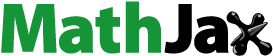
Abstract
The open hole tensile and flexural test of composites holds immense importance in aerospace and structural engineering. These tests provide critical insights into how composites perform under real-world conditions, allowing for the development of materials that meet rigorous standards, thereby ensuring the safety and durability of aircraft and other applications. This study investigates the influence of hole size on the open hole tensile (OHT) and flexural properties (OHF) of plain weave aramid/epoxy (AFRP), basalt/epoxy (BFRP), and aramid-basalt/epoxy (ABFRP) inter-ply hybrid laminates. Laminates were fabricated using hand layup followed by the compression molding method. The specimens were cut according to ASTM D5766 and ASTM D7264 to analyze OHT and OHF performance for three different hole sizes viz., 4, 6, and 8 mm. Results indicated that the ABFRP inter-ply layup composite significantly enhanced the mechanical performance of when compared to AFRP laminates. However, the decline in mechanical properties was observed in all the composites with the increase in hole size. BFRP laminates showed higher hole sensitivity under tensile loading due to its brittle fracture mechanism while AFRP exhibited higher hole sensitivity under flexural loading which can be attributed to its the poor compressive strength. The effect of hole size on the damage mechanism has also been highlighted through scanning electron microscope (SEM). The fibre fracture was observed to be dominant failure mechanism in large hole specimens while matrix cracking and delamination were found to be dominant failure mechanism in small holes.
1. Introduction
Over recent years, there has been substantial attention in the field of materials science, and aircraft applications, towards fiber-reinforced composites owing to their superior strength and stiffness-to-weight ratios in contrast to conventional materials such as metals or alloys (Agarwal & Broutman, Citation1990; Chawla, Citation2012; Mallick, Citation2007). In addition, the growing concern for environmental issues and regulations by authorities has promoted the employment of eco-friendly fibres in polymer reinforced composites (Chichane et al., Citation2023; Neto et al., Citation2022; Pandita et al., Citation2014). The natural, eco-friendly basalt fibres have recently gained attention as a potential substitute for glass or carbon reinforcements and are found in volcanic rocks originating from frozen lava (Czigány et al., Citation2005; Fiore et al., Citation2015). This new generation of basalt fibers boasts advantageous characteristics such as effective sound insulation, superior heat resistance surpassing that of glass, robust resistance against chemical exposure, and minimal water absorption (Elmahdy & Verleysen, Citation2020; Sarasini et al., Citation2013). The impressive mechanical strength of basalt fibers, coupled with their cost-effectiveness, positions this material as a potential alternative to glass fibers in diverse industrial sectors such as aerospace, automotive, transportation, and shipbuilding (Bandaru et al., Citation2017; Czigány, Citation2006; Dorigato & Pegoretti, Citation2014). Unfortunately, the exceptional stiffness and strength of these composites are accompanied by reduced toughness (Agarwal et al., Citation2023; Fernandes et al., Citation2023; Pai et al., Citation2021; Zubair & Pai, Citation2019). Given the urgent demand for innovative lightweight materials with enhanced toughness, there is a resurgence in research interest surrounding the concept of “hybridization” (Gupta & Srivastava, Citation2016; Swolfs et al., Citation2014). Hybrid composites showcase unique attributes that can effectively address a variety of conflicting design needs in a more economically efficient manner than traditional composites (Pandya et al., Citation2011; Phillips, Citation1976). Previous investigations have revealed that impact properties of basalt fibres can be enhanced by incorporating aramid fibres that have higher ductility (Pai et al., Citation2021b; Citation2022; Citation2023; Wang et al., Citation2008).
Hybrid composite structures reinforced with aramid and basalt have found extensive applications in aerospace, military, protective apparel, and marine industries. However, one of the primary factors affecting the design of composite structure is the load carrying capacity of the composite joints. Mechanical joints such as bolts, pins, screws, and rivets are the preferred choice for assembling primary structural composite components (Caminero et al., Citation2013; Guo et al., Citation2021; Jule et al., Citation2021). These joints require notches such as holes and slots to be drilled into the structures during practical applications to install necessary components. Holes may also form during the service life of the component due to impact damage. Hence, holes in composites create structural weaknesses, leading to reduced strength and shorter service life due to stress concentration near the notched areas (Green et al., Citation2007; Kumar et al., Citation2020). Ensuring safety and dependability in service makes it imperative to accurately measure residual properties and assess failure responses. Bandaru et al. (Citation2016) examined the influence of hybridization on the mechanical performance of polypropylene composites embedded with Kevlar and basalt fibres. According to the authors, hybridization enhanced the tensile behavior and increased the tensile modulus by 13.77% in comparison to Kevlar laminates. Sarasini et al. (Citation2013, Citation2014, Citation2016) studied the impact behavior of epoxy composites reinforced with carbon/basalt, glass/basalt, and aramid/basalt fibres. The findings revealed that hybrid laminates with a sandwich-like structure (intercalated) displayed enhanced flexural performance and greater absorption of impact energy compared to non-hybrid configurations. Previous studies have demonstrated that hybrid laminates with aramid fibres as outer plies are better at withstanding delamination damage when exposed to impact loads. This is in contrast to inner aramid plies, which are less effective in this regard (Dorey et al., Citation1978). Adding aramid layers as surface plies can provide protection to the primary load-bearing basalt plies (0°/90°) against impact damage (Vasudevan et al., Citation2018). In the event of an impact, the external aramid layer disperses the damage across a wider surface area starting from the impact point, effectively managing the risk of delamination within the plies. Pai et al. (Citation2021a) explored the mechanical strengths of aramid-basalt/epoxy inter-ply hybrid laminate with different orientation angle of surface aramid layer. The findings indicated that aramid fabrics positioned on the surface with a (0°/90°) orientation displayed superior mechanical properties when compared to alternative orientations. As a result, creating hybrid composites that incorporate these two types of fibers can effectively achieve a favorable equilibrium among tensile, flexural, and impact properties. Although several studies have investigated the mechanical properties of aramid-basalt hybrid composite, only a limited number of studies have documented the open-hole tensile (OHT) behavior and the mechanisms of failure in hybrid composites. Currently, as the use of brittle-toughness fibre structures and fabric reinforcements continues to expand, there is a need for further research. This study is crucial for gaining a deeper understanding of how these structures perform under open-hole conditions, ultimately ensuring their safety and reliability during service. Previous research into notched laminates has primarily concentrated on attributes related to the notches, encompassing aspects such as their quantity, hole profile, and dimensions. Cunningham et al. (Citation2015) explored how the arrangement of holes influenced the tensile behavior of laminates reinforced with glass fiber. Beyene et al. (Citation2016) explored how different notch configurations, including circular holes, transverse ellipses, longitudinal ellipses, and slot geometry, affected the flexural performance of glass/epoxy composites. The study revealed that notch geometry with sharp curvature changes, such as the rectangular slot geometry, results in greater stress concentration. This, in contrast to circular geometry, leads to a higher loss of strength. Sun et al. (Citation2019) studied the open hole tensile performance of carbon/epoxy and basalt/epoxy composites with different hole sizes. The author concluded that large hole sizes resulted in significant strength reduction. Sebaey and Wagih (Citation2019) studied the flexural characteristics of notched carbon-aramid/epoxy laminates for five-hole diameters using a four-point bending test and concluded that the hybridization methodology affected the flexural response.
