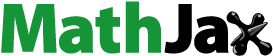
Abstract
Fiber-reinforced polymer composites (FRPs) experience exposure to diverse environments throughout their intended design life. Investigating the aging process of FRPs immersed in water is crucial for enhancing the material’s durability. In this study, glass fiber, bamboo fiber, nanoclay and epoxy composites are produced using the hand lay-up process. Tensile and flexural tests are performed in adherence to ASTM standards. The water uptake percentage of soaked specimens is computed, and a comparison is drawn between the results of dry and water-immersed composite specimens. Water-soaking condition negatively affects all the composites. Water-soaked specimens exhibit lesser tensile and flexural strengths than dry specimens by 7.4–14.8% and 7–13.6%, respectively. The addition of nanoclay further improves the tensile and flexural strengths of epoxy and all composites by 6–11%. Water uptake (%) and water-soaking effects on the strength of composites decline when nanoclay is added. The addition of nanoclay declines the percentage of reduction of tensile strength from 8.6–14.8% to 7.4–12%. Similarly, the percentage of flexural strength reduction declines from 8–13.6% to 7–11%. The dry and water-soaked specimens’ fracture surfaces (Scanning Electron Microscope [SEM] images) show significant variations.
1. Introduction
Natural fiber-reinforced polymer composites (NFPCs) aim to assimilate the characteristics of natural fibers with the functional and structural enhancements obtainable by the polymer. The outcome of the resulting composite materials is strength, stiffness and lightweight properties. Natural fibers, viz., jute (Arya et al., Citation2024), flax (Agopian et al., Citation2023), hemp (Song et al., Citation2024), sisal (Olhan et al., Citation2023) and bamboo (Kaima et al., Citation2023), present numerous benefits, such as affordability, biodegradability and high specific strength. These fibers show improved mechanical properties when combined with polymer matrices, whether thermoplastics or thermosets, making them appropriate for numerous applications. A unique benefit of NFPCs is eco-friendliness (Gholampour & Ozbakkaloglu, Citation2020). Natural fibers are biodegradable and reduce the environmental effects compared to synthetic fibers (Vigneshwaran et al., Citation2020). NFPCs can be used in industries like automotive (Verma et al., Citation2019; Singh, Citation2021) and construction (Silva et al., Citation2020). In the automotive industry, these composites are utilized in manufacturing lightweight components, thereby improving the fuel efficiency of vehicles. Also, NFPCs help as structural elements, providing strength and sustainability in the construction industry. NFPCs face obstacles like moisture absorption, restricted thermal stability and variability. The recent research focuses on better processing methods, creating new composites and studying advanced natural fibers. These efforts aim to overcome limitations of natural fibers and to understand the complete potential of NFPCs across several industries (Batu & Lemu, Citation2020).
Investigating water absorption in composites is crucial in materials engineering, focusing on the interaction between composite materials and water to estimate their performance and durability. This process involves water infiltration into composite structures, potentially causing changes in properties, dimensional stability and overall structural integrity (Musthaq et al., Citation2023). Understanding water soaking in composites is essential for predicting the long-term behavior of composite materials in the automotive and construction industries. This investigation aims to uncover the consequences of water exposure on composites, providing valuable insights to enhance material design and address potential issues arising from water-induced damage. Researchers are committed to developing strategies that mitigate the impact of water soaking, advancing the field and ensuring optimal performance of composite structures in diverse environmental conditions (Kini et al., Citation2018; Avci et al., Citation2023; Shettar et al., Citation2020). The different strategies are surface treatment of natural fibers, hybridization, and matrix modification by incorporating fillers/additives.
Saada et al. (Citation2024) assessed the tensile strength of palm fibers – epoxy composites. Both untreated and treated with sodium carbonate fibers are used to prepare the composites. Fiber treatment enhances the tensile strength of composites. Natural fibers’ surface treatment aims to modify the fiber’s surface properties to make it more hydrophobic, reducing its affinity for water and thus lowering water absorption (Ramachandran et al., Citation2022). NaOH solution treatment alters the surface chemistry of natural fiber and reduces the presence of hydrophilic groups. This process results in decreased water absorption (Herlina Sari et al., Citation2017). Rajeshkumar (Citation2020) investigated the effect of NaOH treatment of Phoenix sp. fiber on water absorption of Phoenix sp. fiber-epoxy composites. Results show that NaOH treatment substantially reduces the moisture sorption of composites by improving interfacial bonding. Scanning Electron Microscope (SEM) analysis reveals NaOH treatment improves the composite’s interfacial bonding and mechanical properties.
Hybridization includes adding different reinforcing materials into a combined structure to increase material properties (He et al., Citation2022). This method combines various fibers or particles with distinct mechanical, thermal or chemical characteristics to modify the composite’s properties for specific performance needs (Abd El-Baky et al., Citation2020). Introducing hydrophobic synthetic fibers, such as glass or aramid fibers, into the composite improves moisture resistance (Feng Ng, Citation2022). Zhang et al. (Citation2021) investigated the effect of bamboo and glass fiber hybridization on Benzoxazine resin-based composites’ water absorption and mechanical properties. At 25 °C, the saturated moisture content of bamboo fiber and glass-bamboo fiber hybrid composites are recorded as 3.64% and 3.11%, respectively. The hybrid composites exhibit the maximum mechanical properties under dry and water-saturated conditions. Hence, hybridization could significantly improve the composites’ mechanical properties and water absorption resistance.
