to obtain the desired surface finish and material removal rate. It is essential to investigate the effects of each parameter separately and the interactions between the parameters. Thus, the effect of each parameter can be better understood, and the optimal values can be determined. This knowledge can be used to improve the machining process and achieve the desired surface finish.
Table 2. Technical specifications of machine tool and Pulse generator.
5.1. Surface roughness (SR)
This refers to the surface texture and unevenness of a material. It is a way to gauge how smooth or rough a surface is, and it depends on a few factors, including the way it was made, the tools that were used, and the makeup of the materials. (a) shows the SR analysis of the texture of the machined surface using Mitutoyo SJ-210.
(b) shows the machined workpieces obtained using WCEDM. To measure the change in surface height over a specific distance, a profilometer is often used to determine the surface roughness.
Material removal rate (MRR) refers to the volume of material removed per unit time during the machining process. It is a measure of the efficiency of material removal and is typically expressed in cubic millimeters per minute (mm³/min) as shown in EquationEq. (1)(1)
(1) .
(1)
(1)
Here represents the initial and final weights of the workpiece (in g),
represents the density of the workpiece material (2.5668 g/cm3),
and represent the machining time (in min). MRR was determined by weight loss criteria with the help of a digital weighing balance with an accuracy of 0.0001 g. Weight differences before and after the experiment were calculated (Karthikeyan et al., Citation2022).
5.2. Process parameter design
lists the process parameters used in the experimental process. The three significant elements were TON, PF, and IP. The ideal values for the WCEDM process parameters were determined through a three-tier evaluation of each factor. The Taguchi design was employed to select the experimental parameters, and OA was used to create a matrix with three degrees of control for each of the three controllable components. The L9 OA presents the results of nine tests carried out using various combinations of the three input variables. It is worth noting The Taguchi method has been widely utilized to optimize process parameters in the manufacturing industry.
Table 3. Design of experiments for WCEDM process.
Using these techniques, the number of experiments required can be decreased by identifying the best set of process parameters to obtain the desired outcomes. Influencing machining factors, such as PF, IP, and TON, were considered during the experiment. Here, PF was varied from 70 to 90 kg/cm2, TON was varied from 100 to 110 s, and IP was assumed to be 100, 120, and 140 A. To maintain consistency, the servo voltage, wire feed, and tension were kept constant. Using Minitab software, the TAGUCHI method and L9 OA were optimized. In this study, the Taguchi design with an L9 OA was used to select the experimental parameters, which enabled us to identify the optimal values for the process parameters with minimal experimentation.
6. Results and discussion
6.1. Tensile test
Samples made using AA7475 alloy with different compositions, namely AA7475, HMMCs 1, HMMCs 2, and HMMCs 3, were evaluated using a universal testing machine (UTM) to determine their mechanical properties (Naidu et al., Citation2021). The UTM follows a general procedure for calculating the mechanical characteristics:
Sample preparation: The samples were prepared according to ASTM E8 standards with AA7475 alloy, Gr, and ZrO2, according to the desired shape and dimensions.
Mounting the sample: Each prepared sample was securely mounted onto the UTM using appropriate grips or fixtures to ensure stability during testing.
Initial setup: Configure the UTM with the necessary parameters, such as a suitable load cell, crosshead speed, and gauge length for elongation measurement. These settings may vary depending on the specific testing standards and requirements.
YS determination: An increasing load was gradually applied to each sample using the UTM until it reached a point where a permanent deformation of 0.2% (or a predetermined value) occurred. The load was recorded as the yield strength of the material.
UTS testing: Continue applying an increasing load using the UTM until each sample fractures. The loads at the fracture point were recorded as the ultimate tensile strength.
By following these steps for each sample, the UTM can provide data on the mechanical properties, as listed in , including the YS and UTS for all the samples.
Table 4. The tensile test results with different composition.
As listed in , four compositions were used in the tensile test. The YS and UTS values for each composition are recorded in N/mm2. The results demonstrate that the HMMCs 3 exhibits the greatest Ultimate Tensile Strength value of 320 N/mm2, whereas the highest yield strength value of 150 N/mm2 is achieved for HMMCs 1 (Madhavarao et al., Citation2024). shows the tensile strength of different compositions.
6.2. Hardness test
Rockwell hardness testing is a widely used technique for determining the hardness value of materials. The Rockwell hardness testing machine initiated the process by applying a minor load to establish the zero position, following which a major load was applied to measure the depth of penetration. In the present study, the Rockwell hardness testing machine was utilized to assess the hardness of four samples. To ensure accurate testing, samples were subjected to metallographic finishing. This involves utilizing emery paper with varying grits, such as 100, 220, 400, 600, and 1000. By progressively employing these different grits, the surface underwent refinement, eliminating any roughness and achieving a smooth finish.
