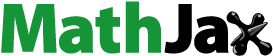
Abstract
In this article, a wireless power transfer (WPT) system with a single transmitter to power multiple electronic loads simultaneously using multiple receivers is proposed. We analyzed various types of coil geometries on the receiver side, including circular, square, hexagonal and octagonal shapes. Then, employing the finite element analysis (FEA) method with the ANSYS MAXWELL simulation tool, we identified the most efficient coil design system based on parameters, such as self-inductance, mutual inductance and coefficient of coupling factor. Subsequently, the electrical parameters necessary for the proposed concept were calculated from the equivalent circuit model and simulation of the proposed model was performed using the LT-Spice simulation tool. An 85 kHz resonant frequency was achieved using a high-frequency inverter (HFI), and inductor-capacitor-capacitor (LCC) compensation topologies were employed to provide different power capabilities to the loads.
1. Introduction
Wireless power transfer (WPT) systems are widely used in various electronic applications, including consumer-based portable electronics (Yalong et al., Citation2013; Feng et al., Citation2017), medical equipment (Xu et al., Citation2013; Varghese & Bobba, Citation2016), autonomous vehicles, such as autonomous underwater vehicles (AUVs) (Bobba et al., Citation2023) and unmanned aerial vehicles (UAVs) (Satyavani et al., Citation2021; Vaishnavi & Bobba, Citation2019), which have gained increasing attention in recent days. Researchers have designed numerous models for wirelessly powering electronic devices, including battery charging applications (Buja et al., Citation2015).
The conventional WPT model typically involves a single load (Ahmad et al., Citation2018). However, recent research has shifted focus toward a system with multiple loads, where power is transferred from one source to multiple loads simultaneously (Zhang et al., Citation2015). One such approach involves using multiple receiver coils to balance the series-connected battery voltages (Liu et al., Citation2018). Another method employs receiver circuits with different resonant frequencies, allowing specific receiver coils to receive power by adjusting the operational frequency of the power source (Ahn & Hong, Citation2013). However, in these systems, not all receiver coils can receive power simultaneously.
In the reference article, author proposed a dual-side LCC topology for an efficient WPT system capable of powering for multiple loads (Sreelakshmi & Bobba, Citation2021; Vu et al., Citation2018). In Pruthvi et al. (Pruthvi et al., Citation2018), various coil structures were chosen and simulated under various misalignment cases. Additionally, the variation of magnetic fields between the transmitter coil and receiver pads for distinct misalignment cases were observed and analyzed in this article. Thus, this article provides a better coil structure for a feasible universal wireless charging system (UWC) for smartphone and laptop applications. Lateral misalignment is a major factor hampering the advancement of this WPT technology. In this article, a structure consisting of a transmission coil and four cascaded receiving coils for a wireless charging system is proposed to suppress fluctuation in mutual inductance with lateral misalignments (Li & Yi, Citation2020). To improve efficiency in the case of lateral misalignments, a method that adjusts an equivalent load resistor has been proposed (Huang et al., Citation2014). In addition, a constant output voltage is obtained by adjusting the equivalent load resistor. However, the equivalent load resistor corresponding to the maximum efficiency is not the same as that corresponding to the constant output voltage for a given mutual inductance (Zhang et al., Citation2015; Zhang et al., Citation2015). To concurrently achieve high efficiency and constant output voltage for WPT, a maximum efficiency point tracking control scheme has been proposed (Li et al., Citation2015).
This article focuses on the design of WPT coils using multiple receivers for powering various electronic applications. The system operates at a high frequency of 85 kHz, and circular coil structure are chosen for design. All the receiver coils lie on the same plane, with equal distances maintained between each receiver and the transmitter. The effectiveness of the proposed model is analyzed using the ANSYS MAXWELL and LT-Spice simulation tool.
The remainder of this article is organized as follows: Section 2 describes the proposed model. Section 3 discusses the analysis of WPT coils. Section 4 elaborates on the design of single transmitter and multi-receiver (STMR) coil in ANSYS MAXWELL simulation tool. Section 5 presents the results of the ANSYS MAXWELL 3D simulator. Section 6 covers the LT-Spice simulation and the analysis of results for the STMR WPT system. Finally, Section 7 provides the conclusion.
