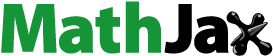
Abstract
Society is shifting to an environmental basis due to threats caused by climate change, resource scarcity, and excessive generation of plastics. Biotechnology has sought alternatives to valorise waste streams, such as sludge generated in municipal wastewater treatment plants (WWTPs). Sludge bio-fermentation is suitable for sustainable volatile fatty acids (VFA) production by anaerobic digestion (AD). This biotechnological process is an excellent opportunity, as VFAs are in high demand by industry. This work aimed to study the environmental impacts generated by the bioproduction of VFA from primary sludge (PS) from the El Salitre WWTP in Bogotá using the life cycle analysis (LCA) methodology. VFA production was carried out in three semi-continuous reactors, using PS, an initial organic load of 14 gVS/L, a temperature of 25 °C, and a pH of 10 for 7 and 16 d, obtaining a higher VFA production and yield for the 16-day experiment with 6048 mgCOD/L; 432 gCOD/gVS, compared to 5472 mgCOD/L; 391 gCOD/gVS produced in 7 d. An LCA was carried out to select the best retention time considering eighteen environmental categories. The study of the impact categories and the modelling of the inventory data were carried out using the ReCiPe Midpoint method and Simapro software. The results showed that energy use in the process steps is the bottleneck point for sustainability and causes significant effects in almost all the impact categories evaluated. Additionally, it was found that producing VFA from municipal WWTP sludge can be considered a sustainable process due to the indicators obtained being lower than those found in the literature. Also, the concentrations achieved allow the use of these VFAs as carbon sources for making polyhydroxyalkanoates.
Reviewing Editor:
1. Introduction
Environmental impact assessment is a management tool widely used by decision-makers to validate the sustainability of a production process (Duan et al., Citation2020). When evaluating new production processes using biotechnologies, it is crucial to carry out a life cycle analysis (LCA) that comprehensively analyses the impacts generated on the environment. It is, therefore, a fundamental methodology for environmental management. One of the advantages of using this method is that the production process can be evaluated, compared, and improved (Ingrao et al., Citation2019). To understand the environmental benefits and costs associated with biogas production processes, LCAs have been carried out in different research projects, allowing the benefits of developing these cleaner energy systems to be evaluated (Ruiz et al., Citation2018).
Detailed technical and economic assessments accompanied by a Life Cycle Assessment can explain why implementing new technologies generates better energy balances with lower environmental impacts and value-added products (Garfí et al., Citation2019). Studies to assess the ecological impacts of the production of volatile fatty acids, considering the independent variables and the substrates used as highly available residual biomass, such as sewage sludge, applying LCA to quantify the impacts of implementing biotechnologies in Colombia are scarce.
According to Elginoz et al. (Citation2020), the production of volatile fatty acids (VFA) by fermentation has been studied since the 1980s. However, the production of VFA in anaerobic reactors instead of biogas production is a relatively new concept for resource recovery, and according to the authors, the production of VFA from wastewater has not been previously studied using VFA. Similarly, Gálvez et al. (Citation2021), in the Life Cycle Analysis for the production process of volatile fatty acids from organic waste rich in proteins and carbohydrates, agree that the studies and literature related to LCA and VFA production systems are scarce.
On the other hand, a literature analysis in Scopus databases identified more than 900 studies related to Life Cycle Assessment to produce plastics; however, only 28 articles are oriented to LCA for the production processes of volatile fatty acids. Therefore, it is essential to identify and evaluate the different production processes oriented towards the circular economy where Life Cycle Assessments for bioprocesses are deepened and thus expand the information to analyse the technical, economic, and environmental feasibility of the production processes of VFAs Pinto et al. (Citation2023), which are also a source of carbon in the production of biopolymers such as polyhydroxyalkanoates.
However, previous studies such as Mosquera et al. (Citation2021) evaluated the life cycle of biogas production by anaerobic digestion using different substrates. Likewise, Amado et al. (Citation2021) analysed the life cycle of pig manure digestion through a process simulation. According to the LCA of waste-to-energy technologies review by Mayer et al. (Citation2019), many articles have been published in the last three decades on this topic, demonstrating the importance of applying LCA methodology in waste, energy, and value-added products.
There is a large availability of sludge from municipal wastewater treatment plants with a high potential for bio-fermentations that allow anaerobic digestion (AD) to take advantage of a large amount of biomass. Therefore, bioprocesses are one of the actions that make it possible to control the environmental impacts that generate pollution due to large volumes of waste and, simultaneously, to adapt to climate change through actions to mitigate greenhouse gases, promoting waste recovery. Considering the importance that this represents, it is necessary to evaluate the possible environmental impacts of the processes, which are developed through data obtained experimentally, generating mass and energy balances and environmental assessment through the methodology of LCA, as this allows the identification and quantification of the environmental impacts of the process from beginning to end.