The samples are prepared as per ASTM E18-08 standards and are placed in a Rockwell hardness tester. A load of 200 g was applied commencing with a minor load to establish the starting position. Subsequently, a major load was applied to measure hardness. This major load induced indentation on the surface of the sample. Each load was maintained for 20 s to ensure precision. Subsequently, the penetration depth was measured, and the Rockwell hardness value was determined using a specific scale associated with the Rockwell hardness testing method. It is crucial to acknowledge that the composition of the samples, particularly the presence of Gr and ZrO2, affects their hardness. The Rockwell hardness testing machine provides a quantitative measurement of the hardness, facilitating the evaluation of the material’s resistance to deformation and its suitability for various applications.
The Rockwell hardness test was performed on three samples with different compositions. The hardness values are listed in . This allowed us to compare the relative hardness of the samples and determine how the addition of Gr and ZrO2 changed the hardness of the material.
Table 5. The hardness test results with different composition.
The results in show that HMMCs 3 has the highest hardness value of 100.6, while HMMCs 2 has the lowest hardness value of 96.83 (Madhavarao et al., Citation2024).
Overall, and demonstrate the influence of varying amounts of Gr and ZrO2 reinforcement materials on the UTS and hardness of AA7475 aluminum alloy. The obtained findings may be utilized to determine the most suitable composition for certain applications, considering the desired mechanical properties. shows the hardness of different compositions.
6.3. Microstructural characterizations
To estimate the distribution range of the reinforcements in the matrix Scanning Electron Microscope analysis is performed as shown in . HMMCs 3 exhibited a better distribution of reinforcements than the other samples. Hence, HMMCs 3 are expected to exhibit high strength owing to the dispersion-strengthening mechanism (Madhavarao et al., Citation2024).
6.4. Surface roughness test (SR)
lists the obtained SR and MRR values for the design of experiments in the WCEDM process of the final workpiece prepared using the stir casting process (discussed in Section 4). appears to show the results of a Taguchi design of experiments for the WCEDM process parameters (Naidu et al., Citation2021). This table shows the data: level, TON, IP, PF, delta, and rank.
Table 6. Obtained SR and MRR values for design of experiments in WCEDM process of AA7475 with Gr and ZrO2.
Table 7. Response table for S/N ratios.
The first column indicates the level of each parameter. In this case, there are three levels for each parameter, numbered 1–3. The second, third, and fourth columns present the actual values used for each parameter at each level (Singuru et al., Citation2024). The difference between the highest and lowest values of each parameter is shown in the delta column. The parameters that had the greatest impact on the outcomes were determined using this difference.
The rank column displays the order of importance of the impact of each parameter on the outcome. Following PF and TON, IP had the greatest effect. The impact of the S/N plot on the SRs in is shown in for these values.
6.5. Material removal rate (MRR)
The first column indicates the level of each parameter. In this case, there are three levels for each parameter, numbered 1–3. The second, third, and fourth columns present the actual values used for each parameter at each level. The difference between the highest and lowest values of each parameter is shown in the delta column. The parameters that had the greatest impact on the outcomes were determined using this difference (Naidu et al., Citation2021). The rank column displays the order of importance of the impact of each parameter on the outcome. Following PF and TON, IP had the greatest effect. The impact of the S/N plot on the MRR in is shown in for these values (Singuru et al., Citation2024).
Table 8. Response table for S/N ratios.
7. Conclusions
The HMMC samples were studied to investigate their unique machining characteristics. Among the three combinations, HMMCs 3 exhibits the greatest ultimate tensile strength value of 320 N/mm2, whereas the highest yield strength value of 150 N/mm2 is achieved for HMMCs 1 and outperformed the remaining samples.
Machining was performed using HMMCs 3. Machining parameters such as PF, IP, and TON, were considered during the experiment. It was discovered that 100 for TON, 70 kg/cm2 for PF, and 140 A for IP are the optimal input parameters for the surface finish. The highest MRR was achieved with a 105 µs TON, 70 kg/cm2 PF, and IP of 140 A. The findings of this study can be helpful for improving the machining performance and productivity of HMMC samples. Future research should focus on exploring the effectiveness of other reinforcing materials on the performance of HMMCs in WCEDM. Additionally, the impact of using different combinations of process inputs such as wire material, workpiece material, TON, PF, and IP, can be investigated.