Studying the mechanical behaviors of open-hole hybrid laminates under different loading conditions remains a challenging task, mainly because of the intricate nature of their damage mechanisms and failure modes. Although several studies have investigated the mechanical properties of aramid-basalt hybrid composite, only a limited number of studies have documented the open-hole tensile (OHT), flexural (OHF) behavior and the mechanisms of failure in hybrid composites. Currently, as the use of brittle-toughness fibre structures and fabric reinforcements continues to expand, there is a need for further research. This study is crucial for gaining a deeper understanding of how these structures perform under open-hole conditions, ultimately ensuring their safety and reliability during service. To the authors best knowledge, the mechanical behavior of aramid-basalt/epoxy hybrid interply laminate with drilled hole has not been explored in the existing research. Therefore, this paper outlines experimental studies on the tensile and flexural behavior of epoxy composites reinforced with aramid and basalt fibres, containing open holes (OH). Three different composite systems were considered for the study viz., aramid laminate (AFRP), basalt laminate (BFRP) and interply aramid-basalt hybrid laminate (ABFRP), in which a core of basalt layers is sandwiched between layers of aramid fibre. Three different hole diameters viz. 4, 6 and 8 mm are examined to study the influence of hole size on the tensile and flexural properties of the manufactured laminates. Further the damage modes of the fractured specimens have also been discussed.
2. Materials and procedures
2.1. Materials and fabrication
The materials used for the composite fabrication are, plain weave bidirectional (2-D) basalt and aramid fabrics as shown in , while the binding materials used are CT/E 556 liquid epoxy resin, and CT/H 951 hardener. The materials were supplied by M/S Composite Tomorrow, Gujarat, India. The Material details are provided in . The resin and hardener were mixed in the ratio of 100:10 and applied to the fabrics by hand lay-up. The five layered composite fabrication was carried out on a mild steel plate with dimensions of 350 × 350 mm. Thick metallic spacers were kept on all four sides of the plates to maintain a thickness of 2.8 mm. Three different laminates were fabricated viz., basalt/epoxy laminate (BFRP), aramid/epoxy laminate (AFRP) and aramid-basalt/epoxy interply hybrid laminate (ABFRP) as depicted in . The ABFRP comprised of a core of three layers of basalt fibres between surface aramid plies. After hand layup laminates were placed under the compression molding machine and compressed until the required thickness of 2.8 mm is achieved. The laminates were retained under compression molding machine for a curing period of 24 h at room temperature. The detailed process of fabrication, computation of laminate densities and void percentage of the laminates have been previously documented in Pai et al. (Citation2023). Theoretical densities of AFRP, BFRP and ABFRP are found to be 2175, 1855 and 1910 kg/m3 respectively.
Table 1. Physical properties of the reinforcements and matrix used.
3. Experimental method
3.1. Tensile test set up
Tensile testing was conducted using an Indian-manufactured BiSS Universal Testing Machine (UTM) with a maximum loading capacity of 50 kN, as shown in . Specimens were cut following the guidelines outlined in the ASTM D3039 (ASTM D3039/D3039M-14, Citation2014) and ASTM D5766 (Standard (ASTM), Citation2011) standard. The span dimension remained fixed at 150 mm, and the overall dimensions of the specimens were 250 mm in length and 25 mm in width. A circular shaped open hole with the diameters, viz., 4, 6 and 8 mm were drilled at the centre of each sample as shown in . The crosshead speed was maintained at a constant rate of 2 mm/min. The Data Acquisition System was employed to record both the applied force and specimen elongation, allowing for the subsequent calculation of the corresponding tensile properties. Five specimens were considered for test in each hole category.
3.2. Flexural test setup
The three point bending test was conducted following the ASTM D7264 (A. D7264, Citation2020) and ASTM D790 (ASTM D790, Citation2021) standard, using an Indian-manufactured UNITEK-9450 Universal Testing Machine (UTM) with a maximum loading capacity of 50 kN as illustrated in . A total of five specimens in each hole category, with a standard span to thickness ratio of 32:1 and 25 mm in width, were subjected to the experiment. The overall length was maintained at 20% greater than span length. A circular shaped open hole with diameters viz., 4, 6, and 8 mm were drilled at the centre of each sample as shown in . The flexural coupons were securely positioned between a pair of supports, and a centrally applied load at a rate of 1 mm/min was applied at the midpoint of the specimen. The displacement at the loading point was measured, and the corresponding flexural properties were calculated.