This study seeks to determine the environmental viability of the bio-fermentation of primary sludge from the WWTP El Salitre-Bogotá, Colombia, for producing volatile fatty acids using the ISO 14040 LCA methodology.
1.1. Life cycle analysis LCA
Life cycle analysis (LCA) is a systematic method used to evaluate the environmental impacts of a product, process, or service over its entire life cycle. The life cycle includes all stages of a product or service, from raw material extraction, manufacturing, distribution, use, and end-of-life disposal (Mosquera et al., Citation2021). LCA was used to evaluate two scenarios for producing volatile fatty acids from wastewater sludge, which will be used as a carbon source in producing polyhydroxyalkanoates PHAs bioplastics.
As for the analysis of environmental impacts, the Life Cycle Assessment (LCA) methodology was used since it directly links the mass and energy balances with environmental impact, using a technological perspective approach of alternatives, where the potential environmental impacts of the proposed schemes were evaluated. ISO 14040 (International Organization for Standardization, Citation2006) was followed; this methodology consists of the following phases:
Definition of the scope: The type of information needed to develop the study, the necessary or acceptable accuracy of the results, and how they should be interpreted for the decision-making process were determined.
Definition of the objective: Application of a Life Cycle Assessment (LCA) to the proposed schemes under a technology foresight analysis for established scenarios, in which data and information obtained in a process simulation modeling will be used.
Scope of the study: The results obtained from the LCA will serve as information for technological and environmental decision-making in producing volatile fatty acids as renewable carbon sources.
Analysis of inventories of the study systems: The mass and energy balances were constructed based on the simulations of the evaluated schemes. The records of the simulated processes were entered into the specialized LCA software tool SimaPro, based on the designated functional unit.
Life cycle impact assessment: The impact categories, category indicators, and characterization models to be assessed and used were selected based on what is reported in the literature for this type of system. After this, the results of the Life Cycle Inventory (LCI) were assigned to the selected impact categories (classification), and the results of the category indicators (characterization) were calculated. This step was carried out using the commercial software tool SimaPro.
Interpretation of results: In this phase, the inventory analysis and impact assessment results are discussed to conclude the objectives and scope. Environmental burdens and points or aspects that can be improved were identified.
Overall, LCA is a valuable tool for identifying environmental impacts, reducing the environmental footprint of products and services, and promoting sustainable practices.
1.2. Volatile fatty acids – VFAs
Volatile fatty acids derived from Wastewater Treatment Plant Sludge can be exploited and marketed (Atasoy et al., Citation2018) thanks to its high demand worldwide due to its potential as a carbon source for the production of biopolymers, fuel sources, pharmaceuticals, and solvents (Gutiérrez et al., Citation2018), taking into account this background it is considered viable to evaluate the production of VFA in Colombia to give added value to the waste.
VFA is obtained by anaerobic fermentation, and this occurs in the second stage of this process, called acidogenesis (Zhou et al., Citation2018); there are different studies that report which is the best operating conditions for more excellent production of VFA and ways of recovering the hydrogen produced in the acetogenesis stage. This product can also be valued. Homoacetogenic and acetogenic bacteria produce VFAs through acidogenesis (Yuan et al., Citation2019). During these fermentations, butyric acid, acetic acid, propionic acid, and valeric acid, among other VFAs, are produced, as well as hydrogen (Khan et al., Citation2016).
In this work, primary sludge (PL) from the El Salitre-Bogotá WWTP is used to produce VFA as it is used as a carbon source for bacterial growth for the production of polyhydroxyalkanoates type bioplastics (Kumar et al., Citation2019) as a solution to reduce the production and consumption of fossil plastics.
On the other hand, the global market value of acetic acid, butyric acid, and propionic acid is 400–800 €/tonne, 1500–1650 €/tonne, and 2000–2500 €/tonne, respectively (Atasoy et al., Citation2018), significant sums for wastes that previously had no commercial value, another reason why VFA production should be optimized.
According to previous research, VFA production depends on operating conditions, substrate, pH, temperature, retention time, and organic load (Khan et al., Citation2016; Strazzera et al., Citation2018). These studies contribute to optimizing the production of VFA to be used as a carbon source and for hydrogen production. This biochemical process is used worldwide to stabilize sludge from municipal treatment plants, industrial water treatment, and for organic waste digestion.
2. Materials and methods
2.1. Experimental design
Acidogenic fermentation was carried out with three semi-continuous reactors (). With an initial organic load in the reactors of 14 gVS/L, they used primary sludge (PS) at 25 °C and an initial pH of 10. The substrate/inoculum (S/X) ratio was set at 1 gVS/gVS to reduce inhibitory effects during fermentation. Before the experiments, the inoculum from an anaerobic digester in a dairy plant near Bogotá was pre-treated with heat to avoid the methanogenesis activity: it was heated until 100° C for 1 h. The reactors were filled with the inoculum, the substrate necessary to establish the organic load, and the pH buffer. The working volume was topped up with distilled water until 4 L, and the reaction time was 7 and 16 d. To guarantee the pH, 200 mL of Hanna instruments pH 10 calibration buffer solution was added to each reactor. Finally, biogas productivity was controlled by taking samples every 48 h; biogas composition was measured with the BIOGAS 5000® Landtec, and pH stability was controlled in the automated plant by the pH sensors that are connected and monitored on display together with the temperature of each reactor and the VFA produced during the experiment were quantified.
