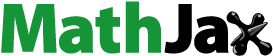
Abstract
Green oils are plant extract oils such as Karanja, Pinnay, Coconut oil, which are non-corrosive, non-toxic and environmentally friendly, and are capable of replacing petrol oil quenchants. In this study, Pinnay oil, Karanja seed oil and their blends (equal volume in proportion) were used as quenchants. Before quenching for hardening, austenitising was performed at three different temperatures ranging from 890 °C to 950 °C. The effects of austenitising temperature and vegetable oil quenchants on the hardness, room-temperature impact toughness and microstructure of normalised 42CrMo4 steel were determined. Prior to the hardening treatment, the as-received 42CrMo4 steel was subjected to normalising treatment, which enhanced the hardness and toughness properties due to grain refinement of the as-received steel. As the oil viscosity decreases, the hardness increases with marginal alteration in the impact resistance. In all the quenching condition, with an increase in austenitising temperature up to 920 °C, there was an increase in hardness around 7% and decrease in toughness around 37%, whereas at 950 °C there was a decrease in hardness around 24% and an increase in the toughness of the steel around 47% as compared to that of 920 °C. Also, blend oil quenched specimens showed balanced hardness and impact toughness compared to Pinnay oil and Karanja oil quenched specimens.
1. Introduction
Austenitisation process is crucial because the room-temperature microstructure prior to hardening has a substantial effect on the final properties, it is important to select an appropriate austenitisation temperature. Critical controlling factors for the austenitisation process include heating temperature and soaking time. For a well-defined martensite microstructure, which is typically assisted by favourable mechanical properties, these parameters must be carefully selected (Samadian et al., Citation2014; Kurt et al., Citation2023). The temperatures used for austenitising control the austenite grain size, which in turn affects the shape and size of the martensite needles (Zhao et al., Citation2013).
Normalising is the process which ensures homogenisation of the grains and fine grain structure after cooling. Normalising is a process that increases a part’s strength, toughness and hardness. Heating the component above its upper critical temperature, maintaining it there for a long enough period of time till austenitising completes, and allowing it to cool to room temperature in still air. It achieves grain size uniformity throughout the structure. The microstructure becomes fine pearlite as a result of this moderate cooling rate (Tecer, Citation2020).
Because of its high strength and hardness, 42CrMo4 (AISI 4140) steel is commonly utilised in a range of applications, including automobile drive components, bolted assemblies and forged parts. When an ideal strength and toughness balance is required, steel is usually employed immediately after heat treatment. Heat treatments, such as quenching and tempering, are used to reinforce materials like steel and other iron alloys. If the process variables are carefully selected, hardening alone can be utilised as the final heat treatment. Tempering is frequently used to reduce brittleness, increase ductility and toughness, and relieve residual stresses caused after quenching (Çalık et al., Citation2020; Zafra et al., Citation2018; Zhang et al., Citation2023). Heating and quenching can drastically alter the mechanical properties of steel, such as percentage elongation, hardness and strength. Steel heat treatment has little impact on other qualities such as its capacity to transfer heat and electricity. There are several methods for heating steel and processing it in a medium to extract heat from a specimen. Owing to the reasonable level of chromium and carbon in medium-carbon steel, hardening followed by tempering is regarded as the most typical heat treatment process (Rooprai et al., Citation2022; Ismail et al., Citation2016; Chuaiphan et al., Citation2013).
The majority of steel products, including precise gauges, bearings, gears, tools and dies, are heat treated before use. The parts are subjected to heat treatment to enhance their quality consistency and specific characteristics, including corrosion resistance, toughness and hardness. The alloy type, quantity present and planned service conditions influence the heat treatment applied. The common heat treatments used to alter the mechanical properties and microstructure of steels are annealing, normalising, hardening and tempering (Agboola et al., Citation2015; Salvetr et al., Citation2023).
Quench hardening is a prominent and essential heat treatment method for enhancing steel performance. Cracks and residual stress concentrations in the material are also the primary reasons for the rejected components, leading to heavy production losses. These problems can be successfully mimicked if the hardening (quenching) parameters are properly designed and controlled. The essentials of a successful hardening process are achieving acceptable mechanical characteristics and limiting the probability of surface and subsurface quenching cracks. The requirement of hardness penetration and distribution (hardenability) across the specimen, temperature of quenching and the severity of the quenching medium for the quenching process influence the formation of a hardened phase, that is, a martensitic structure without warping or core-directed cracks (Fernandes & Prabhu, Citation2008; NKOCET, 2016).