Al-Jumaili et al. (Citation2023) evaluated the mechanical properties of low-density polyethylene (LDPE) – nanoclay nanocomposites. Adding 1 and 2 wt.% nanoclay to LDPE improves tensile strength. Incorporating nanoclay into a matrix offers a multifaceted approach to enhancing moisture resistance, including creating a physical barrier, modifying surface properties, reducing swelling, improving mechanical properties and enhancing filler-matrix compatibility (Kenned et al., Citation2021; Jawaid et al., Citation2022). These advantages make nanoclay a valuable additive for developing polymer composites with superior resistance to moisture absorption and improved performance in various applications, including those exposed to humid or wet environments (Bulut et al., Citation2020). Suresh et al. (Citation2023), explored the impact of nanoclay on polyester resin’s water absorption and mechanical properties. Results show that incorporating nanoclay into the polyester resin reduces the water absorption percentage. Also, nanoclay addition enhances mechanical properties and improves the mechanical properties’ retention rate under water absorption conditions.
The effects of combining glass fibers, bamboo fibers and nanoclay on the mechanical properties of epoxy-based composites are not well documented in the literature. This element appears to have significant consequences for expanding the possible applications of FRPs, especially in the construction and automotive industries. Also, investigating the aging process of FRPs immersed in water is crucial for enhancing the material’s durability. This work aims to investigate the impact of water soaking on the mechanical properties of bamboo, glass fiber and hybrid epoxy-based composites. This work also documents comparing tensile and flexural strength of dry and water-soaked composites. Additionally, the influence of nanoclay on the water soaking and mechanical properties of bamboo, glass fiber and hybrid epoxy-based composites is presented in this work. Fracture surfaces dry and water-soaked specimens under tensile load are assessed to find the reasons for failure.
2. Methodology
2.1. Materials
‘Atul Polymers’ provides epoxy resin (L-12) and hardener (K-6) in a 10:1 ratio to mix. The epoxy, a liquid resin with an intermediate viscosity ranging from 9000 to 12,000 mPa.s, remains unchanged. The K-6 hardener, characterized by lower viscosity, cures at room temperature. ‘Yuje Enterprises, Bengaluru’ supplies glass fiber reels of 360 GSM, featuring bi-directional orientation. Simultaneously, ‘Shreenath Weaving Industries, Gandhinagar, Gujarat’, furnishes a bi-directional woven bamboo fiber 150 GSM reel. ‘Sigma Aldrich’ delivers surface-modified nanoclay exhibiting a ‘sheet-like’ structure with lateral dimensions varying from 200 to 600 nm and a few nanometers thick.
2.2. Composites preparation
The fabrication of epoxy and epoxy-nanoclay composite samples is carried out using a conventional casting technique. Furthermore, bamboo/glass fiber epoxy and hybrid composite laminates are produced using the hand lay-up method, followed by compression molding. After a 24-h curing period at room temperature, following ASTM standards, the laminates are cut into specimens. The specific procedures for laminate preparation are detailed in . Two sets of laminates are fabricated, with and without nanoclay, as specified in and . The process begins with incorporating nanoclay into the matrix material to achieve a uniform dispersion of nanoclay throughout the matrix. Subsequently, magnetic stirring and sonication are employed to break down agglomerates and ensure a homogeneous distribution of the nanoclay particles within the matrix. NaOH-treated bamboo fibers are employed to fabricate the composites. Bamboo fibers are treated with 5 wt.% NaOH solution for 1 h at room temperature. The mass ratio of solution and bamboo fiber is maintained at 30:1. The detailed procedure for the treatment of bamboo fiber is presented in our previous work (Ahmad et al., Citation2023). The specimens are dipped in tap water at ambient temperature, and their weight is observed weekly to assess the water uptake percentage. This study thoroughly compares the mechanical properties of dry specimens with those subjected to water immersion for all the prepared composites.
Table 1. Composite material’s composition (without nanoclay) and notations.
Table 2. Composite material’s composition (with nanoclay) and notations.
2.3. Mechanical characterization of composites
2.3.1. Tensile test
The ZWICK ROELL automated ‘Universal Testing Machine’ (UTM) is employed, and tensile testing is performed per the ASTM D638 standards, which apply to nanoclay-epoxy and epoxy composites. Additionally, the D3039 standards, designed for fiber-reinforced composites, are followed for the same purpose. Five specimens of each set and condition are tested, and the average value is considered tabulation and discussion.
2.3.2. SEM analysis
The fractured specimens undergo analysis following tensile testing using a ZEISS SEM. The specimens are cut to selected dimensions and affixed to the microscope holder to prepare for this examination. A ‘mini sputter coater apparatus’ is utilized to apply a lean layer of conductive material on the specimen. The coating procedure takes 10 min to complete.
2.3.3. Flexural test
The ZWICK ROELL automated ‘Universal Testing Machine’ (UTM) is utilized for conducting flexural tests adhering to the ASTM D7264 standards, which apply to epoxy and nanoclay-epoxy composites. Additionally, the D790 standard, designed for fiber-reinforced composites, is followed for the same purpose. Five specimens of each set and condition are tested, and the average value is considered tabulation and discussion.