For the quantification of VFAs, the fermentation supernatant was used, after separation by centrifugation and decantation.
2.2. Substrates characteristics
The fermentations were carried out using primary sludge (PS) from WWTP El Salitre in Bogotá as substrate and the stabilized sludge from the Alpina dairy industry reactor as inoculum. Following the methods of Gracia et al. (Citation2020). The pH measurements were determined using an Edge pH meter model HI2002. Total solids (TS), volatile solids (VS), and organic matter (OM) of the PS samples were determined by drying the samples at 105 ± 5 °C in a kiln and subsequent calcination at 550 ± 10 °C in a muffle according to 2540B APHA – SM and D3174 of the American Society for Testing and Materials (ASTM). Chemical Oxygen Demand (COD) was measured using commercial vials from Hanna Instruments with a range of 0 to 150 mg/L (HI 93752). Total Kjeldahl nitrogen (TKN) was assessed according to ASTM D1426. Volatile fatty acids (VFA) and alkalinity (ALK) were measured according to the standard 5560D method. The gas composition measurement (CO2, CH4, and O2%) was determined with the BIOGAS 5000® Landtec gas analyzer. The main physicochemical characteristics of the sludge are shown in . Before testing, the sludge and inoculum were kept in a freezer at −4 °C to avoid microbiological degradation.
Table 1. Physico-chemical characteristics of inoculum and primary domestic wastewater sludge.
2.3. Life cycle assessment methodology
The LCA methodology established by the International Organization for Standardization ISO 14040 was applied to assess fermentation’s life cycle and potential environmental impacts to produce Volatile Fatty Acids using primary sludge (PS) from the WWTP. The weighted environmental impact calculation method was ReCiPe 2016 Midpoint (H) V1.08/World (2010) H. The ReCipe method and the 18 midpoint categories were used because they are the most used in LCA studies due to the uncertainty associated with endpoint impact categories (Karolinczak et al., Citation2024; Ferdous et al., Citation2023). ReCipe is a method for LCA impact assessment that translates emissions and resource extractions into environmental impact scores using characterisation factors (Villanova et al., Citation2023).
The inventory data were taken from the mass and energy balances of the proposed experiments. In contrast, the information on substrate availability and chemicals was taken from the Ecoinvent databases. It was assumed that the plant would have an average operating capacity of 80%, considering a semi-continuous process with a hydraulic retention time of 7 and 16 d. The system boundary includes the delivery of the substrates, the mechanical pre-treatment, and the Anaerobic Digestion process where the VFAs will be produced. The above is to evaluate a sustainable alternative for producing VFAs through sludge biomass from wastewater treatment plants (Pöschl et al., Citation2010).
The energy balance for the reactors is defined by EquationEquation (1)(1)
(1) .
(1)
(1)
Treac is the internal temperature of the reactor in °C; Tamb is the ambient temperature in °C; c is the reactor liquid heat capacity; – p is the density of the reactor liquid; – V is the volume of the reactor, G is the denominator conductivity given in
Sampling was conducted with Δt = 10 s or, equivalently, Δt = 0.0001157 d to obtain data from the system. The ambient temperature, reactor power, and reactor temperature were taken in each sample.
3. Results and discussion
3.1. Results of the life-cycle assessment
The LCA performed started with defining the process steps according to the results obtained during the fermentations and the corresponding assumptions.
3.1.1. Process design and construction of mass and energy balances for inventory analysis
A full-scale scenario was constructed based on the results of the Anaerobic Digestion experiments for VFA production. The mass and energy flow diagrams are shown in . In addition, VFA production through residual fermentations is defined under the conditions described in Section 2.1 for 7 and 16 d of production.
Figure 2. (a) Mass and energy flow diagram VFA production 7 d. (b) Mass and energy flow diagram VFA production 16 d.

The production and yield of VFA in the acidogenic fermentation after 16 d was 6048 mgCOD/L and 432 gCOD/gVS, respectively, while in 7 d, with slightly lower results, 5472 mgCOD/L and a yield of 391 gCOD/gVS were produced.
3.2. Comparative analysis by impact category using simapro
To perform a comparative analysis of the characterization of the leading environmental impacts generated when producing 1 kg of VFA from the fermentation of volatile fatty acids, the ReCiPe 2016 Method was applied. The main impacts are water consumption, ionizing radiation, land use, marine eutrophication, terrestrial ecotoxicity, and associated ozone and health impacts. See .