4. Results and discussion
4.1. Tensile behavior
illustrates the stress vs strain response of different composite systems for varying hole sizes. FRPs have linear elastic mechanical characteristics without a significant yielding stage, which reduces failure strain and elongation rates. The brittle elastic behavior of all laminates was evident, even when a notch was present. The sharp drop in load depicted in this curve, as specified in ASTM D3039 (ASTM D3039/D3039M-14, Citation2014), indicates significant damage occurring, ultimately leading to specimen fracture. also illustrates that the findings in this study align well with typical observations seen in the majority of FRP composites. Elastic modulus, tensile strength, and strain at failure were estimated using stress-strain curves in accordance with ASTM standards. shows the tensile characteristics of the tested laminates for various open hole diameters. A comparison between the mechanical performance of different composite systems as well as with increasing hole size is discussed in the next section.
Figure 7. Effect of hole size on stress-strain response of (a) AFRP, (b) ABFRP, and (c) BFRP composites.

Table 2. Tensile properties of AFRP, ABFRP and BFRP composite systems corresponding to varying hole size.
4.2. Tensile properties
depicts the affect of hole size on the tensile properties of the composite systems considered. Regardless of hole size, introducing basalt layers to aramid increases the tensile strength of the ABFRP laminate. BFRP displayed almost twice the tensile strength of the AFRP for the no hole case. Similar observation has been reported in (Bozkurt, Citation2015; Velumayil, Citation2022). This phenomenon can be elucidated by prior research, which demonstrated that blending high elongation/low modulus fibers like Kevlar or glass with low elongation/high modulus and rigid synthetic fibers such as carbon or basalt leads to a well-balanced hybrid composite laminate (Safri et al., Citation2018; Vara & Talupula, Citation2018). This interchangeably incorporates the desired mechanical properties from each reinforcement while minimizing undesired behavior (Swolfs et al., Citation2014; Wang et al., Citation2008).
Figure 8. Variation of tensile characteristics with hole size (a) Average tensile strength, (b) average tensile modulus and (c) average failure strain.

Basalt fibre is known to offer high strength and modulus but being brittle in nature it is often hybridized with ductile aramid fibres (Bulut, Citation2017; Chowdhury et al., Citation2020). Ductile materials like aramid absorb most of the applied load in the form of plastic deformation, hence they attain lower tensile strength. In contrast brittle materials are resistant to deformation and do not dissipate the applied energy before failure, thereby achieving higher strength. Bazan and Wierzbicka-Miernik (Citation2021) has also stated that the positive hybrid effect of aramid-basalt reinforcement is contributed by the aramid fibres tendency to delaminate but not brittle break when stretched and the high strength characteristics of basalt fibres. The higher tensile strength may also be associated with the higher density of the basalt fibres as reported in Velumayil (Citation2022). Therefore hybridization resulted in intermediate strength which was also explained by Hayashi (Citation1972) on the basis of synergistic effect called hybridization. The no hole ABFRP showed an increase of 28.97% in ultimate strength compared to AFRP. The improved mechanical performance due to aramid-basalt hybridization has been confirmed by various literatures (Bandaru et al., Citation2016; Pai et al., Citation2021; Citation2022; Sarasini et al., Citation2013).
The effect of hole size on the tensile strength is evident since ultimate strength decreased as hole size increased regardless of the material. To clearly examine the effect of hole diameter on the tensile performance of each material, strength retention was calculated and reported as shown in . shows the percentage loss in tensile strength compared to the no hole case for increasing hole sizes. The hybrid ABFRP laminates were found to record the least loss in strength as the hole size increased when compared to AFRP and BFRP laminates. However, BFRP showed the highest tensile strength at all hole sizes while AFRP showed the lowest tensile strength.
The strength retention observed was in the order of ABFRP > AFRP > BFRP. This also indicates the higher hole sensitivity of BFRP laminates. Due to its greater ductility and failure strain, AFRP can reduce stress concentration at the hole’s edge, redistribute loads away from the hole, and delay crack propagation, thus showing lower hole sensitivity than BFRP. While the basalt fibres being more brittle and less ductile, it resulted in faster fracture growth at low strain or earlier failure, which reduced strength retention. This indicates that basalt fibre is highly hole sensitive compared to other composite systems. At a hole size of 8 mm, BFRP lost more than 50% of its strength.
BFRP shows the highest tensile modulus for all hole sizes and AFRP shows the lowest modulus. Owing to the brittle nature of basalt fibres it resists deformation which is responsible for the higher modulus. The positive effect of hybridization is seen with the tensile modulus of hybrid ABFRP being intermediate (Hayashi, Citation1972; Swolfs et al., Citation2014). An increase in tensile modulus of 36.31% is seen in ABFRP compared to AFRP considering the no hole case. Increase in hole size had a negative effect on elastic modulus of the material. With the increase in hole size, modulus decreased regardless of the material. This decrease in modulus may be associated with stress concentration in the vicinity of the hole edge. The occurrence of hole introduces discontinuity in the fibres or in other words the woven fabrics are torn or damaged when a hole is drilled, thus causing a strong anisotropy in the laminate which deteriorates the modulus (Belgacem et al., Citation2018). Larger holes induce greater anisotropy which explains greater loss in modulus (Kumar et al., Citation2020). The introduction of an 8 mm hole showed a tensile modulus loss of 48.2%, 39.8% and 35.4% in BFRP, AFRP and ABFRP laminates respectively.
AFRP showed the highest tensile strain owing to its high ductility as mentioned in the previous section while BFRP showed the least tensile strain due to its brittle fracture behavior. The tensile strain of AFRP is 25.5% higher than BFRP laminate for the no hole case. An increase in strain is observed in basalt fibres with the insertion of aramid layers. Since the damage first initiated in the basalt fibres and transfer of stress to surface aramid fibres took place closer to failure load the stretching and bridging effect offered by aramid fibres allowed the hybrid ABFRP laminate to fail at a higher strain than in the case of only basalt fibres (Swolfs et al., Citation2014). Therefore, ABFRP laminate showed intermediate performance. Introduction of hole decreased the failure strain of the material. Presence of hole results in lesser area to support the stress which leads to early failure (Khashaba et al., Citation2007). The 4 mm hole caused a large decrease in strain compared to specimen without hole. Strain values of other hole sizes were only slightly reduced from this value. 8 mm hole size led to a decrease of 12.9%, 16.1% and 16.9% in tensile strain of AFRP, ABFRP and BFRP laminate respectively.