2.3.4. Water uptake
Water uptake tests are performed as per the ASTM D570-98 standards. Initially, dry samples are weighed using a digital weighing machine and dipped in tap water for 84 d at room temperature. The samples are removed from the water every week, dried using a clean cloth, and reweighed. Water uptake (%) assessment is determined by analyzing the changes in weight over specific time intervals (Shettar et al., Citation2021).
The water uptake percentage is determined by:
3. Results and discussions
3.1. Water uptake curves
As depicted in , graphs are plotted to illustrate the relationship between the percentage of water uptake and the duration of water soaking for the specimens. The water uptake behavior of all composites follows the typical pattern observed in polymers. Weekly weight measurements of the specimens are conducted to observe changes, facilitating the calculation of water uptake percentages over time. It is observed that over the 84 d, all specimens reach a saturation point. As presented in , at room temperature, toward the end of 84 d, the water uptake (%) of PE, BE, BGE and GE are 2.19%, 1.75%, 1.57% and 1.10%, respectively. Epoxy resins have a certain degree of hygroscopicity, meaning they have an affinity to absorb moisture from their surroundings, including water (Korkees, Citation2023). This absorption occurs due to polar functional groups within the epoxy resin molecules, such as hydroxyl (-OH) and ether (-O-) groups, which can form hydrogen bonds with water molecules (Qiu et al., Citation2023). Water absorption can cause swelling of epoxy resin, leading to increased volume and softening of the material. This swelling phenomenon can further promote water absorption, creating more space for water molecules to enter the resin matrix (Li et al., Citation2024). Additionally, water molecules can act as plasticizers, reducing the cohesive forces within the resin and increasing its susceptibility to further water absorption (Muñoz & García-Manrique, Citation2015).
Water molecules generally diffuse into polymer composites in three different ways (Zhang et al., Citation2021). First, water molecules penetrate via microscopic spaces among polymer chains. Second, water molecules dispersed within pores and cracks at the fiber-resin interfaces. Third, water molecules diffused via the microcracks in the matrix caused by swelling. As presented in , NaOH-treated BE composite has lower water uptake (%) than PE for the following reasons: (1) NaOH treatment of bamboo fibers can remove hemicellulose and lignin from the fiber surface, exposing more hydroxyl groups (Wang et al., Citation2019). This enhances the compatibility and adhesion between the bamboo fibers and the epoxy matrix, reducing the pathways for water infiltration into the composite. (2) NaOH treatment can convert the surface of the bamboo fibers from hydrophilic to hydrophobic, making them less prone to water absorption (Mohammed et al., Citation2022). This modification reduces the tendency of water molecules to penetrate the composite material.
shows that GBE and GE composites have lesser water uptake (%) than PE and BE composites throughout the soaking period. Liu et al. (Citation2022) found that introducing glass fiber reduces the water uptake (%). Glass fibers typically have a more hydrophobic surface than bamboo fibers. When combined in a hybrid composite, the hydrophobic nature of glass fibers can reduce the overall hydrophilicity of the composite material (Wang et al., Citation2023). This can inhibit water absorption by creating a barrier that limits water penetration into the composite matrix (Kini et al., Citation2018).
As presented in , adding nanoclay reduces the water uptake (%) of all the composites. Finally, after 84 d, the water uptake (%) of EN, BEN, BGEN and GEN are 1.97%, 1.61%, 1.46% and 1.05%, respectively. Numerous researchers have documented how adding nanoclay to epoxy composites improves the behavior of water suppression (Chee et al., Citation2020; Kowshik et al., Citation2022). Introducing nanoclay into the epoxy decreases water molecules’ mean free path within the network, reducing water uptake (%). The higher aspect ratio of nanoclay creates challenging pathways for water molecules, significantly contributing to enhanced resistance against water uptake (Shettar et al., Citation2021).
3.2. Tensile strength of dry and water-soaked specimens
As shown in , the tensile strength of water-soaked PE, BE, BGE and GE composites decrease by 14.81%, 13.86%, 10% and 8.6%, respectively. As described in Section 3.1, BE, BGE and GE composites have lesser water uptake (%) than PE, which is the primary cause for the lesser reduction in tensile strength. NaOH treatment improves the bonding of bamboo fiber and matrix. Glass fibers typically exhibit better adhesion with epoxy resin. Fibers can enhance composite’s mechanical properties, such as its resistance to deformation and cracking. The improved interfacial bonding between the fiber and matrix can enhance the overall integrity of the composite material. These enhancements can reduce the propensity for fiber-matrix debonding and damage, which can occur due to water absorption; thus, the reduction in tensile strength is restricted (Kowshik et al., Citation2021).
As shown in , the addition of nanoclay enhances the tensile strength of epoxy and all composites. EN, BEN, BGEN and GEN composites’ tensile strength improves by 5–7% compared to PE, BE, BGE and GE composites by adding nanoclay. Numerous researchers have documented that nanoclay addition can improve the tensile strength of aforesaid composites through several mechanisms: (1) nanoclay particles have high aspect ratios and large surface areas, which enable them to form a strong network within the epoxy matrix, which enhances the load-bearing capacity of the composite (Guo et al., Citation2018). (2) Nanoclay improves interfacial adhesion, which reduces the interface debonding and improves the transfer of stress between the matrix and the reinforcement (Bin Rashid et al., Citation2024), resulting in higher tensile strength. (3) The presence of nanoclay particles can impede crack propagation. Cracks are deflected, absorbed or bridged by the nanoclay particles, preventing further propagation and thereby increasing the overall tensile strength (Kiran Zhade et al., Citation2022; Kini et al., Citation2023).