Considering all the impact categories analysed by SIMAPRO, the impact is positive for the 7-day VFA production system from sewage sludge related to the 16-day (Bacenetti et al., Citation2016; Rigon et al., Citation2019). The main result of this part of the study is to obtain the values corresponding to each category of impact on the production of 1 kg of volatile fatty acids; in this way, it is possible to identify the categories of environmental impacts with greater relevance and those impacts generated to a lesser extent.
Comparing the production process over 7 and 16 d, there is evidence of more significant environmental impacts in the 16-day production process because of longer equipment running time and higher energy consumption. The environmental impact of water consumption is explained because the process analysed was with semi-continuous reactors, using tap water. However, in a scaling process this water must be replaced by wastewater from the treatment plant to reduce this impact. shows the comparative results in actual units for all environmental impact categories assessed under the ReCiPe 2016 method.
Table 2. Environmental impacts by impact category to produce VFAs over 16 and 7 days.
In this way and by what is proposed by Elginoz et al. (Citation2020) in the Life Cycle Analysis for the production process of VFA from wastewater from dairy industries for five days, it is identified that electricity consumption for mixing and heating is the main contributor to all impact categories. Thus, the reactor agitation processes and the addition of substrate are the phases that contribute most to the impacts generated since this is where the most significant energy demand is implemented, which coincides with the results of this study.
Pinto et al. (Citation2023) defined that the environmental impacts generated are significantly influenced by the yield for obtaining Volatile Fatty Acids, so it is essential to identify the processes that achieve a higher yield, which will reflect lower environmental impacts generated. However, the circular economy-oriented scenarios, as assessed in their study, lead to a decrease in the impact of energy resource depletion because alternative methods of waste treatment consume more energy than other methods.
3.3. Inventory data modeling using SimaPro software
Environmental impacts were assessed with the Recipe method. In terms of environmental impacts per process for producing 1 kg of VFA, the environmental impact of natural gas and coal prevails in the analysis of fossil resources. The most significant contribution to the process analysis of fossil resources can be identified in the use of electricity and water. On the other hand, the contribution of greenhouse gases from digestion is carbon dioxide, and the impact is generated in the electricity needed to operate the reactor. As shown in .
Figure 4. (a) Fossil resources to produce 1 kg of VFAs in 16 d. (b) Fossil resources to produce 1 kg of VFAs in 7 d.

Figure 5. (a) Contribution per process to fossil resource VFAs 16 d. (b) Contribution per process to fossil resource VFAs 7 d.

Figure 6. (a) Global warming to produce 1 kg of VFAs in 16 d. (b) Global warming to produce 1 kg of VFAs in 7 d.

Figure 7. (a) Contribution per process to global warming VFAs 16 d. (b) Contribution per process to global warming VFAs 7 d.

It is worth noting that CO2 production is the most produced gas in the global warming category, representing 92% of the category for the two production periods, 0.1705 and 0.0815 kg CO2-eq, which compared to the results obtained by Elginoz et al. (Citation2020) and Chen et al. (Citation2017) who performed LCA for the production of Volatile Fatty Acids from dairy wastewater and from food waste, a total of 6.03 kg CO2 -eq and 5 kg CO2-eq respectively were produced in the global warming category, the impacts of this study are considerably low. It is vital to note that the results of these studies include plant construction and the VFA purification steps.
In addition to the above, it is worth mentioning that the waste generated at the end of the operation is fully reusable, as the liquid fraction of the digestate containing VFAs is used as a carbon source to produce polyhydroxyalkanoates. In this sense, the valorisation of waste (Cusenza et al., Citation2021; Fusi et al., Citation2016; Mosquera et al., Citation2021; Sadhukhan et al., Citation2021) from wastewater treatment plant sludge avoids environmental impacts and generates a positive environmental effect in two ways, on the one hand.
Comparing the results of this study with those reported by Mosquera et al. (Citation2021) of the production in the same comparison periods of 16 and 7 d of the gases hydrogen sulphide, methane, atmospheric nitrogen, and carbon dioxide, it can be validated that the results for 16 d of the present research are superior to those of Mosquera et al., but in the opposite case, the production of greenhouse gases for a production of 7 d is lower in the process developed in this contribution than the emissions into the air during the biogas production process developed by Mosquera et al. This confirms that the most sustainable production is carried out in 7 d. According to the life cycle analysis for producing 1 kg of VFA in 7 and 16 d, it was assessed that to realize a more sustainable biotechnological process, the acidogenesis for obtaining VFA should be realized in 7 d, as a reasonable amount is produced and with a smaller ecological footprint compared to 16 d. Therefore, the conditions for optimizing the process need to be studied further.
The production of VFA for this study is determined in two different periods; on the one hand, the 7-day production process shows that the results for the Life Cycle Assessment for the environmental impact categories are low concerning the 16-day production process. However, it is identified that the impacts generated are more significant than those presented by Mosquera et al. (Citation2021); this may be due to the continuous and prolonged energy consumption to make the production process more efficient for obtaining VFA.