4.3. Damage assessment of fractured tensile specimens
4.3.1. Specimens without hole
indicates the various failure modes observed in three different composites without the hole. The AFRP laminate exhibited significant elongation before fibre breakage in the tensile loading direction at the fracture surface, indicating a ductile fracture. The greater strain response observed in aramid fibres is attributed to their high ductility. While the BFRP laminates showed smooth fracture surface which corresponds to brittle fracture as depicted in . The hybrid ABFRP laminate in showed the intervention of both these properties with the bridging effect from the top and bottom surface aramid layers. This failure mechanism accounts for the moderate strain response achieved through hybridization.
Figure 10. Fractured surfaces (top view and side view) of tensile specimens (a) AFRP, (b) BFRP, and (c) ABFRP composites.

SEM investigations were performed to comprehend the damage processes involved in AFRP and BFRP laminates as shown in . Interfacial adhesion examination through SEM is also useful to compare the mechanical strength between the different materials. In the elongated aramid fibres at the region of fracture confirm the ductile failure mode of the AFRP laminate. Additionally, the fibres in the fibre bundles are no longer bonded to the matrix and have been completely exposed at the surface. This indicates a weak bond between aramid fibres and the epoxy matrix. In contrast confirms the brittle failure mode of the BFRP laminate as it shows smoother cut of fibre breakage. The fibres show relatively better bonding with matrix since the epoxy is still adhering around the fibres hence holding them together which explains the superior mechanical properties of BFRP as compared to AFRP.
4.3.2. Open hole specimens
A close-up view of the fractured specimens for 6 mm hole size are shown in . The existence of the hole, serves as a stress riser in the specimen, is responsible for the localized rupture in the area around the hole. The hole causes an interruption in the continuity fibres, changing the stress distribution along the fibre orientation. Stress concentration causes the detachment of discontinuous fibre tips from the matrix at very low loads. This results in microcracks in the material, which, when subjected to additional loading, progress into larger cracks, eventually leading to fibre-matrix debonding and delamination. Ultimately, fibre breakage occurs resulting in complete fracture of the specimen at the failure load.
The fracture was seen to begin at the perimeter of the hole and grow transverse to the direction of tensile loading along the specimen width. shows stretched fibres which can be attributed to the high ductile nature of aramid fibres. Failure initiated with a matrix crack forming around the hole’s edge, subsequently propagating across the width, and ultimately resulting in fibre-matrix debonding and, eventually, fibre breakage. While in the case of hybrid ABFRP in the basalt fibres fractured first and were bridged by the surrounding ductile aramid fibres. This allowed for the stronger and stiffer basalt fibres to reach its ultimate strength giving rise to enhanced tensile strength of the hybrid structure. Delamination can be observed as whitish-yellow appearance due to the exposed aramid fibres separated from the basalt layer. BFRP laminate showed smoother fracture surface with damage is still confined to the area around the hole, confirming brittle fracture mechanism as shown in . Failure initiated with matrix cracks originating at the hole’s edge, then extending across the width. Subsequently, fibre breakage ensued, resulting in sudden fracture of the specimen into two pieces.
Delamination was evident in the hybrid ABFRP as whitish-yellow appearance on the top and bottom surface. This is due to matrix damage which debonds the surface aramid layer from the core basalt exposing the aramid fibres. shows that the delamination zone around the open hole decreased with the increase in hole size (Belgacem et al., Citation2018; Green et al., Citation2007; Shaari & Jumahat, Citation2017; Wisnom et al., Citation2008). This is because for a specimen with small hole size, more loads are required to be applied before failure can occur. Moreover, when the diameter is smaller the transverse cracks initiating from the hole edge can easily link up and propagate to larger available area between hole edge and specimen edge (Green et al., Citation2007; Wisnom et al., Citation2008).
4.4. Flexural behavior
depicts the flexural load versus deflection curves of different composite systems for varying hole sizes.
a indicates that the flexural curve of aramid laminate is relatively nonlinear due to the nonlinear compressive behavior of aramid fibres (Carl, Citation1978). From load versus deflection plots it can be deduced that with increase in load, deflection also increases and at a certain deflection it attains peak load after which it starts to decline. There is no sharp decline in the load instead, a plateau is seen at the peak load until the specimen completely fails (Sebaey & Wagih, Citation2019). Such a behavior is an indication of stable propagation of damage unlike BFRP laminate which shows a specific peak followed by a rapid decline in peak load within a smaller range of deflection as depicted in . This is associated with the brittle damage propagation of basalt fibres which has also been substantiated in the following sections. The load vs. deflection curve also demonstrates the coupling effect of hybridizing aramid and basalt fibres. Since the laminate features aramid layers on the surface, it effectively prevents catastrophic failure. Stress transfer occurs between the two reinforcements, allowing the hybrid laminate to exhibit intermediate performance. indicates the pseudo ductile behavior (Czél & Wisnom, Citation2013) of hybrid system comprising of initial linear response which then turns into nonlinear region owing to the plastic deformation in aramid layers. The flexural characteristics of the composite for various hole sizes is shown in .
Table 3. Flexural properties of composite systems for varying hole sizes.
4.5 Flexural properties
Flexural strength and flexural modulus were calculated using load displacement curves in accordance with ASTM standards. illustrate the influence of hole size on the flexural properties of the composite.
Figure 15. Variation of flexural properties (a) flexural strength and (b) flexural modulus of composites for different hole sizes.