2. Proposed model of multi receivers
is the illustration of STMRs WPT system. The proposed model of single transmitter multi-receiver WPT system is designed in ANSYS MAXWELL simulation tool and it is operated by using high frequency inverter (HFI) at 85 kHz, which is taken from the SAE standards of WPT. The method of inductor-capacitor-capacitor (LCC) compensation capacitance is proposed to suppress the effect of cross-coupling mutual inductance. LCC topology are profound as good for tunning purpose and to get good coupling by keeping transfer distance between transmitter and receiver coils as high. The single transmitter multi-receiver coils with the transfer distance of 100 mm between them and 160 mm mutual distance in between receiver coils. And all the receiver coils are placed in the same plane.
3. Analysis of WPT coils
3.1. System with single transmitter and multi receiver WPT
The proposed model of the WPT system can be represented in terms of lumped circuit elements: inductor (L), capacitor (C) and resistor (R), as shown in . Rp is the parasitic resistor of the transmitter coil (Tx), Rs1 is the parasitic resistor of Rx1, Rs2 is the parasitic resistor of Rx2, Rs3 is the parasitic resistor of Rx3 and Rs4 is the parasitic resistor of Rx4. L1 represents the inductance of Tx, L2 is the inductance of Rx1, L3 is the inductance of Rx2, L4 is the inductance of Rx3 and L5 is the inductance of Rx4. Cs1 is the external compensation capacitance of Rx1, Cs2 is the external compensation capacitance of Rx2, Cs3 is the external compensation capacitance of Rx3 and Cs4 is the external compensation capacitance of Rx4. M12 represents the mutual inductance between Tx and Rx1, M13 is the mutual inductance between Tx and Rx2, M14 is the mutual inductance between Tx and Rx3, M15 is the mutual inductance between Tx and Rx4, M23 is the mutual inductance between Rx1 and Rx2, M24 is the mutual inductance between Rx1 and Rx3, M25 is the mutual inductance between Rx1 and Rx4, M34 is the mutual inductance between Rx2 and Rx3, M35 is the mutual inductance between Rx2 and Rx4 and M45 is the mutual inductance between Rx3 and Rx4.
The equivalent circuit of the proposed model is shown in . All the receiver coils are coupled to the transmitter coil. The geometry of all the receiver coils is similar. In a magnetic coupling resonant wireless power transmission system, power is transferred by coupled coils. The coupling coefficient at the resonant frequency.
(1)
(1)
The coupling coefficient can be equated as
(2)
(2)
By applying Kirchhoff’s Voltage Law (KVL), of proposed model is presented as follows
(3)
(3)
where I1 is the current of transmitter coil (Tx), I2 is the current of receiver 1 (Rx1), I3 is the current of receiver 2 (Rx2). I4 is the current of receiver 3 (Rx3). I5 is the current of receiver 4 (Rx4).
According to above equations, the relation between I2 an IΟ is as follows:
(4)
(4)
According to Equation(3)(3)
(3) and Equation(5)
(5)
(5) , the system model for proposed structure can be expressed as follows:
(5)
(5)
The Current can be obtained by solving (6)
(6)
(6)
where
The output voltage of the load resistor is obtained by
(7)
(7)
According to (7) and (8), the expressions of Output power and the efficiency can be obtained as follows:
(8)
(8)
(9)
(9)
The compensation capacitance for each receiving coil is utilized to mitigate the impact of cross-coupling mutual inductance on the output voltage. This compensation is achieved through the use of capacitance as follows:
(10)
(10)
4. Single transmitter multi-receivers coil design in ANSYS MAXWELL
4.1. Transmitter and receiver coils design
The single-transmitter multi-receiver coils were designed using ANSYS MAXWELL simulation software, commonly employed for finite element analysis (FEA). Both the top and side views of the spiral coil are provided in and .
The inductance of the transmitter and receiver coils are determined by Wheeler (Wheeler, Citation1928)
(11)
(11)
(12)
(12)
where Dout and Din represent the outer and inner diameters of the spiral coil, S stands for the distance between two wires, W denotes the diameter of the wire and N signifies the number of turns. The design of transmitter and receiver coils is based on EquationEquations (11)
(11)
(11) and Equation(12)
(12)
(12) .