According to Dahiya et al. (Citation2023), the literature has limited Life Cycle Assessment for VFA production. However, VFA production from waste is considered an innovative waste management option, as studies have shown that these options have a lower environmental impact than conventional waste management technologies. In addition, LCA studies for VFA production have several limitations, such as considering laboratory and large-scale efficiency and using the type and amount of inoculum used at both scales, data availability, and methodological considerations.
A comparative analysis of results obtained by other authors about VFA production was performed, summarised in .
Table 3. Comparison of LCA results of VFAs with other studies.
presents the results of studies by Tong et al. (Citation2018), who performed LCA for anaerobic digestion, composting, incineration, and gasification from food waste generated in restaurants in Singapore. Jin et al. (Citation2015) evaluated the LCA for anaerobic digestion from food waste, while Di Maria and Micale (Citation2014) performed the LCA analysis for Anaerobic Digestion from municipal solid waste. In the same line, Elginoz et al. (Citation2020) presented the LCA to produce VFA from food waste, and Xu et al. (Citation2015) elaborated the LCA for the anaerobic digestion process from food waste on the one hand and from food waste with sludge on the other hand. Gálvez et al. (Citation2021) presented the life cycle analysis for Volatile Fatty Acids obtained from different substrates, specifically from food waste and microalgae, and in turn, the LCA of these products with the implementation of composting as a treatment. As Katakojwala and Mohan (Citation2020) proposed, they elaborated the LCA to produce microcrystalline cellulose from bagasse using three processes to compare their environmental impacts.
It was identified that among other VFA production processes, the category Ecotoxicity in freshwater has the highest impact for the 16-day VFA production process. On the other hand, the VFA production process in 7 d shows a lower impact in the categories of Land use, Water consumption, Scarcity of mineral resources, Marine eutrophication, and Depletion of stratospheric ozone.
Considering the above, it was quantitatively analysed that the greater the number of days to produce VFA, the greater the impact generated, and the impact with the most significant variation corresponds to the category of Ecotoxicity in fresh water, followed by Marine Ecotoxicity and Non-Carcinogenic Human Toxicity with values of 127%, 126%, and 119% respectively. For this analysis, it should be noted that a 100% variation corresponds to a doubling of the value obtained from the 7-day LCA. Thus, the optimum time for the production process to reduce environmental impacts is 7 d for environmental sustainability and economic competitiveness.
Additionally, it was identified that the life cycle analyses carried out by different authors do not include all the environmental impact categories (see ), mainly focusing on some categories such as global warming, terrestrial acidification, and those related to human health, which makes this study a novelty in the field of carrying out a complete and multi-criteria LCA assessment, carrying out an outstanding environmental analysis among the research that has already been carried out.
On the other hand, the production of PHAs that have been evaluated, to a greater extent, have focused on productive processes from agricultural waste or rich in organic matter such as food waste; this study considers using as raw material the primary sludge resulting from a Wastewater Treatment Plant (PTAR Salitre) may represent a possible solution or alternative management of sewage sludge.
Likewise, the research on the environmental issue tends to carry out the production analysis with its environmental impacts, leaving aside the issue of economic competitiveness compared to other production processes that may be more economical but, at the same time, more polluting. This means that the study conducted when considering the environmental advantages and evaluating the economic competitiveness compared to conventional plastic is innovative when making decisions, as it presents a multi-criteria evaluation, making it a complete study.
In addition, it is possible to identify that the research carried out for the production of PHAs and those related to the Life Cycle Analysis of a productive process are mainly carried out by authors located in European and Asian countries or developed in the United States, showing a deficiency in the development of research on these topics in Latin American countries, which is why this study can stand out at a local and regional level in Latin America.
Finally, to mitigate the CO2 emissions generated, it is necessary to improve the energy system by switching from an ON/OFF system to a continuous power system, which will reduce these emissions. Generally, a constant power system tends to be more efficient regarding energy savings than an ON/OFF system. Continuous systems are designed to run constantly and are more energy efficient than repeatedly switched on and off. When an ON/OFF system is used, each time the equipment is turned on, there may be a transient increase in energy demand, resulting in additional consumption. In addition, frequent on/off cycling can result in energy losses due to inefficient start-up processes and periods when the equipment is in standby mode.
5. Conclusions
As attested by numerous studies analyzed in the present paper, VFA synthesis through fermentation is an environmentally friendly process. The results presented in this work demonstrate that sludge generated in WWTPs in developing countries could be used as a feedstock to produce value-added products and improve these processes’ environmental and economic sustainability. This research delivered biobased VFAs under different operational parameters, using primary sludge and mixed microbial cultures as substrates for VFA production. However, many challenges must be addressed to make VFA production and recovery from waste streams a sustainable option for carbon recovery. By comparing the results obtained in the production process over 7 and 16 d, there is evidence of more significant environmental impacts in the 16-day production process because of longer equipment running time and higher energy consumption.