It is obvious from the above results that the inter-ply hybrid specimen shows the highest strength retention. This is due to hybrid specimens can sustain loads even after the breakage of the low elongation fibres by transferring the load to the high elongation fibres at the surface (Basha et al., Citation2022). The introduction of the 8 mm hole caused a decrease in strength of 44.3%, 32.25%, and 81.09% for BFRP, ABFRP and AFRP respectively. AFRP showed higher hole sensitivity in flexural loading due to its poor compressive and bending properties (Bozkurt, Citation2015; Dorey et al., Citation1978; Sarasini et al., Citation2013). This indicates that 8 mm hole diameters mark an important hole dimension since it led to a significant reduction in strength. This is due to the larger amount of material removed, resulting in a reduced load-bearing area and consequently a greater decrease in strength. In addition, larger holes with specimen dimension or width are kept constant, the edge effect also comes into play. The higher proximity of the hole edge to the specimen edge facilitates instantaneous crack propagation to the edge of the specimen and cause early failure (Dorey et al., Citation1978), [77].
illustrates the loss in flexural strength with increasing hole size. Figure indicates that the presence of holes causes the flexural modulus to decrease. The flexural modulus decreases with increasing hole diameter due to the presence of holes causing a discontinuity in the fibre orientation, which, in turn, reduces the stiffness (Khashaba et al., Citation2007). Hybrid composite laminates displayed an elevation in flexural modulus when compared to AFRP composite laminate. The presence of high strength basalt layers increased the flexural modulus. The flexural modulus of BFRP laminate is nearly 3 times while the flexural modulus of ABFRP laminate is almost twice that of AFRP laminate.
4.6. Damage assessment of fractured flexural specimens
Compressive, tensile, shear, and/or delamination failures are commonly associated mechanisms in flexural loading (Subagia et al., Citation2014). Tensile forces act on the support face of the specimens during flexural loading, while compressive forces act on the loading face. The surface layers undergo maximum stress in the process. The fractured specimens with 6 mm hole damage modes are shown in . High stress concentrations which began at the hole’s edge and extended to the free edge were the root cause of all the specimens’ failures. The final failure occurred due to fibre breakage on the bottom surface. The bottom surface of fractured specimens showed more damage than the top surface indicating tensile failure of aramid fibres, which are known for their high toughness and ductility. Hence, aramid fibres tend to delaminate but not brittle break as shown in . The appearance of kinks or whitish areas near the loading point in composite materials reinforced with aramid fibres is the result of compressive failure of the fibres (Bazhenov, Citation1995). Due to the brittle fracture behavior of basalt fibres, increased crack propagation and fibre breakage are observed as shown in . In the hybrid specimen, , it is evident that matrix cracking first initiated in the lower basalt layers, subsequently leading to fibre rupture. This behavior has been elucidated by Kretsis (Citation1987) stating that in hybrid laminates, lower-strain fibres tend to break before higher-strain fibres. Thus, enabling the fibre with a lower strain and greater strength to approach its maximum strength. This indicates that the hybrid specimen’s flexural strength is higher than that of a laminate made entirely of aramid. Debonding and delamination occurred at the interface between the aramid and basalt layers due to a difference in the mechanical properties.
A decrease in delamination zone is observed with an increase in the diameter of the hole as indicated in . Similar observation has been reported in the previous section under tensile test. In comparison to smaller hole sizes, the catastrophic process zone is quite wide since it redistributes the stress concentration.
In conclusion, while the higher load carrying capacity of the basalt layers benefits the aramid layers, the higher tolerance to damage and ductile fracture properties of aramid fibres benefits basalt layers. These combined effects lead to improved flexural performance and delays the occurrence of final failure. Larger holes function as an adequate stress riser to cause failure, which causes an early fracture with a smaller delamination zone. Therefore, it can be said that fibre fracture is a dominant failure mechanism in large holes while matrix cracking and delamination are the dominant failure mechanism in small holes.
5. Conclusion
In this investigation, open hole tensile and flexural behavior were evaluated for aramid/epoxy (AFRP), basalt/epoxy (BFRP), and hybrid aramid-basalt/epoxy (ABFRP) composites for three different hole sizes 4, 6, and 8 mm respectively. The following findings can be drawn from the experimental evaluations:
The inclusion of basalt layers significantly enhanced the tensile strength and modulus of the hybrid composite laminate. The surface aramid layers in the inter-ply hybrid type lay-up sequence allowed for larger failure strain by allowing a more ductile failure.
The stress concentration effect at the hole boundary reduced the material’s tensile properties. Strength retention deteriorated for larger holes in the order of AFRP > ABFRP > BFRP.
BFRP laminates were more hole sensitive due to their brittle damage mechanism. Hybrid laminates showed delamination as a major failure mode due to the poor interfacial bonding and difference in mechanical properties between the aramid and basalt fibres.
Larger holes exhibited smaller delamination area. Matrix cracking, fibre fracture and fibre pull out were the mode of damage observed in the open hole specimens.
For specimens without holes, BFRP laminates displayed a flexural strength of 382 MPa, which is higher than that of ABFRP (226 MPa), and AFRP (160 MPa). As the hole diameter increases, the flexural strength reduces significantly. However, the introduction of 8 mm hole caused a decrease in strength of 44.3%, 32.25%, and 81.09% for BFRP, ABFRP and AFRP respectively.
Flexural strength and tensile strength reduction show reverse trends. BFRP laminate showed highest hole sensitivity under tensile loading due to its brittle damage mechanism leading to highest strength reduction while AFRP showed the highest strength reduction due to its poor compressive and bending properties which influenced the damage propagation. However, due to their ability to absorb higher energy and high ductility, resulted in considerable increase in failure strain of the hybrid laminate accompanied by stable failure than BFRP.
The results obtained from this research on open hole aramid-basalt/epoxy tensile and flexural specimens offer critical data that can be directly applied in engineering and industry to optimize the design and performance of composite structures, enhancing their durability, safety, and overall efficiency.
Author contributions
Oshin Fernandes: Conceptualization and design, Analysis, Interpretation of the data; Drafting of the paper. Jyoti Dutta: Conceptualization and design, Analysis, Interpretation of the data; Drafting of the paper. Yogeesha Pai: Conceptualization and design, Methodology, Analysis, Interpretation of the data; Drafting of the paper, Review, and editing. Deepthi G Pai: Conceptualization and design; Review and editing of the paper, Software.
Acknowledgments
The authors acknowledge “Department of Aeronautical and Automobile Engineering, Manipal Institute of Technology, Manipal” for providing Advanced Composite laboratory facility to carry out this research.