4.2. Analysis of different geometry at receiver side in single transmitter multi- receiver system
displays the top view of a circular-type transmitter and the corresponding multi-receiver coils of the same circular type. Similarly, exhibits the top view of a circular-type transmitter paired with square-type multi-receiver coils. showcases the top view of a circular-type transmitter with hexagonal-type multi-receiver coils, while illustrates the top view of a circular-type transmitter and octagonal-type multi-receiver coils. In all of , the transmitter coil is circular, and the receiver coils in their respective figures are positioned on the same plane. These figures were generated using the ANSYS MAXWELL simulation tool.
displays the parameters utilized in coil design for the analysis of various geometries in multiple receivers. These parameters, listed in the table above, are considered for all geometries, including circular, square, hexagonal, and octagonal-shaped coils.
Table 1. Coil Parameters for different geometry in receiver side of system.
4.3. Results obtained in ANSYS MAXWELL simulator
presents a graph depicting mutual inductance based on the different geometries employed on the receiver side of the single-transmitter and multi-receiver coils.
Additionally, provides a graph representing the coefficient of coupling for the various receiver geometries in the single-transmitter and multi-receiver setups. It is evident from both and that receiver coils with circular geometries exhibit higher mutual inductance and favorable coefficient of coupling factors. The order of mutual inductance and coefficient of coupling, as determined through FEA using the ANSYS MAXWELL simulation tool, is as follows: first, circular-shaped receiver coil; second, octagonal-shaped receiver coil; third, hexagonal-shaped receiver coil; and lastly, square-shaped receiver coil.
4.4. Circular type-based geometry for single transmitter multi-receiver proposed model
In , we present the top view of circular-type receivers utilized in the single-transmission multi-receiver setup. Additionally, in , an isometric view of the circular-type receiver coils is depicted, illustrating their placement at a specific distance within the proposed single-transmitter multi-receiver system. The transmitter coil is larger than the receiver coils in size. Furthermore, each receiver coil shares identical dimensions. All receiver coils, namely Rx1, Rx2, Rx3 and Rx4, are situated on the same plane. Both the transmitter and receiver are positioned in parallel, with a transfer distance of 100 mm between the transmitter coil and the receiver coils.
The multi-receiver coils and transmitter were designed based on the parameters outlined in . The transfer distance between the transmitter coil and the receiver coils remains at 100 mm. Additionally, all multi-receiver coils are positioned 160 mm apart from each other and are situated on the same plane. illustrates the isometric view demonstrating the placement of the transmitter and multi-receiver coils.
Table 2. Coil parameters.
The flux linkage between the multi-receiver coils primarily depends on the coupling factor. As the transfer distance between the transmitter and multi-receiver coils increases, the coupling between them weakens, as expressed in the equation.
5. ANSYS MAXWELL 3D simulation results
displays the self-inductance between the transmitter and multi-receiver coils at a transfer distance of 100 mm.
Table 3. Self inductance.
showcases the mutual inductance between the transmitter and multi-receiver coils, also at a transfer distance of 100 mm.
Table 4. Mutual inductance.
presents the coefficient of coupling between the transmitter and receiver coils, maintaining a transfer distance of 100 mm.
Table 5. Coefficient of coupling.
illustrates the coefficient of coupling graph based on variations in the transfer distance between the transmitter and receiver coils. When the transfer distance increased from 10 mm to 100 mm, the coefficient of coupling changes accordingly. The red curve represents the coefficient of coupling between transmitter and receiver coil 1, the violet curve represents the coefficient of coupling between transmitter and receiver coil 2, the blue curve represents the coefficient of coupling between transmitter and receiver coil 3 and the purple curve represents the coefficient of coupling between transmitter and receiver coil 4. This graph was obtained using the FEA method with ANSYS MAXWELL simulation tool. The operating frequency during simulation was 85 kHz.
depicts the mutual inductance graph in relation to the varying transfer distance between the transmitter coil and receiver coils. When the transfer distance increased from 10 mm to 100 mm, the mutual inductance changes accordingly. The red curve represents the mutual inductance between transmitter and receiver coil 1, the violet curve represents the mutual inductance between transmitter and receiver coil 2, the blue curve represents the mutual inductance between transmitter and receiver coil 3, the purple curve represents the mutual inductance between transmitter and receiver coil 4. This graph was obtained using the FEA method with ANSYS MAXWELL imulation tool. The operating frequency during simulation was 85 kHz.