The primary contribution of greenhouse gases from digestion is carbon dioxide, and the impact is generated in the electricity needed to operate the reactor. It is identified that the environmental impacts of this type of process are influenced to a greater extent and almost entirely by electricity consumption, i.e. to achieve sustainability and mitigate the impacts, it is necessary to evaluate alternatives or different energy sources such as solar or wind as mentioned above, this to identify the most sustainable production process for obtaining PHAs. The most sustainable production occurs in 7 d. According to the life cycle analysis for producing 1 kg of VFA in 7 and 16 d, it was assessed that to realize a more sustainable biotechnological process, the acidogenesis for obtaining VFA should be made in 7 d, as a reasonable amount is produced and with a smaller ecological footprint compared to 16 d. Therefore, the conditions for optimizing the process need to be studied further.
Finally, it should be noted that the LCA has considered different impact categories, which can lead to a complete analysis of the sustainability of the production process, unlike some research where the LCA focuses mainly on categories such as global warming and soil acidification, ignoring the other impacts that can be generated on water, air, soil and even on biodiversity and human health.
Acknowledgments
The authors are grateful for the funding obtained by the cooperative research project between the Universidad Cooperativa de Colombia and the Universidad Distrital Francisco José de Caldas, ‘Evaluation of the potential for obtaining PHAs from wastewater and mixed crops at pilot scale.’ ID: INV2600-2020. To the Minciencias project - EcosNord No. 80740-225-2021, ‘Sustainable biorefinery schemes for energy, materials and nutrients recovery.’ To the facilities of the biotechnology laboratory of the Universidad Cooperativa de Colombia. Colombian Institute of Educational Credit and Technical Studies Abroad ICETEX and the Ministry of Science, Technology, and Innovation MINCIENCIAS, for funding this research (Project number 2021-1016 of 2021). Laboratory of Energy, Materials and Environment of the Universidad de la Sabana for the support in the frame of project number INGPHD-50-2022 (Energy, Materials and Environment research group).
Data availability statement
The authors confirm the statement of data availability.
Disclosure statement
No potential conflict of interest was reported by the author(s).
Additional information
Funding
Notes on contributors
Jeniffer Gracia
Jeniffer Paola Gracia Rojas, PhD in Engineering, Master in Sustainable Development and Environmental Management, Environmental Administrator. Professor at the Faculty of Environment and Natural Resources of the Francisco José de Caldas District University, 15 years of experience in university teaching for undergraduate and graduate programs. With research and publications in the field of environment and biotechnology, recognition by Minciencias as an Associate Researcher. Academic peer National Accreditation Council of the Ministry of National Education.
Iván Cabeza
Iván Cabeza is an Associate Professor in the Department of Chemical Processes and Biotechnology at the Universidad de La Sabana (Colombia). He holds a degree in Chemical Engineering from the Universidad Industrial de Santander (Colombia), a Master’s degree in Renewable Energy from the Universidad de León (Spain) and a Ph.D. in Natural Resources and Environment from the Universidad de Sevilla (Spain). Dr Cabeza also has postdoctoral experience in biotechnology from the Universidad Nacional de Colombia. His research interests include bioprocesses, renewable energy and biorefinery.
Paola Acevedo
Paola Acevedo is Director of Chemical Engineering - EAN University, Chemical Engineer with a PhD in Chemical Engineering. She has excellent training in the management and development of chemical and physicochemical processes in industry and research. She has extensive knowledge in specific areas such as: sustainable development, economic evaluation, biofuels, bioprocesses, life cycle analysis, technology transfer, process safety and process simulation. She also has skills and experience in teaching, research.