The tensile strength of water-soaked EN, BEN, BGEN and GEN composites decreases by 12%, 11%, 9% and 7.4%, respectively, which are lesser compared to PE, BE, BGE and GE composites. Adding nanoclay to aforesaid composites can mitigate the detrimental effects of water soaking on tensile strength by acting as a barrier to water diffusion, improving interfacial adhesion, imparting hydrophobicity and reducing matrix swelling (Merah et al., Citation2022; Merah & Mohamed, Citation2019). Numerous researchers have documented that adding nanoclay to polymer composites declines the effect of water soaking on the tensile strength of composites (Chee et al., Citation2020; Kowshik et al., Citation2022; Kini et al., Citation2022).
3.3. SEM analysis of dry and water-soaked specimens
displays SEM images of all dry and water-soaked specimens. The SEM images of dry and water-soaked specimens show significant variations. shows the SEM images of dry and water-soaked specimens of PE. shows PE's relatively smooth fracture surface morphology compared to a water-soaked PE specimen (). Water-soaked PE specimens reveal signs of phase separation, degradation (shear leaps and crazing) or leaching of components due to water ingress.
From , it can be observed that under tensile load, specimens have failed owing to fiber pull-out, rupture of matrix and fiber breaking. The fibers are surrounded by a substantial amount of matrix residues, indicating a strong bond between the fibers and the matrix. The interfacial bond between the fibers and epoxy is so strong that cracks occur around the pulled fibers, as shown in .
Specimen failure under water-soaked conditions () arises from matrix and fiber-matrix interface degradation, including cracking or rupturing matrix. show no traces of matrix materials on the fibers. This demonstrated how water-soaking could degrade the fiber-matrix interface and explained why the tensile strength of the composites soaked in water is lower than that of the dry composites.
shows the SEM images of all dry and water-soaked specimens with nanoclay. The fracture surfaces of PE () and EN () specimens show significant differences. EN specimens display a more complex morphology, with nanoclay imparting a river surface-like pattern. Nanoclay acts as adequate reinforcement, improving tensile strength by enhancing load transfer mechanisms and restricting the propagation of cracks within the epoxy matrix. In contrast to the dry EN specimen (), the water-soaked EN specimen () displays a less rough surface with shear leaps and crazing. When compared to the water-soaked EN specimen (), the water-soaked PE specimen () has more impact of water-soaking conditions. Adding nanoclay creates barriers for water molecules to infiltrate the epoxy resin, significantly enhancing resistance against water uptake (Al Imran et al., Citation2021; Alamri & Low, Citation2012). This may be the reason for the lesser percentage of decrease in tensile strength of EN specimens (11.9%) compared to PE specimens (14.8%).
shows that adding nanoclay has enhanced the interfacial bonding between fibers and matrix, thus improving the tensile strength of composites. Compared to SEM images of BE, BGE and GE (), the SEM images of BEN, BGEN and GEN () display a larger cluster of matrix on the fibers, indicating enhanced bonding between fiber-matrix. This might be the reason for the improved tensile strength of BEN, BGEN and GEN composites.
The water-soaking condition has adverse effects on the composites. Compared to dry specimens (), water-soaked specimens () have lesser clusters of matrix on the fibers, indicating matrix and fiber-matrix interface degradation. But, the water-soaked specimens of BEN, BGEN and GEN composites () exhibit more clusters of matrix on the fibers compared to BE, BGE and GE () composites, indicating the addition of nanoclay has diminished the effect of water-soaking effect. This may be the reason for the lesser percentage of reduction in tensile strength of BEN, BGEN and GEN composites compared to BE, BGE and GE composites.
3.4. Flexural strength of dry and water-soaked specimens
shows the flexural strength of dry specimens compared with water-soaked specimens. Compared to PE, the BE, BGE and GE composites exhibit higher flexural strength. The fibers help to distribute applied loads evenly throughout the composite material, reducing the risk of deformation and failure (Periyasamy et al., Citation2023). This reinforcement of bamboo and glass fibers enhances the ability of the epoxy to withstand bending forces, which increases the composites’ flexural strength (Hasan et al., Citation2023; Rajak et al., Citation2021).
shows that the composites’ flexural strength decreases under water-soaking condition compared to dry condition. The flexural strength of the water-soaked PE specimen is 95 MPa, which is 13.6% less when compared to PE dry specimens (110 MPa). The presence of water in the PE breaks down the chemical bonds and reduces the material’s overall strength (Luo et al., Citation2022).
Like tensile strength, the flexural strength of water-soaked BE, BGE and GE composites decrease by 12.9%, 9.3% and 8%, respectively. Also, the presence of water can lead to degradation at the interface of fibers and the matrix material, causing diminished adhesion and bonding between the fibers and matrix, ultimately resulting in a decline in flexural strength (Manaia & Manaia, Citation2021).