Disclosure statement
No potential conflict of interest was reported by the authors.
Data availability statement
The authors confirm that the data supporting the findings of this study are available within the article and its supplementary materials.
Additional information
Notes on contributors
Oshin Fernandes
Oshin Fernandes has obtained her B. Tech degree in Aeronautical Engineering from MIT Manipal, India. Her research interests include: Composite materials, Manufacturing and characterization.
Jyoti Dutta
Jyoti Dutta has obtained her B. Tech degree in Aeronautical Engineering from MIT Manipal, India. Her research interests include: Composite materials, Manufacturing and characterization.
Yogeesha Pai
Yogeesha Pai is a faculty in the Department of Aeronautical and Automobile engineering at Manipal Institute of Technology – Manipal Academy of Higher Education (MAHE) (Institute of Eminence)- Manipal, India. He has obtained his PhD degree in Polymer Composites from MIT Manipal and M.Tech degree in Aerospace Engineering from IIT Kharagpur, India. His research interests include: Advanced composite materials and characterization, Aircraft structures, Smart materials, Vibrational analysis.
Deepthi G. Pai
Deepthi G Pai has obtained her M.Tech from Software Engineering, and currently pursuing her PhD in Computer Science and Engineering, MIT Manipal, india. Her research interests include: Machine learning, Neural network and deep learning.
References
- A. D7264. (2020). Standard test method for flexural properties of polymer matrix composite. https://doi.org/10.1520/D7264
- Agarwal, B. D., & Broutman, L. J. (1990). Analysis and performance of fiber composites. 2nd ed. New Delhi: John Wiley & Sons Inc.
- Agarwal, S., Pai, Y., Pai, D., & Mahesha, G. T. (2023). Assessment of ageing effect on the mechanical and damping characteristics of thin quasi-isotropic hybrid carbon-Kevlar/epoxy intraply composites and damping characteristics of thin intraply composites. Cogent Engineering, 10(1). https://doi.org/10.1080/23311916.2023.2235111
- ASTM D3039/D3039M-14. (2014). Standard test method for tensile properties of polymer matrix composite. ASTM International.
- ASTM D790. (2021). Standard test methods for flexural properties of unreinforced and reinforced plastics and electrical insulating materials, 1–6.
- Bandaru, A. K., Patel, S., Sachan, Y., Ahmad, S., Alagirusamy, R., & Bhatnagar, N. (2016). Mechanical behavior of Kevlar/basalt reinforced polypropylene composites. Composites Part A: Applied Science and Manufacturing, 90, 642–652. doi: https://doi.org/10.1016/j.compositesa.2016.08.031
- Bandaru, A. K., Sachan, Y., Ahmad, S., Alagirusamy, R., & Bhatnagar, N. (2017). On the mechanical response of 2D plain woven and 3D angle-interlock fabrics. Composites Part B: Engineering, 118, 135–148. https://doi.org/10.1016/j.compositesb.2017.03.011
- Basha, M., Wagih, A., Melaibari, A., Lubineau, G., & Eltaher, M. A. (2022). On the impact damage resistance and tolerance improvement of hybrid CFRP/Kevlar sandwich composites. Microporous and Mesoporous Materials, 333(January), 111732. https://doi.org/10.1016/j.micromeso.2022.111732
- Bazan, P., & Wierzbicka-Miernik, A. (2021). A novel hybrid composites based on biopolyamide 10.10 with basalt/aramid fibers: Mechanical and thermal investigation. 223(June). https://doi.org/10.1016/j.compositesb.2021.109125
- Bazhenov, S. L. (1995). Bending failure of aramid fibre-reinforced composite. Composites, 26(11), 757–765. https://doi.org/10.1016/0010-4361(95)98196-R
- Belgacem, L., Ouinas, D., Aurelio, J., Olay, V., & Argüelles, A. (2018). Experimental investigation of notch effect and ply number on mechanical behavior of interply hybrid laminates (glass/carbon/epoxy). Composites Part B Engineering. 145(January), 189–196. https://doi.org/10.1016/j.compositesb.2018.03.026
- Beyene, A. T., Belingardi, G., & Koricho, E. G. (2016). Effect of notch on quasi-static and fatigue flexural performance of twill E-glass/epoxy composite. Composite Structures, 153, 825–842. https://doi.org/10.1016/j.compstruct.2016.05.094
- Bozkurt, Y. (2015). Hybridization effects on tensile and bending behavior of aramid/basalt fiber reinforced epoxy composites. https://doi.org/10.1002/pc
- Bulut, M. (2017). Mechanical characterization of basalt/epoxy composite laminates containing graphene nanopellets. Composites Part B Engineering, 122, 71–78. https://doi.org/10.1016/j.compositesb.2017.04.013
- Caminero, M. A., Lopez-Pedrosa, M., Pinna, C., & Soutis, C. (2013). Damage monitoring and analysis of composite laminates with an open hole and adhesively bonded repairs using digital image correlation. Composites Part B: Engineering, 53, 76–91. https://doi.org/10.1016/j.compositesb.2013.04.050
- Carl, Z. (1978). The flexural strength of aramid fiber composites. 12(4), 422–430. https://doi.org/10.1177/002199837801200407
- Chawla, K. K. (2012). Composite materials: Science and engineering. 3rd ed. New York: Springer Science & Business Media. https://doi.org/10.1007/978-0-387-74365-3
- Chichane, A., Boujmal, R., & El Barkany, A. (2023). Bio-composites and bio-hybrid composites reinforced with natural fibers: Review. Materials Today: Proceedings, 72, 3471–3479. https://doi.org/10.1016/j.matpr.2022.08.132
- Chowdhury, I. R., Nash, N. H., Portela, A., Dowd, N. P. O., & Comer, A. J. (2020). Analysis of failure modes for a non-crimp basalt fiber reinforced epoxy composite under flexural and interlaminar shear loading. Composite Structures, 245(April), 112317. https://doi.org/10.1016/j.compstruct.2020.112317
- Cunningham, D., Harries, K. A., & Bell, A. J. (2015). Open-hole tension capacity of pultruded GFRP having staggered hole arrangement. Engineering Structures, 95, 8–15. https://doi.org/10.1016/j.engstruct.2015.03.042
- Czél, G., & Wisnom, M. R. (2013). Demonstration of pseudo-ductility in high performance glass/epoxy composites by hybridisation with thin-ply carbon prepreg. Composites Part A: Applied Science and Manufacturing, 52, 23–30. https://doi.org/10.1016/j.compositesa.2013.04.006
- Czigány, T. (2006). Special manufacturing and characteristics of basalt fiber reinforced hybrid polypropylene composites: Mechanical properties and acoustic emission study. Composites Science and Technology, 66(16), 3210–3220. https://doi.org/10.1016/j.compscitech.2005.07.007
- Czigány, T., Vad, J., & Pölöskei, K. (2005). Basalt fiber as a reinforcement of polymer composites. Periodica Polytechnica Mechanical Engineering, 49(1), 3–14.