Moreover, future research can explore the use of advanced optimization techniques, such as artificial intelligence, machine learning, and genetic algorithms, to determine the optimal combination of process parameters. This can help to reduce the number of experiments required to obtain the best-suited process parameters and improve the accuracy of the results. Furthermore, the impact of WCEDM on the mechanical properties of the HMMCs was explored. This can help understand the full potential of WCEDM for the machining of HMMCs and aid in the development of new materials with improved mechanical properties.
Author contributions
SM: conception of the work, data collection, data analysis and interpretation, drafting of the article, and final approval of the version to be published. VVSKR: conception of the work, Critical revision of the article. RBRC and TB: conception of the work, critical revision of the article, analysis, and interpretation of the data. DVN and RDN: critical revision of the article and final approval of the version to be published.
Disclosure statement
No potential conflict of interest was reported by the author.
Data availability statement
The data that support the findings of this study are available from the corresponding author, (Singuru Madhavarao) upon reasonable request.
References
- Almeida, S., Mo, J., Bil, C., Ding, S., & Wang, X. (2021). Comprehensive servo control strategies for flexible and high-efficient wire electric discharge machining. A systematic review. Precision Engineering, 71, 7–28. https://doi.org/10.1016/j.precisioneng.2021.02.007
- Babu, B. S., Sathiyaraj, S., Ramesh, A. K. P., Afridi, B. A., & Varghese, K. K. (2021). Investigation of machining characteristics of aluminium 6061 by wire cut EDM process. Materials Today: Proceedings, 45, 6247–6252. https://doi.org/10.1016/j.matpr.2020.10.698
- Battina, N. M., Hari Krishna, C., & Vanthala, V. S. P. (2022). Influence of pin profile on formability of friction stir-welded aluminum tailor-welded blanks: an experimental and finite element simulation analysis. Transactions of the Canadian Society for Mechanical Engineering, 46(3), 602–613. https://doi.org/10.1139/tcsme-2022-0031
- Battina, N. M., Vanthala, V. S. P., & Ch, H. K. (2021). Effect of friction stir welding process parameters on mechanical and metallurgical behavior of AA6061-T6 and AA2017-T6 tailored blanks. Engineering Research Express, 3(2), 025043. https://doi.org/10.1088/2631-8695/ac09d9
- Boppana, S. B., Dayanand, S., Anil Kumar, M. R., Kumar, V., & Aravinda, T. (2020). Synthesis and characterization of nano graphene and ZrO2 reinforced Al 6061 metal matrix composites. Journal of Materials Research and Technology, 9(4), 7354–7362. https://doi.org/10.1016/j.jmrt.2020.05.013
- Chekuri, R. B. R., Eshwar, D., Kotteda, T. K., & Varma, R. S. (2022). Experimental and thermal investigation on die-sinking EDM using FEM and multi-objective optimization using WOA-CS. Sustainable Energy Technologies and Assessments, 50, 101860. https://doi.org/10.1016/j.seta.2021.101860
- Chockalingam, K., Jawahar, N., Muralidharan, N., & Jeyaraj, K. L. (2019). Material subtraction study of AISI T-15-HSS by wire cut electrical discharge machining (CNC-wire cut EDM) based on Taguchi grey relational analysis. International Journal of Machining and Machinability of Materials, 21(3), 139–168. https://doi.org/10.1504/IJMMM.2019.099481
- Dar, S. A., Kumar, J., Sharma, S., Singh, G., Singh, J., Aggarwal, V., Chohan, J., Kumar, R., Sharma, A., Mishra, M., & Obaid, A. J. (2022). Investigations on the effect of electrical discharge machining process parameters on the machining behavior of aluminium matrix composites. Materials Today: Proceedings, 48, 1048–1054. https://doi.org/10.1016/j.matpr.2021.07.126
- Dayakar, K., Raju, K. K., & Raju, C. R. B. (2019). Prediction and optimization of surface roughness and MRR in wire EDM of maraging steel 350. Materials Today: Proceedings, 18, 2123–2131. https://doi.org/10.1016/j.matpr.2019.06.635
- Devarasiddappa, D., & Chandrasekaran, M. (2020). Experimental investigation and optimization of sustainable performance measures during wire-cut EDM of Ti-6Al-4V alloy employing preference-based TLBO algorithm. Materials and Manufacturing Processes, 35(11), 1204–1213. https://doi.org/10.1080/10426914.2020.1762211
- Divya, M., Singh, S. K., Nagari, S., & Subbiah, R. (2020). Optimisation of machining parameters of wire-cut EDM on super alloy materials–A review. Materials Today: Proceedings, 26(2), 1021–1027. https://doi.org/10.1016/j.matpr.2020.01.306
- Doreswamy, D., Bongale, A. M., Piekarski, M., Bongale, A., Kumar, S., Pimenov, D. Y., Giasin, K., & Nadolny, K. (2021). Optimization and modeling of material removal rate in wire-EDM of silicon particle reinforced Al6061 composite. Materials (Basel, Switzerland), 14(21), 6420. https://doi.org/10.3390/ma14216420
- Gangil, M., Pradhan, M. K., & Purohit, R. (2017). Review on modelling and optimization of electrical discharge machining process using modern techniques. Materials Today: Proceedings, 4(2), 2048–2057. https://doi.org/10.1016/j.matpr.2017.02.050
- Gavisiddesha, P., Thotappa, C., Algur, V., & Suresh Reddy, B. (2022). Optimization of wire cut electric discharge machining characteristics of hybrid aluminium composites (Al6061/Gr/SiCp) using Taguchi method. In Sustainable Machining Strategies for Better Performance: Select Proceedings of SMSBP 2020. (pp. 49–60). Springer Singapore.