As shown in , the flexural strength of the nanoclay-filled composites exhibits better properties when compared to composites without nanoclay. There is a 9.5% improvement in the dry EN composites compared to dry PE, which indicates that the addition of the nanoclay helps enhance flexural strength. Merah and Mohamed (Citation2019) noticed a similar trend of results in their work.
Similar to tensile strength, the flexural strength of the BEN, BGEN and GEN composites improves by adding nanoclay compared to BE, BGE and GE composites. Nanoclay particles can improve the interfacial interactions between the polymer matrix and the reinforcing fibers. This can lead to better load transfer between the matrix and fibers, resulting in a composite material that can withstand higher bending forces without failure (Ramesh et al., Citation2022). A similar trend of results is noticed by Chee et al. (Citation2021), Ahmad et al. (Citation2023) and Kowshik et al. (Citation2022) in their work.
The flexural strength of water-soaked EN, BEN, BGEN and GEN composites decreases by 11%, 10%, 8% and 7%, respectively, which are lesser compared to PE, BE, BGE and GE composites. Nanoclay reduces the moisture absorption of the composite. Lower moisture absorption can help prevent the polymer matrix’s degradation and the composite’s weakening over time.
4. Conclusions
Tensile and flexural strengths are increased, and water uptake (%) is decreased when bamboo and glass fibers are reinforced with epoxy resin.
The tensile and flexural strengths of the dry and water-soaked specimens are compared. Water-soaking condition negatively affects all the composites. Water-soaked specimens exhibit lesser tensile and flexural strengths compared to dry specimens.
The fiber-reinforced composites display a lesser percentage of decrease in tensile and flexural strengths compared to pure epoxy.
Adding nanoclay further improves the tensile and flexural strengths of epoxy and all composites. Water uptake (%) and the effect of water-soaking on tensile and flexural strengths decline when nanoclay is added.
The water-soaking condition affects the properties of pure epoxy more and glass fiber-epoxy-nanoclay composites less.
Using SEM analysis of the fracture surface, the reasons for the specimen’s failure under tensile load are examined. The fracture surfaces of dry and water-soaked specimens show significant variations.
This work can be extended for different wt.% combinations constituent. Also, water-soaking of composites may be carried out at a controlled or elevated temperature.
Author contributions
Syed Mansoor Ahmad: conceptualization, data curation, formal analysis, investigation, methodology, visualization, writing – original draft preparation, and editing. Gowrishankar M C: conceptualization, review, editing, and supervision. Manjunath Shettar: conceptualization, methodology, visualization, writing – original draft preparation, review, and editing. All authors read and approved the final manuscript.
Disclosure statement
The authors declare no conflicts of interest.
Data availability statement
The data supporting this study’s findings are available from the corresponding author upon reasonable request.
Additional information
Notes on contributors
Syed Mansoor Ahmad
Syed Mansoor Ahmad is a Research Scholar in the Department of Mechanical and Industrial Engineering, at Manipal Institute of Technology, Manipal Academy of Higher, Manipal, India. He is pursuing his doctoral degree in the area of hybrid polymer composites. He has published his research papers in reputed international journals and conferences. His research interests include polymers and polymer composites.
Gowrishankar M. C.
Gowrishankar M. C. is a Professor in the Department of Mechanical and Industrial Engineering, at Manipal Institute of Technology, Manipal Academy of Higher Education, Manipal, India. His research interests include Machining of Materials, Heat Treatment of Ferrous and Non-Ferrous Materials. He has published more than 100 papers in reputed journals and conference proceedings.
Manjunath Shettar
Manjunath Shettar is an Associate Professor in the Department of Mechanical and Industrial Engineering, at Manipal Institute of Technology, Manipal Academy of Higher Education, Manipal, India. His research interests include Polymer Nanocomposites, Hybrid Composites, and Aging Behaviour of Polymer Nanocomposite. He has published more than 75 papers in reputed journals and conference proceedings.