- Dorey, G., Sidey, G. R., & Hutchings, J. (1978). Impact properties of carbon fibre/Kevlar 49 fibre hydrid composites. Composites, 9(1), 25–32. https://doi.org/10.1016/0010-4361(78)90514-1
- Dorigato, A., & Pegoretti, A. (2014). Flexural and impact behaviour of carbon/basalt fibers hybrid laminates. Journal of Composite Materials, 48(9), 1121–1130. https://doi.org/10.1177/0021998313482158
- Elmahdy, A., & Verleysen, P. (2020). Mechanical behavior of basalt and glass textile composites at high strain rates: A comparison. Polymer Testing, 81(June 2019), 106224. https://doi.org/10.1016/j.polymertesting.2019.106224
- Fernandes, O., Dutta, J., & Pai, Y. (2023). Effect of various factors and hygrothermal ageing environment on the low velocity impact response of fibre reinforced polymer composites- a comprehensive review. Cogent Engineering, 10(1). https://doi.org/10.1080/23311916.2023.2247228
- Fiore, V., Scalici, T., Di Bella, G., & Valenza, A. (2015). A review on basalt fibre and its composites. Composites Part B: Engineering, 74, 74–94. Jun. https://doi.org/10.1016/j.compositesb.2014.12.034
- Green, B. G., Wisnom, M. R., & Hallett, S. R. (2007). An experimental investigation into the tensile strength scaling of notched composites. Composites Part A: Applied Science and Manufacturing, 38(3), 867–878. https://doi.org/10.1016/j.compositesa.2006.07.008
- Guo, Q., Zhang, Y., Li, D., Lv, Q., Sun, X., Ma, M., & Chen, L. (2021). Experimental and numerical investigation of open-hole tensile properties and damage mechanisms of 3D woven composites under weft-loading. Thin-Walled Structures, 161(December), 107455. https://doi.org/10.1016/j.tws.2021.107455
- Gupta, M. K., & Srivastava, R. K. (2016). A review on characterization of hybrid fibre reinforced polymer composite. Gupta MK American Journal of Polymer Science & Engineering, 4(1), 1–7.
- Hayashi, T. (1972). On the improvement of mechanical properties of composites by hybrid composition [Paper presentation]. Proc 8th Int Reinforced Plastics Conference, pp. 149–52.
- Jule, L. T., Ramaswamy, K., Nagaprasad, N., Shanmugam, V., & Vignesh, V. (2021). Design and analysis of serial drilled hole in composite material. Materials Today: Proceedings, 45, 5759–5763. https://doi.org/10.1016/j.matpr.2021.02.587
- Khashaba, U. A., Selmy, A. I., El-Sonbaty, I. A., & Megahed, M. (2007). GFR/EPOXY composites under static and fatigue loads. Composite Structures, 81(4), 606–613. https://doi.org/10.1016/j.compstruct.2006.11.005
- Kretsis, G. (1987). A review of the tensile, compressive, flexural and shear properties of hybrid fibre-reinforced plastics. Composites, 18(1), 13–23. https://doi.org/10.1016/0010-4361(87)90003-6
- Kumar, S. A., Rajesh, R., & Pugazhendhi, S. (2020). A review of stress concentration studies on fibre composite panels with holes/cutouts. Proceedings of the Institution of Mechanical Engineers, Part L: Journal of Materials: Design and Applications, 234(11), 1461–1472. https://doi.org/10.1177/1464420720944571
- Mallick, P. K. (2007). Fiber-reinforced composites materials, manufacturing and design. 3rd ed. Boca Raton: CRC Press Taylor & Francis Group. https://doi.org/10.3144/expresspolymlett.2007.115
- Neto, J., Queiroz, H., Aguiar, R., Lima, R., Cavalcanti, D., & Banea, M. D. (2022). A review of recent advances in hybrid natural fiber reinforced polymer composites. Journal of Renewable Materials, 10(3), 561–589. https://doi.org/10.32604/jrm.2022.017434
- Pai, Y., Dayananda Pai, K., & Vijaya Kini, M. (2023). Effect of ageing conditions on the low velocity impact behavior and damage characteristics of aramid-basalt/epoxy hybrid interply composites. Engineering Failure Analysis, 152(April), 107492. https://doi.org/10.1016/j.engfailanal.2023.107492
- Pai, Y., Pai K, D., & Kini, M. V. (2021). Effect of aramid fabric orientation angle on the mechanical characteristics of basalt-aramid/epoxy hybrid interply composites. Materials Research, 24(5) https://doi.org/10.1590/1980-5373-mr-2021-0209
- Pai, Y., Pai K, D., & Kini, M. V. (2022). Experimental investigations on the moisture absorption and mechanical behaviour of basalt-aramid/epoxy hybrid interply composites under different ageing environments. Cogent Engineering, 9(1), 0–20. https://doi.org/10.1080/23311916.2022.2080354
- Pai, Y., Pai, D. K., & Kini, M. V. (2021a). Evaluation of the mechanical characteristics of hygrothermally aged 2-D basalt-aramid/epoxy hybrid interply composites. Journal of Physics: Conference Series, 2070(1), 012234. https://doi.org/10.1088/1742-6596/2070/1/012234
- Pai, Y., Pai, K. D., & Kini, M. V. (2021b). A review on low velocity impact study of hybrid polymer composites. Materials Today: Proceedings, 46, 9073–9078. https://doi.org/10.1016/j.matpr.2021.05.390