In and , the magnetic field intensity and magnetic density are demonstrated, both acquired through FEA using the ANSYS MAXWELL 3D simulator. These results pertain to the circular-type receivers utilized in the proposed concept of the single-transmitter multi-receiver WPT system.
6. LT-Spice simulation and results analysis of STMR WPT system
6.1. LT-Spice simulation model of STMR-WPT system
shows the LTspice simulation circuit of the proposed model for the STMR-WPT system. The inductance and coefficient of coupling values for the transmitter and multi-receiver coils are derived from the ANSYS Maxwell 3D simulation results, as shown in and , respectively, for a 100 mm transfer distance. This LT-Spice simulation was conducted at an operating frequency of 85 kHz, with a 28 V DC supply provided to the high-frequency inverter (HFI) in the proposed model. The HFI’s output is then fed to the transmitter coil through LCC compensation components.
On the receiver side, four receiver coils are connected to loads with varying power requirements. Each receiver coil includes a secondary capacitance, and the output of the receiver coils is connected to a rectifier circuit to convert the output to DC. The ripple content of the rectifier is filtered out by an LC filter, and the output power of the receivers is measured at the end of the resistive load of each receiver coil section.
6.2. Input voltage and input current of proposed system
In , the input voltage (left y-axis) and current waveforms (right y-axis) for the proposed model of STMR are depicted over time (x-axis). The voltage waveform is represented in blue, and the current waveform is represented in red. This simulation was conducted using the LTspice simulation tool. A 28-volt input is supplied to the HFI, which operates at 85 kHz. The output of this inverter is then transmitted to the STMR's transmitter coil through LCC compensation components.
6.3. Output power obtained at the end of the multi-receivers
From , output power waveforms (y-axis) simulated toward time (x-axis) is using LT-Spice simulation tool. The simulation was performed based on the equivalent model of STMR system shown in . In this model, LCC compensation topology is employed to achieve a good coefficient of coupling between transmitter and multi-receiver coils. The transfer distance between transmitter and multi-receiver coils is set to 100 mm and operating frequency of 85 kHz. The output of the receiver coils is connected to rectifier which is shown in and inductor-capacitor (LC) filters are used to filter out the ripple content of rectifier and gives output as direct current (DC). shows that the output power of 95 W is obtained at end of the resistive load of the receiver coil 1 (Rx1) section, shows that the output power of 115 W is obtained at end of the resistive load of the receiver coil 2 (Rx2) section, shows that the output power of 135 is obtained at end of the resistive load of the receiver coil 3 (Rx3) section, shows that the output power of 60 W is obtained at end of the resistive load of the receiver coil 4 (Rx4) section. All the receiver end output waveforms are shown in common figure in for effective visualizations. The output power ratings of these receiver coils vary because each receiver coils are meticulously designed to power a different load rating.
7. Conclusion
In this article, we simulated a system for STMR WPT to power a load with different powering capabilities. A detailed comparison of different coil geometries (circular, square, hexagonal and octagonal) on the receiver side of single-transmitter multi-receiver coils was analyzed using the FEA method with ANSYS MAXWELL simulation tool. It was found that the circular design exhibited favorable results in terms of self-inductance, mutual inductance and coefficient of coupling. Therefore, for further analysis, we selected the circular-shaped coil design on the receiver side for single-transmitter and multi-receiver coils. This design was then implemented and evaluated using the ANSYS MAXWELL simulation tool, with a coil distance of 100 mm. The coupling coefficient exhibited significant variation with an operating frequency of 85 kHz. On the receiver side, we employed multiple receiver coils, separated by a distance of 160 mm, all placed on the same plane. The transmitter side featured a single, larger coil compared to the receiver coils, which shared a consistent design. Implementing LCC compensation topologies resulted in a favorable coupling factor. The mutual inductance and coefficient of coupling values obtained from ANSYS MAXWELL for circular coil as a receiver side of STMR coils and other electrical parameter are obtained from equivalent circuit model shown in are used in the LT-Spice simulation tool.