References
- Abd El-Baky, M., Attia, M., Abdelhaleem, M., & Hassan, M. (2020). Mechanical characterization of hybrid composites based on flax, basalt and glass fibers. Journal of Composite Materials, 54(27), 4185–4205. https://doi.org/10.1177/0021998320928509
- Agopian, J. C., Téraube, O., Hajjar-Garreau, S., Charlet, K., & Dubois, M. (2023). Study of carbon-flax hybrid composites modified by fibre fluorination. Journal of Fluorine Chemistry, 272, 110213. https://doi.org/10.1016/j.jfluchem.2023.110213
- Ahmad, S. M., M C, G., Shettar, M., & Sharma, S. (2023). Experimental investigation of mechanical properties and morphology of bamboo-glass fiber- nanoclay reinforced epoxy hybrid composites properties and morphology of bamboo-glass. Cogent Engineering, 10(2), 2279209. https://doi.org/10.1080/23311916.2023.2279209
- Al Imran, K., Hossain, M. K., Hosur, M., & Jeelani, S. (2021). Assessment of moisture barrier, mechanical, and thermal property of base/nanophased carbon-epoxy composites in seawater. Journal of Composite Materials, 55(5), 703–715. https://doi.org/10.1177/0021998320953480
- Alamri, H., & Low, I. M. (2012). Effect of water absorption on the mechanical properties of nano-filler reinforced epoxy nanocomposites. Materials and Design, 42, 214–222. https://doi.org/10.1016/j.matdes.2012.05.060
- Al-Jumaili, S. K., Alkaron, W. A., & Atshan, M. Y. (2023). Mechanical, thermal, and morphological properties of low-density polyethylene nanocomposites reinforced with montmorillonite: Fabrication and characterizations. Cogent Engineering, 10(1), 2204550. https://doi.org/10.1080/23311916.2023.2204550
- Arya, S., Kumar, R., & Chauhan, S. (2024). Preparation and characterization of woven jute fabric layered composite by using bamboo fiber reinforced polymers as resin matrix. Construction and Building Materials, 411, 134343. https://doi.org/10.1016/j.conbuildmat.2023.134343
- Avci, A., Eker, A. A., Bodur, M. S., & Candan, Z. (2023). Water absorption characterization of boron compounds-reinforced PLA/flax fiber sustainable composite. International Journal of Biological Macromolecules, 233, 123546. https://doi.org/10.1016/j.ijbiomac.2023.123546
- Batu, T., & Lemu, H. G. (2020). Investigation of mechanical properties of false banana/glass fiber reinforced hybrid composite materials. Results in Materials, 8, 100152. https://doi.org/10.1016/j.rinma.2020.100152
- Bin Rashid, A., Haque, M., Islam, S. M. M., & Uddin Labib, K. M. R. (2024). Nanotechnology-enhanced fiber-reinforced polymer composites: Recent advancements on processing techniques and applications. Heliyon, 10(2), e24692. https://doi.org/10.1016/j.heliyon.2024.e24692
- Bulut, M., Alsaadi, M., & Erkliğ, A. (2020). The effects of nanosilica and nanoclay particles inclusions on mode II delamination, thermal and water absorption of intraply woven carbon/aramid hybrid composites. International Polymer Processing, 35(4), 367–375. https://doi.org/10.3139/217.3940
- Chee, S. S., Jawaid, M., Alothman, O. Y., & Fouad, H. (2021). Effects of nanoclay on mechanical and dynamic mechanical properties of bamboo/Kenaf reinforced epoxy hybrid composites. Polymers, 13(3), 395. https://doi.org/10.3390/polym13030395
- Chee, S. S., Jawaid, M., Sultan, M. T. H., Alothman, O. Y., & Abdullah, L. C. May (2020). Effects of nanoclay on physical and dimensional stability of Bamboo/Kenaf/nanoclay reinforced epoxy hybrid nanocomposites. Journal of Materials Research and Technology, 9(3), 5871–5880. https://doi.org/10.1016/j.jmrt.2020.03.114
- Feng Ng, L. (2022). Introduction to epoxy/synthetic/natural fibre composites. Handbook of epoxy/fiber composites (pp. 869–901). Springer Nature Singapore. https://doi.org/10.1007/978-981-19-3603-6_33
- Gholampour, A., & Ozbakkaloglu, T. (2020). A review of natural fiber composites: Properties, modification and processing techniques, characterization, applications. Journal of Materials Science, 55(3), 829–892. https://doi.org/10.1007/s10853-019-03990-y
- Guo, F., Aryana, S., Han, Y., & Jiao, Y. (2018). A review of the synthesis and applications of polymer–nanoclay composites. Applied Sciences, 8(9), 1696. https://doi.org/10.3390/app8091696
- Hasan, K. M. F., Hasan, K. M. N. A., Ahmed, T., György, S. T., Pervez, M. N., Bejó, L., Sándor, B., & Alpár, T. (2023). Sustainable bamboo fiber reinforced polymeric composites for structural applications: A mini review of recent advances and future prospects. Case Studies in Chemical and Environmental Engineering, 8, 100362. https://doi.org/10.1016/j.cscee.2023.100362
- He, F., Biolzi, L., & Carvelli, V. (2022). Effect of fiber hybridization on mechanical properties of concrete. Materials and Structures, 55(7), 195. https://doi.org/10.1617/s11527-022-02020-9
- Herlina Sari, N., Wardana, I. N. G., Surya Irawan, Y., & Siswanto, E. (2017). The effect of sodium hydroxide on chemical and mechanical properties of corn husk fiber. Oriental Journal of Chemistry, 33(6), 3037–3042. https://doi.org/10.13005/ojc/330642