- Gill, R. S., Samra, P. S., & Kumar, A. (2022). Effect of different types of reinforcement on tribological properties of aluminium metal matrix composites (MMCs): A review of recent studies. Materials Today: Proceedings, 56, 3094–3101. https://doi.org/10.1016/j.matpr.2021.12.211
- Girisha, L., Sridhar, S., Tadepalli, L. D., Swetha, M., Subbiah, R., & Marichamy, S. (2021). Performance analysis and taguchi approach on wire cut EDM using microwave sintered chromium composite. Materials Today: Proceedings, 45, 2105–2108. https://doi.org/10.1016/j.matpr.2020.09.700
- Gore, A. S., & Patil, N. G. (2018). Wire electro discharge machining of metal matrix composites: A review. Procedia Manufacturing, 20, 41–52. https://doi.org/10.1016/j.promfg.2018.02.006
- Gupta, V., Singh, B., & Mishra, R. K. (2020). Machining of titanium and titanium alloys by electric discharge machining process: a review. International Journal of Machining and Machinability of Materials, 22(2), 99–121. https://doi.org/10.1504/IJMMM.2020.105661
- Hakami, F., Pramanik, A., & Basak, A. K. (2017). Tool wear and surface quality of metal matrix composites due to machining: A review. Proceedings of the Institution of Mechanical Engineers, Part B: Journal of Engineering Manufacture, 231(5), 739–752. https://doi.org/10.1177/0954405416667402
- Kannan, E., Trabelsi, Y., Boopathi, S., & Alagesan, S. (2022). Influences of cryogenically treated work material on near-dry wire-cut electrical discharge machining process. Surface Topography: Metrology and Properties, 10(1), 015027. https://doi.org/10.1088/2051-672X/ac53e1
- Karthikeyan, G., Elatharasan, G., Thulasi, S., & Vijayalakshmi, P. (2021). Tensile, compressive and heat transfer analysis of ZrO2 reinforced aluminum LM6 alloy metal matrix composites. Materials Today: Proceedings, 37(Part 2), 303–309. https://doi.org/10.1016/j.matpr.2020.05.270
- Karthikeyan, R., Senthil Kumar, V., Punitha, A., & Chavan, U. M. (2022). An integrated ANN-GA approach to maximize the material removal rate and to minimize the surface roughness of wire cut EDM on Titanium alloy. Advances in Materials and Processing Technologies, 8(1), 22–32. https://doi.org/10.1080/2374068X.2020.1793267
- Kavimani, V., Prakash, K. S., & Thankachan, T. (2019). Multi-objective optimization in WEDM process of graphene–SiC-magnesium composite through hybrid techniques. Measurement, 145, 335–349. https://doi.org/10.1016/j.measurement.2019.04.076
- Madhavarao, S., Rao, V. V. S. K., & Chekuri, R. B. R. (2024). Preparation and investigation of mechanical behavior and characterization of AA7475/ZrO2/Gr hybrid MMC. Engineering Research Express, 6(1), 015071. https://doi.org/10.1088/2631-8695/ad20b6
- Ming, W., Jia, H., Zhang, H., Zhang, Z., Liu, K., Du, J., Shen, F., & Zhang, G. (2020). A comprehensive review of electric discharge machining of advanced ceramics. Ceramics International, 46(14), 21813–21838. https://doi.org/10.1016/j.ceramint.2020.05.207
- Ming, W., Xie, Z., Ma, J., Du, J., Zhang, G., Cao, C., & Zhang, Y. (2021). Critical review on sustainable techniques in electrical discharge machining. Journal of Manufacturing Processes, 72, 375–399. https://doi.org/10.1016/j.jmapro.2021.10.035
- Nagaral, M., Auradi, V., Kori, S. A., & Hiremath, V. (2019). Investigations on mechanical and wear behavior of nano Al2O3 particulates reinforced AA7475 alloy composites. Journal of Mechanical Engineering and Sciences, 13(1), 4623–4635. https://doi.org/10.15282/jmes.13.1.2019.19.0389