For the proposed system, the input supply parameters were set to 28 volts and an operating frequency of 85 kHz. The simulations were conducted using the LTspice simulation tool, and the results showed an output power of 95 W at receiver 1 coil (Rx1), 115 W at receiver 2 coil (Rx2), 135 W at receiver 3 coil (Rx3) and 60 W at receiver 4 coil (Rx4). This demonstrates that our proposed system can power a load with varying powering capabilities.
Author contributions
Conceptualization, S.P.Y, P.B.B.,Y.S., P.N, S.D and A.J.; methodology, S.P.Y, P.B.B.,Y.S., P.N,.; software, S.P.Y and P.B.B.; validation, S.P.Y, P.B.B., Y.S .and P.N.; formal analysis, S.P.Y, P.B.B., Y.S., P.N, S.D and A.J.; investigation S.P.Y and P.B.B.; resources, S.P.Y and P.B.B.; data curation, S.P.Y, P.B.B.,Y.S.,P.N, S.D and A.J.; writing – original draft preparation, S.P.Y, P.B.B.,Y.S.,P.N, S.D and A.J.; writing – review and editing, S.P.Y and P.B.B.; visualization, S.P.Y, P.B.B.,Y.S., P.N, S.D and A.J.; supervision,. S.P.Y, P.B.B. and Y.S; project administration, S.P.Y, P.B.B, Y.S., P.N, S.D and A.J.; funding acquisition, P.B.B., Y.S.V and S.D.
Nomenclature | ||
FEA | = | Finite Element Analysis |
HFI | = | High-Frequency Inverter |
kHz | = | Kilohertz |
LCC | = | Inductor-Capacitor-Capacitor |
STMR | = | Single Transmitter and Multi-Receivers |
WPT | = | Wireless Power Transfer |
Disclosure statement
There are no interests to declare.
Data availability statement
The original contributions presented in the study are included in the article; further inquiries can be directed to the corresponding author.
References
- Ahmad, A., Alam, M. S., & Chabaan, R. (2018). A comprehensive review of wireless charging technologies for electric vehicles. IEEE Transactions on Transportation Electrification, 4(1), 38–63. https://doi.org/10.1109/TTE.2017.2771619
- Ahn, D., & Hong, S. (2013). A study on magnetic field repeater in wireless power transfer. IEEE Transactions on Industrial Electronics, 60(1), 360–371. https://doi.org/10.1109/TIE.2012.2188254
- Bobba, P. B., Anwar, M. H., Yadav, S. P., & Rao, D. S. (2023 Effect of coil geometry and dimensions on the performance of wireless power transfer in AUV application [Paper presentation]. 2023 IEEE 3rd International Conference on Sustainable Energy and Future Electric Transportation (SEFET) (pp. 1–7), Bhubaneswar, India. https://doi.org/10.1109/SeFeT57834.2023.10244892
- Buja, G., Bertoluzzo, M., & Mude, K. N. (2015). Design and experimentation of WPT charger for electric city car. IEEE Transactions on Industrial Electronics, 62(12), 7436–7447. https://doi.org/10.1109/TIE.2015.2455524
- Feng, J., Li, Q., & Lee, F. C. (2017 Omni-direcitional wireless power transfer for portable devices [Paper presentation]. 2017 IEEE Applied Power Electronics Conference and Exposition (APEC) (pp. 1675–1681), Tampa, FL. https://doi.org/10.1109/APEC.2017.7930924
- Huang, S. D., Li, Z. Q., & Li, Y. (2014). Transfer efficiency analysis of magnetic resonance wireless power transfer with intermediate resonant coil. Journal of Applied Physics, 115(17), 17A336. https://doi.org/10.1063/1.4867125
- Li, H., Li, J., Wang, K., Chen, W., & Yang, X. (2015). A maximum efficiency point tracking control scheme for wireless power transfer systems using magnetic resonant coupling. IEEE Transactions on Power Electronics, 30(7), 3998–4008. https://doi.org/10.1109/TPEL.2014.2349534
- Li, Z., & Yi, J. (2020). Modeling and design of a transmission coil and four cascaded receiving coils wireless charging structure with lateral misalignments. IEEE Access, 8, 75976–75985. https://doi.org/10.1109/ACCESS.2020.2988888