- Jawaid, M., Chee, S. S., Asim, M., Saba, N., & Kalia, S. (2022). Sustainable kenaf/bamboo fibers/clay hybrid nanocomposites: Properties, environmental aspects and applications. Journal of Cleaner Production, 330, 129938. https://doi.org/10.1016/j.jclepro.2021.129938
- Kaima, J., Preechawuttipong, I., Peyroux, R., Jongchansitto, P., & Kaima, T. (2023). Experimental investigation of alkaline treatment processes (NaOH, KOH and ash) on tensile strength of the bamboo fiber bundle. Results in Engineering, 18, 101186. https://doi.org/10.1016/j.rineng.2023.101186
- Kenned, J. J., Sankaranarayanasamy, K., & Kumar, C. S. (2021). Chemical, biological, and nanoclay treatments for natural plant fiber-reinforced polymer composites: A review. Polymers and Polymer Composites. 29(7), 1011–1038. https://doi.org/10.1177/0967391120942419
- Kini, A. U., Shettar, M., Gowrishankar, M. C., & Sharma, S. (2023). A technical review on epoxy-nanoclay nanocomposites: Mechanical, hygrothermal and wear properties. Cogent Engineering, 10(2), 2257949. https://doi.org/10.1080/23311916.2023.2257949
- Kini, A., Shettar, M., Kowshik, S., R, N., & Chate, G. (2022). Water soaking and re-drying effect on mechanical and wear properties of nanoclay-polyester nanocomposites. Materials Research, 25, e20210478. https://doi.org/10.1590/1980-5373-mr-2021-0478
- Kini, U. A., Shettar, M., Sharma, S., Hiremath, P., & Gowrishankar, M. C. (2018). Investigation on effect of cold soaking on the properties of nanoclay-GFRP composite. Materials Research Express, 6(1), 015206. https://doi.org/10.1088/2053-1591/aae967
- Kiran Zhade, S., Kumar Chokka, S., Suresh Babu, V., & Sai Srinadh, K. V. (2022). A review on mechanical properties of epoxy-glass composites reinforced with nanoclay. Epoxy-based composites. IntechOpen. https://doi.org/10.5772/intechopen.102159
- Korkees, F. (2023). Moisture absorption behavior and diffusion characteristics of continuous carbon fiber reinforced epoxy composites: A review. Polymer-Plastics Technology and Materials, 62(14), 1789–1822. https://doi.org/10.1080/25740881.2023.2234461
- Kowshik, S., M C, G., Shettar, M., Bhat, R., & B M, G. (2021). Durability prediction analysis on mechanical properties of GFRP upon immersion in water at ambient temperature. Cogent Engineering, 8(1), 1956869. https://doi.org/10.1080/23311916.2021.1956869
- Kowshik, S., Shettar, M., Rangaswamy, N., Chate, G., & Somdee, P. (2022). Effect of nanoclay on mechanical, flammability, and water absorption properties of glass fiber-epoxy composite. Cogent Engineering, 9(1), 2069070. https://doi.org/10.1080/23311916.2022.2069070
- Li, C., Feng, C., Zhang, L., Shan, J., & Shi, M. (2024). A review of the effect of hydrothermal aging on the mechanical properties of < scp > 2D</scp > fiber‐reinforced resin matrix composites. Polymers for Advanced Technologies, 35(1), 1–28. https://doi.org/10.1002/pat.6239
- Liu, Z., Wang, H., Yang, L., & Du, J. (2022). Research on mechanical properties and durability of flax/glass fiber bio-hybrid FRP composites laminates. Composite Structures, 290, 115566. https://doi.org/10.1016/j.compstruct.2022.115566
- Luo, Q., Li, Y., Zhang, Z., Peng, X., & Geng, G. (2022). Influence of substrate moisture on the interfacial bonding between calcium silicate hydrate and epoxy. Construction and Building Materials, 320, 126252. https://doi.org/10.1016/j.conbuildmat.2021.126252
- Manaia, J. P., & Manaia, A. (2021). Interface modification, water absorption behaviour and mechanical properties of injection moulded short hemp fiber-reinforced thermoplastic composites. Polymers, 13(10), 1638. https://doi.org/10.3390/polym13101638
- Merah, N., & Mohamed, O. (2019). Nanoclay and water uptake effects on mechanical properties of unsaturated polyester. Journal of Nanomaterials, 2019, 1–11. https://doi.org/10.1155/2019/8130419
- Merah, N., Ashraf, F., & Shaukat, M. M. (2022). Mechanical and moisture barrier properties of epoxy–nanoclay and hybrid epoxy–nanoclay glass fibre composites: A review. Polymers, 14(8), 1620. https://doi.org/10.3390/polym14081620
- Mohammed, M., Rahman, R., Mohammed, A. M., Adam, T., Betar, B. O., Osman, A. F., & Dahham, O. S. (2022). Surface treatment to improve water repellence and compatibility of natural fiber with polymer matrix: Recent advancement. Polymer Testing, 115, 107707. https://doi.org/10.1016/j.polymertesting.2022.107707
- Muñoz, E., & García-Manrique, J. A. (2015). Water absorption behaviour and its effect on the mechanical properties of flax fibre reinforced bioepoxy composites. International Journal of Polymeric Science, 2015, 1–10. https://doi.org/10.1155/2015/390275
- Musthaq, M. A., Dhakal, H. N., Zhang, Z., Barouni, A., & Zahari, R. (2023). The effect of various environmental conditions on the impact damage behaviour of natural-fibre-reinforced composites (NFRCs)—A critical review. Polymers, 15(5), 1229. https://doi.org/10.3390/polym15051229
- Olhan, S., Antil, B., Khatkar, V., & Behera, B. K. (2023). Mechanical, thermal, and viscoelastic behavior of sisal fibre-based structural composites for automotive applications: Experimental and FEM analysis. Composite Structures, 322, 117427. https://doi.org/10.1016/j.compstruct.2023.117427