References
- Amado, M., Carrasco, J., Ochoa, L. D., Rangel, C. J., Becerra, A. P., Cabeza, I. O., & Acevedo, P. A. (2021). Technical and environmental analysis of large-scale pig manure digestion through process simulation and life cycle assessment. Chemical Engineering Transactions, 87, 439–444. https://doi.org/10.3303/CET2187074
- Atasoy, M., Owusu-Agyeman, I., Plaza, E., & Cetecioglu, Z. (2018). Bio-based volatile fatty acid production and recovery from waste streams: Current status and future challenges. Bioresource Technology, 268, 773–786. https://doi.org/10.1016/j.biortech.2018.07.042
- Bacenetti, J., Sala, C., Fusi, A., & Fiala, M. (2016). Agricultural anaerobic digestion plants: What LCA studies pointed out and what can be done to make them more environmentally sustainable. Applied Energy, 179(1), 669–686. https://doi.org/10.1016/j.apenergy.2016.07.029
- Chen, Y., Jiang, X., Xiao, K., Shen, N., Zeng, R. J., & Zhou, Y. (2017). Enhanced volatile fatty acids (VFAs) production in a thermophilic fermenter with stepwise pH increase - Investigation on dissolved organic matter transformation and microbial community shift. Water Res., 112, 261–268. https://doi.org/10.1016/j.watres.2017.01.067
- Cusenza, M. A., Longo, S., Guarino, F., & Cellura, M. (2021). Energy and environmental assessment of residual bio-wastes management strategies. Journal of Cleaner Production, 285(20), 124815. https://doi.org/10.1016/j.jclepro.2020.124815
- Dahiya, S., Lingam, Y., & Mohan, V. (2023). Understanding acidogenesis towards green hydrogen and volatile fatty acid production – Critical analysis and circular economy perspective. Chemical Engineering Journal, 464, 141550. https://doi.org/10.1016/j.cej.2023.141550
- Di Maria, F., & Micale, C. (2014). Life cycle analysis of incineration compared to anaerobic digestion followed by composting for managing organic waste: the influence of system components for an Italian district. The International Journal of Life Cycle Assessment, 20(3), 377–388. https://doi.org/10.1007/s11367-014-0833-z
- Duan, N., Khoshnevisan, B., Lin, C., Liu, Z., & Liu, H. (2020). Life cycle assessment of anaerobic digestion of pig manure coupled with different digestate treatment technologies. Environment International, 137, 105522. https://doi.org/10.1016/j.envint.2020.105522
- Elginoz, N., Atasoy, M., Finnveden, G., & Cetecioglu, Z. (2020). Ex-ante life cycle assessment of volatile fatty acid production from dairy wastewater. Journal of Cleaner Production, 269, 122267. https://doi.org/10.1016/j.jclepro.2020.122267
- Elginoz, N., Khatami, K., Owusu-Agyeman, I., & Cetecioglu, Z. (2020). Life cycle assessment of an innovative food waste management system. Frontiers in Sustainable Food Systems, 4, 23. https://doi.org/10.3389/fsufs.2020.00023
- Ferdous, J., Bensebaa, F., & Pelletier, N. (2023). Integration of LCA, TEA, process simulation and optimization: A systematic review of current practices and scope to propose a framework for pulse processing pathways. Journal of Cleaner Production, 402, 136804. https://doi.org/10.1016/j.jclepro.2023.136804
- Fusi, A., Bacenetti, J., Fiala, M., & Azapagic, A. (2016). Life cycle environmental impacts of electricity from biogas produced by anaerobic digestion. Frontiers in Bioengineering and Biotechnology, 4, 26. https://doi.org/10.3389/fbioe.2016.00026
- Gálvez, J., Greses, S., Magdalena, J.-A., Iribarren, D., Tomás-Pejó, E., & González-Fernández, C. (2021). Life cycle assessment of volatile fatty acids production from protein- and carbohydrate-rich organic wastes. Bioresource Technology, 321, 124528. https://doi.org/10.1016/j.biortech.2020.124528
- Garfí, M., Castro, L., Montero, N., Escalante, H., & Ferrer, I. (2019). Evaluating environmental benefits of low-cost biogas digesters in small-scale farms in Colombia: A life cycle assessment. Bioresource Technology, 274, 541–548. https://doi.org/10.1016/j.biortech.2018.12.007
- Gracia, J., Mosquera, J., Montenegro, C., Acevedo, P., & Cabeza, C. (2020). Volatile fatty acids production from fermentation of waste activated sludge. Chemical Engineering Transactions, 79, 217–222. https://doi.org/10.3303/CET2079037
- Gutiérrez, E., Garcia-Aguirre, J., Irizar, I., & Aymerich, E. (2018). From sewage sludge and agri-food waste to VFA: Individual acid production potential and up-scaling. Waste Management (New York, N.Y.), 77, 203–212. https://doi.org/10.1016/j.wasman.2018.05.027
- Ingrao, C., Bacenetti, J., Adamczyk, J., Ferrante, V., Messineo, A., & Huisingh, D. (2019). Investigating energy and environmental issues of agro-biogas derived energy systems: A comprehensive review of life cycle assessments. Renewable Energy. 136, 296–307. https://doi.org/10.1016/j.renene.2019.01.023
- International Organization for Standardization. (2006). ISO 14040 - Environmental management—Life cycle assessment—Principles and framework. International Organization for Standardization.