- Pandita, S. D., Yuan, X., Manan, M. A., Lau, C. H., Subramanian, A. S., & Wei, J. (2014). Evaluation of jute/glass hybrid composite sandwich: Water resistance, impact properties and life cycle assessment. Journal of Reinforced Plastics and Composites, 33(1), 14–25. https://doi.org/10.1177/0731684413505349
- Pandya, K. S., Veerraju, C., & Naik, N. K. (2011). Hybrid composites made of carbon and glass woven fabrics under quasi-static loading. Materials & Design, 32(7), 4094–4099. https://doi.org/10.1016/j.matdes.2011.03.003
- Phillips, L. N. (1976). The hybrid effect – Does it exist? Composites, 7(1), 7–8. https://doi.org/10.1016/0010-4361(76)90273-1
- Safri, S. N. A., Sultan, M. T. H., Jawaid, M., & Jayakrishna, K. (2018). Impact behaviour of hybrid composites for structural applications: A review. Composites Part B: Engineering, 133, 112–121. https://doi.org/10.1016/j.compositesb.2017.09.008
- Sarasini, F., Tirillò, J., D'Altilia, S., Valente, T., Santulli, C., Touchard, F., Chocinski-Arnault, L., Mellier, D., Lampani, L., & Gaudenzi, P. (2016). Damage tolerance of carbon/flax hybrid composites subjected to low velocity impact. Composites Part B: Engineering, 91, 144–153. https://doi.org/10.1016/j.compositesb.2016.01.050
- Sarasini, F., Tirillò, J., Ferrante, L., Valente, M., Valente, T., Lampani, L., Gaudenzi, P., Cioffi, S., Iannace, S., & Sorrentino, L. (2014). Drop-weight impact behaviour of woven hybrid basalt-carbon/epoxy composites. Composites Part B: Engineering, 59, 204–220. https://doi.org/10.1016/j.compositesb.2013.12.006
- Sarasini, F., Tirillò, J., Valente, M., Ferrante, L., Cioffi, S., Iannace, S., & Sorrentino, L. (2013). Hybrid composites based on aramid and basalt woven fabrics: Impact damage modes and residual flexural properties. Materials & Design, 49, 290–302. https://doi.org/10.1016/j.matdes.2013.01.010
- Sarasini, F., Tirillò, J., Valente, M., Valente, T., Cioffi, S., Iannace, S., & Sorrentino, L. (2013). Effect of basalt fiber hybridization on the impact behavior under low impact velocity of glass/basalt woven fabric/epoxy resin composites. Composites Part A: Applied Science and Manufacturing, 47(1), 109–123. https://doi.org/10.1016/j.compositesa.2012.11.021
- Sebaey, T. A., & Wagih, A. (2019). Flexural properties of notched carbon – Aramid hybrid composite laminates. Journal of Composite Materials, 53(28-30), 4137–4148. https://doi.org/10.1177/0021998319855773
- Shaari, N., & Jumahat, A. (2017). Hole Size effects on the open hole tensile properties of woven kevlar-glass fibre hybrid composite laminates. Materialstoday: Proceedings, 25, 309–318.
- Standard (ASTM). (2011). D5766/D5766M-11 Standard test method for open-hole tensile strength of polymer matrix composite. American Society for Testing and Materials, 11(Reapproved), 1–7. https://doi.org/10.1520/D5766
- Subagia, I. D. G. A., Kim, Y., Tijing, L. D., Sang, C., & Kyong, H. (2014). Composites: Part B Effect of stacking sequence on the flexural properties of hybrid composites reinforced with carbon and basalt fibers. Composites Part B Engineering, 58, 251–258. https://doi.org/10.1016/j.compositesb.2013.10.027
- Sun, G., Wang, L., Chen, D., & Luo, Q. (2019). Tensile performance of basalt fiber composites with open circular holes and straight notches. International Journal of Mechanical Sciences, 176(August), 105517. https://doi.org/10.1016/j.ijmecsci.2020.105517
- Swolfs, Y., Gorbatikh, L., & Verpoest, I. (2014). Composites: Part A fibre hybridisation in polymer composites: A review. Composites Part A Applied Science and Manufacturing. 67, 181–200. https://doi.org/10.1016/j.compositesa.2014.08.027
- Vara, V., & Talupula, S. (2018). ScienceDirect A review on reinforcement of basalt and aramid (Kevlar 129) fibers. Materials Today: Proceedings, 5(2), 5993–5998. https://doi.org/10.1016/j.matpr.2017.12.202
- Vasudevan, A., Senthil Kumaran, S., Naresh, K., Velmurugan, R., & Shankar, K. (2018). Advanced 3D and 2D damage assessment of low velocity impact response of glass and Kevlar fiber reinforced epoxy hybrid composites. Advances in Materials and Processing Technologies, 4(3), 493–510. https://doi.org/10.1080/2374068X.2018.1465310
- Velumayil, R. (2022). Hybridization effect on mechanical properties of basalt/kevlar/epoxy composite laminates.
- Wang, X., Hu, B., Feng, Y., Liang, F., Mo, J., Xiong, J., & Qiu, Y. (2008). Low velocity impact properties of 3D woven basalt/aramid hybrid composites. Composites Science and Technology, 68(2), 444–450. https://doi.org/10.1016/j.compscitech.2007.06.016
- Wisnom, M. R., Khan, B., & Hallett, S. R. (2008). Size effects in unnotched tensile strength of unidirectional and quasi-isotropic carbon/epoxy composites. Composite Structures, 84(1), 21–28. https://doi.org/10.1016/j.compstruct.2007.06.002
- Zubair, M., & Pai, Y. (2019). Review on impact response of polymer composites. Journal of Mechanical Engineering Research and Developments, 42(4), 238–242. https://doi.org/10.26480/jmerd.04.2019.238.242