- Periyasamy, D., Manoharan, B., Arockiasamy, F. S., Aravind, D., Senthilkumar, K., Rajini, N., Muhammed, F. F., & Al-Lohedan, H. A. (2023). Exploring the recycling potential of HDPE films reinforced with flax fiber for making sustainable decorative tiles. Journal of Materials Research and Technology, 25, 2049–2060. https://doi.org/10.1016/j.jmrt.2023.06.067
- Qiu, C., Jiang, L., Gao, Y., & Sheng, L. (2023). Effects of oxygen-containing functional groups on carbon materials in supercapacitors: A review. Materials and Design, 230, 111952. https://doi.org/10.1016/j.matdes.2023.111952
- Rajak, D. K., Wagh, P. H., & Linul, E. (2021). Manufacturing technologies of carbon/glass fiber-reinforced polymer composites and their properties: A review. Polymers, 13(21), 3721. https://doi.org/10.3390/polym13213721
- Rajeshkumar, G. (2020). An experimental study on the interdependence of mercerization, moisture absorption and mechanical properties of sustainable Phoenix. Journal of Industrial Textiles, 49(9), 1233–1251. https://doi.org/10.1177/1528083718811085
- Ramachandran, A., Mavinkere Rangappa, S., Kushvaha, V., Khan, A., Seingchin, S., & Dhakal, H. N. (2022). Modification of fibers and matrices in natural fiber reinforced polymer composites: A comprehensive review. Macromolecular Rapid Communications, 43(17), 2100862. https://doi.org/10.1002/marc.202100862
- Ramesh, M., Rajeshkumar, L. N., Srinivasan, N., Kumar, D. V., & Balaji, D. (2022). Influence of filler material on properties of fiber-reinforced polymer composites: A review. e-Polymers, 22(1), 898–916. https://doi.org/10.1515/epoly-2022-0080
- Saada, K., Zaoui, M., Amroune, S., Benyettou, R., Hechaichi, A., Jawaid, M., Hashem, M., & Uddin, I. (2024). Exploring tensile properties of bio composites reinforced date palm fibers using experimental and Modelling Approaches. Materials Chemistry and Physics, 314, 128810. https://doi.org/10.1016/j.matchemphys.2023.128810
- Shettar, M., Doshi, M., & Rawat, A. K. (2021). Study on mechanical properties and water uptake of polyester-nanoclay nanocomposite and analysis of wear property using RSM. Journal of Materials Research and Technology, 14, 1618–1629. https://doi.org/10.1016/j.jmrt.2021.07.034
- Shettar, M., Shettigar, P., Manjunath, M., & Rao, U. S. (2020). Study on effect of water soaking conditions on properties and morphology of glass fiber–cement–polyester composites. Journal of Materials Research and Technology, 9(4), 8697–8704. https://doi.org/10.1016/j.jmrt.2020.05.117
- Silva, G., Kim, S., Aguilar, R., & Nakamatsu, J. (2020). Natural fibers as reinforcement additives for geopolymers – A review of potential eco-friendly applications to the construction industry. Sustainable Materials and Technologies, 23, e00132. https://doi.org/10.1016/j.susmat.2019.e00132
- Singh, T. (2021). Optimum design based on fabricated natural fiber reinforced automotive brake friction composites using hybrid CRITIC-MEW approach. Journal of Materials Research and Technology, 14, 81–92. https://doi.org/10.1016/j.jmrt.2021.06.051
- Song, H., Liu, T., & Gauvin, F. (2024). Enhancing mechanical performance of green fiber cement composites: Role of eco-friendly alkyl ketene dimer on surfaces of hemp fibers. Journal of Materials Research and Technology, 28, 3121–3132. https://doi.org/10.1016/j.jmrt.2023.12.255
- Suresh, S., Shettar, M., Gowrishankar, M. C., & Sharma, S. (2023). Durability analysis on properties of water soaked PNNCs and CS-ANN model for wear property analysis of PNNCs. Cogent Engineering, 10(1), 2213977. https://doi.org/10.1080/23311916.2023.2213977
- Verma, S. K., Gupta, A., Patel, V. K., Gangil, B., & Ranikoti, L. (2019). The potential of natural fibers for automotive sector (pp. 31–49). Automotive Tribology. Energy, Environment, and Sustainability. Springer, Singapore. https://doi.org/10.1007/978-981-15-0434-1_3
- Vigneshwaran, S., Sundarakannan, R., John, K. M., Joel Johnson, R. D., Prasath, K. A., Ajith, S., Arumugaprabu, V., & Uthayakumar, M. (2020). Recent advancement in the natural fiber polymer composites: A comprehensive review. Journal of Cleaner Production, 277, 124109. https://doi.org/10.1016/j.jclepro.2020.124109
- Wang, D., Bai, T., Cheng, W., Xu, C., Wang, G., Cheng, H., & Han, G. (2019). Surface modification of bamboo fibers to enhance the interfacial adhesion of epoxy resin-based composites prepared by resin transfer molding. Polymers, 11(12), 2107. https://doi.org/10.3390/polym11122107
- Wang, Q., Chen, T., Wang, X., Zheng, Y., Zheng, J., Song, G., & Liu, S. (2023). Recent progress on moisture absorption aging of plant fiber reinforced polymer composites. Polymers, 15(20), 4121. https://doi.org/10.3390/polym15204121
- Zhang, K., Liang, W., Wang, F., & Wang, Z. (2021). Effect of water absorption on the mechanical properties of bamboo/glass-reinforced polybenzoxazine hybrid composite. Polymers and Polymer Composites. 29(1), 3–14. https://doi.org/10.1177/0967391120903664