- Jin, Y., Chen, T., Chen, X., & Yu, Z. (2015). Life-cycle assessment of energy consumption and environmental impact of an integrated food waste-based biogas plant. Applied Energy, 151, 227–236. https://doi.org/10.1016/j.apenergy.2015.04.058
- Karolinczak, B., Walczak, J., Bogacka, M., & Zubrowska-Sudol, M. (2024). Life cycle assessment of sewage sludge mono-digestion and co-digestion with the organic fraction of municipal solid waste at a wastewater treatment plant. The Science of the Total Environment, 907, 167801. https://doi.org/10.1016/j.scitotenv.2023.167801
- Katakojwala, R., & Mohan, S. V. (2020). Microcrystalline cellulose production from sugarcane bagasse: Sustainable process development and life cycle assessment. Journal of Cleaner Production, 249, 119342. https://doi.org/10.1016/j.jclepro.2019.119342
- Khan, M. A., Ngo, H. H., Guo, W. S., Liu, Y., Nghiem, L. D., Hai, F. I., Deng, L. J., Wang, J., & Wu, Y. (2016). Optimization of process parameters for production of volatile fatty acid, biohydrogen and methane from anaerobic digestion. Bioresource Technology, 219, 738–748. https://doi.org/10.1016/j.biortech.2016.08.073
- Kumar, G., Ponnusamy, V. K., Bhosale, R. R., Shobana, S., Yoon, J., Bhatia, S. K., Rajesh Banu, J., & Kim, S. (2019). A review on the conversion of volatile fatty acids to polyhydroxyalkanoates. Bioresource Technology, 287, 121427. https://doi.org/10.1016/j.biortech.2019.121427
- Mayer, F., Bhandari, R., & Gäth, S. (2019). Critical review on life cycle assessment of conventional and innovative waste-to-energy technologies. The Science of the Total Environment, 672, 708–721. https://doi.org/10.1016/j.scitotenv.2019.03.449
- Mosquera, J., Rangel, C., Thomas, J., Santis, A., Acevedo, P., & Cabeza, I. (2021). Biogas production by pilot-scale anaerobic co-digestion and life cycle assessment using a real scale scenario: Independent parameters and co-substrates influence. Processes, 9(11), 1875. https://doi.org/10.3390/pr9111875
- Pinto, A. S. S., McDonald, L. J., Jones, R. J., Massanet-Nicolau, J., Guwy, A., & McManus, M. (2023). Production of volatile fatty acids by anaerobic digestion of biowastes: Techno-economic and life cycle assessments. Bioresource Technology, 388, 129726. https://doi.org/10.1016/j.biortech.2023.129726
- Pöschl, M., Ward, S., & Owende, P. (2010). Evaluation of energy efficiency of various biogas production and utilization pathways. Applied Energy, 87(11), 3305–3321. https://doi.org/10.1016/j.apenergy.2010.05.011
- Rigon, M. R., Zortea, R., Moraes, C. A. M., & Modolo, R. C. E. (2019). Suggestion of life cycle impact assessment methodology: Selection criteria for environmental impact categories. In A. Petrillo and F. De Felice (Eds.), New frontiers on life cycle assessment—Theory and application. IntechOpen.
- Ruiz, D., Miguel, G. S., Corona, B., Gaitero, A., & Domínguez, A. (2018). Environmental and economic analysis of power generation in a thermophilic biogas plant. The Science of the Total Environment, 633(15), 1418–1428. https://doi.org/10.1016/j.scitotenv.2018.03.169
- Sadhukhan, J., Sen, S., & Gadkari, S. (2021). The mathematics of life cycle sustainability assessment. Journal of Cleaner Production, 309(1), 127457. https://doi.org/10.1016/j.jclepro.2021.127457
- Strazzera, G., Battista, F., Garcia, N., Frison, N., & Bolzonella, D. (2018). Volatile fatty acids production from food wastes for biorefinery platforms: A review. Journal of Environmental Management, 226, . 78–288. https://doi.org/10.1016/j.jenvman.2018.08.039
- Tong, H., Shen, Y., Zhang, J., Wang, C.-H., Shu Ge, T., & Wah Tong, Y. (2018). A comparative life cycle assessment on four waste-to-energy scenarios for food waste generated in eateries. Applied Energy, 225, 1143–1157. https://doi.org/10.1016/j.apenergy.2018.05.062
- Villanova, R., Murcia, D. A., Correa, A., López, G., Hernández, P., Gávara, R., & Navas, L. M. (2023). Environmental consequences of shelf life extension: Conventional versus active packaging for fresh-cut salads. Agronomy, 13(11), 2749. https://doi.org/10.3390/agronomy13112749
- Xu, C., Shi, W., Hong, J., Zhang, F., & Chen, w (2015). Life cycle assessment of food waste-based biogas generation. Renewable and Sustainable Energy Reviews, 49, 169–177. https://doi.org/10.1016/j.rser.2015.04.164
- Yuan, Y., Hu, X., Chen, H., Zhou, Y., Zhou, Y., & Wang, D. (2019). Advances in enhanced volatile fatty acid production from anaerobic fermentation of waste-activated sludge. The Science of the Total Environment, 694, 133741. https://doi.org/10.1016/j.scitotenv.2019.133741
- Zhou, M., Yan, B., Wong, J., & Zhang, Y. (2018). Enhanced volatile fatty acids production from anaerobic fermentation of food waste: A mini-review focusing on acidogenic metabolic pathways. Bioresource Technology, 248(Pt A), 68–78. https://doi.org/10.1016/j.biortech.2017.06.121