- Liu, M., Fu, M., Wang, Y., & Ma, C. (2018). Battery cell equalization via megahertz multiple-receiver wireless power transfer. IEEE Transactions on Power Electronics, 33(5), 4135–4144. https://doi.org/10.1109/TPEL.2017.2713407
- Pruthvi, T. V., Kavitha, M., Bobba, P. B., & Reddy, D. M. (2018 Design of a universal wireless charging system for mobile and laptop applications [Paper presentation]. 2018 IEEE International Conference on Power Electronics, Drives and Energy Systems (PEDES) (pp. 1–6), Chennai, India. https://doi.org/10.1109/PEDES.2018.8707924
- Satyavani, Y., Bobba, P. B., & Sandeep, V. (2021 Design and development of wireless power transfer system for UAV [Paper presentation]. 2021 International Conference on Sustainable Energy and Future Electric Transportation (SEFET) (pp. 1–6), Hyderabad, India. https://doi.org/10.1109/SeFet48154.2021.9375735
- Sreelakshmi, K., & Bobba, P. B. (2021) Design and development of efficient wireless power transfer system for multiple loads [Paper presentation]. 2021 International Conference on Sustainable Energy and Future Electric Transportation (SEFET) (pp. 1–6), Hyderabad, India. https://doi.org/10.1109/SeFet48154.2021.9375663
- Vaishnavi, M., & Bobba, P. B. (2019 Extensive analysis on load dynamics of an unmanned aerial vehicle [Paper presentation]. 2019 IEEE 5th International Conference for Convergence in Technology (I2CT) (pp. 1–6), Bombay, India. https://doi.org/10.1109/I2CT45611.2019.9033633
- Varghese, B. J., & Bobba, P. B. (2016 Design and analysis of a robust system for wirelessly powering implantable devices [Paper presentation].2016 IEEE 1st International Conference on Power Electronics, Intelligent Control and Energy Systems (ICPEICES) (pp. 1–5), Delhi, India. https://doi.org/10.1109/ICPEICES.2016.7853422
- Vu, V.-B., Tran, D.-H., & Choi, W. (2018). Implementation of the constant current and constant voltage charge of inductive power transfer systems with the double-sided LCC compensation topology for electric vehicle battery charge applications. IEEE Transactions on Power Electronics, 33(9), 7398–7410. https://doi.org/10.1109/TPEL.2017.2766605
- Wheeler, H. A. (1928). Simple inductance formulas for radio coils. Proceedings of the Institute of Radio Engineers, 16(10), 1398–1400. https://doi.org/10.1109/JRPROC.1928.221309
- Xu, Q., Wang, H., Gao, Z., Mao, Z. H., He, J., & Sun, M. (2013). A novel mat-based system for position-varying wireless power transfer to biomedical implants. IEEE Transactions on Magnetics, 49(8), 4774–4779. https://doi.org/10.1109/TMAG.2013.2245335
- Yalong, Z., Xueliang, H., Jiaming, Z., & Linlin, T. (2013). Design of wireless power supply system for the portable mobile device. Proceedings of IWS (pp. 1–4). https://doi.org/10.1109/IEEE-IWS.2013.6616717
- Zhang, Y., Lu, T., Zhao, Z., Chen, K., He, F., & Yuan, L. (2015). Wireless power transfer to multiple loads over various distances using relay resonators. IEEE Microwave and Wireless Components Letters, 25(5), 337–339. https://doi.org/10.1109/LMWC.2015.2409776
- Zhang, Y., Lu, T., Zhao, Z., He, F., Chen, K., & Yuan, L. (2015). Selective wireless power transfer to multiple loads using receivers of different resonant frequencies. IEEE Transactions on Power Electronics, 30(11), 6001–6005. https://doi.org/10.1109/TPEL.2014.2347966
- Zhang, Y., Lu, T., Zhao, Z., He, F., Chen, K., & Yuan, L. (2015). Employing load coils for multiple loads of resonant wireless power transfer. IEEE Transactions on Power Electronics, 30(11), 6174–6181. https://doi.org/10.1109/TPEL.2015.2396943