- Naidu, B. V. V., Varaprasad, K. C., & Rao, K. P. (2021). Development of regression models for wire electrical discharge machining of aluminium metal matrix composites. Materials Today: Proceedings, 39, 236–239. https://doi.org/10.1016/j.matpr.2020.06.586
- Palanisamy, D., Devaraju, A., Manikandan, N., Balasubramanian, K., & Arulkirubakaran, D. (2020). Experimental investigation and optimization of process parameters in EDM of aluminium metal matrix composites. Materials Today: Proceedings, 22, 525–530. https://doi.org/10.1016/j.matpr.2019.08.145
- Pandiyarajan, R., Maran, P., Marimuthu, S., & Ganesh, K. C. (2017). Mechanical and tribological behavior of the metal matrix composite AA6061/ZrO2/C. Journal of Mechanical Science and Technology, 31(10), 4711–4717. https://doi.org/10.1007/s12206-017-0917-3
- Pandiyarajan, R., Maran, P., Marimuthu, S., & Prabakaran, M. P. (2022). Investigation on mechanical properties of ZrO2, C and AA6061 metal matrix composites. Advances in Materials and Processing Technologies, 8(sup1), 178–186. https://doi.org/10.1080/2374068X.2020.1810946
- Prakash, V., Kumar, P., Singh, P. K., Hussain, M., Das, A. K., & Chattopadhyaya, S. (2019). Micro-electrical discharge machining of difficult-to-machine materials: a review. Proceedings of the Institution of Mechanical Engineers, Part B: Journal of Engineering Manufacture, 233(2), 339–370. https://doi.org/10.1177/0954405417718591
- Praveen, D. V., Raju, D. R., & Raju, M. V. J. (2020). Optimization of machining parameters of wire-cut EDM on ceramic particles reinforced Al-metal matrix composites – A review. Materials Today: Proceedings, 23(3), 495–498. https://doi.org/10.1016/j.matpr.2019.05.392
- Sathish, T., Mohanavel, V., Ansari, K., Saravanan, R., Karthick, A., Afzal, A., Alamri, S., & Saleel, C. A. (2021). Synthesis and characterization of mechanical properties and wire cut EDM process parameters analysis in AZ61 magnesium Alloy + B4C + SiC. Materials (Basel, Switzerland), 14(13), 3689. https://doi.org/10.3390/ma14133689
- Seshaiah, S., Sampathkumar, D., Mariappan, M., Mohankumar, A., Balachandran, G., Kaliyamoorthy, M., Rajendran, B., & Gopal, R. (2022). Optimization on material removal rate and surface roughness of stainless steel 304 wire cut EDM by response surface methodology. Advances in Materials Science and Engineering, 2022, 1–10. https://doi.org/10.1155/2022/6022550
- Siddesh Kumar, N. G., Shiva Shankar, G. S., Basavarajappa, S., & Suresh, R. (2017). Some studies on mechanical and machining characteristics of Al2219/n-B4C/MoS2 nano-hybrid metal matrix composites. Measurement, 107, 1–11. https://doi.org/10.1016/j.measurement.2017.05.003
- Singuru, M., V.v.s, K. R., & Chekuri, R. B. R. (2024). Optimization and modelling of WCEDM process parameters for GZR-AA7475 HMMC using GRA and Taguchi approach. World Journal of Engineering, https://doi.org/10.1108/WJE-09-2023-0354
- Slătineanu, L., Dodun, O., Coteaţă, M., Nagîţ, G., Băncescu, I. B., & Hriţuc, A. (2020). Wire electrical discharge machining: A review. Machines, 8(4), 69. https://doi.org/10.3390/machines8040069
- Subrahmanyam, M., & Nancharaiah, T. (2020). Optimization of process parameters in wire-cut EDM of Inconel 625 using Taguchi’s approach. Materials Today: Proceedings, 23(3), 642–646. https://doi.org/10.1016/j.matpr.2019.05.449