Water, brine, oil and synthetic solutions are typical quenchants that are generally used depending on the application needs. Water has the weakness of developing cracks after quenching by distortion or causing dimensional variations as a result of retained austenite or metastable martensite formed by a rapid rate of cooling, whereas oil does not induce sufficient hardness owing to a slower cooling rate without much dimensional instability. A polymer quenchant can offer a cooling rate between water and oil; however, it is difficult to concentrate. This necessitates the development of a suitable quenching medium that is both cost-effective and capable of delivering a significant hardness. As a result, eco-friendly oils are slowly substituting standard conventional oils because they are non-toxic, environmentally beneficial and less expensive (Ramesh & Narayan Prabhu, Citation2014; Prathviraj et al., Citation2020).
Engine oils and additives have many disadvantages, such as toxicity, non-biodegradability and steadily rising costs, as a result of worldwide scarcity. Bio-based oils are ecologically friendly, renewable and biodegradable, in contrast to conventional mineral oils (Owuna, Citation2020). Vegetable oil quenchants, sometimes referred to as green oils, are derived from plant sources, such as seeds, leaves, flowers and fruits, by hot, cold or solvent extraction. A mixture of vegetable and animal oils was also used (Otero et al., Citation2012). Bio oils are defined as having low toxicity to humans and the environment, and are highly biodegradable in nature (Chandrakar & Suhane, Citation2014).
It was investigated how the mechanical and microstructure of quenched medium carbon steel were affected by the cooling effects of mineral oil blends (SAE40) and palm kernel oil used as quenching media. It was found that samples quenched in pure palm kernel oil had the highest hardness value compared to those cooled in SAE40, indicating that the quench severity of pure palm kernel oil is higher than that of SAE40. But the treated samples’ hardness in the combination of mineral and palm kernel oil was just moderate (Agboola et al., Citation2016). A comparative analysis of the mechanical properties of low-carbon steel was carried out with a variety of quenching media, including water, oil-in-water emulsion, brine, cottonseed oil and hybrid polymer quenchant. The samples quenched in brine solution were found to have the best mechanical properties, although they could be more vulnerable to corrosion attack (Rana Bhavesh et al., Citation2020). In a different investigation, AISI 4140 steel was mechanically evaluated using a variety of quenchants, including ice water (ice cubes with salt), water, coconut oil, castor oil and salt water. Compared to other quenched specimens, it was shown that AISI 4140 (42CrMo4 steel) specimens quenched in castor oil showed good wear resistance, ductility, and strength (Bhagyalaxmi et al., Citation2019).
For many years, engineers have worked tirelessly to strengthen and toughen alloy steels, with a particular focus on the influence of material microstructures on mechanical characteristics. Design engineers usually focus on preventing early failures by applying stresses lower than a high-ductility material’s yield strength. For this reason, design requirements gave priority to a material’s toughness (Sakkaki et al., Citation2020).
The aim of this work is to achieve a balanced hardness and room-temperature impact resistance property of 42CrMo4 steel while hardening in a green oil medium. The uniqueness of this work lies in the usage of a green oil blend quenchant for better property distribution. Also to find a desirable austenitising temperature for 42CrMo4 steel.
2. Experimental details
2.1. 42CrMo4 steel’s chemical composition
Spectroscopic methods were used to find out the chemical composition of the purchased 42CrMo4 steel. Chemical composition (wt. %) is within the standard composition of 42CrMo4 steel (). shows the 42CrMo4 steel’s chemical composition in the as-received state; for comparison purposes, the standard composition is also shown.
Table 1. 42CrMo4 steel’s chemical composition.
2.2. Charpy impact test samples
The samples were machined according to ASTM E-23 for the Charpy impact tests. Using Wire EDM, CNC lathe and a CNC mill, 10 mm side square specimens of 55 mm length with a ‘V’ notch at the middle of the top square face were created.
2.3. Hardness and microstructure samples
Testing and examining the hardness and microstructure of samples is a crucial step in many industries. The specimens were prepared using wire EDM and tested according to the ASTM E18-22 standard. The same samples were utilised for the microstructure study as well.
Using emery sheets ranging in grade from 400 to 1000 in increments of 200 then 1500 and 2000, the specimens for the microstructure analysis were polished. After that, they were polished using alumina paste on a polishing wheel until the surface was mirror-polished. For SEM examination, Nital was used to etch the specimens.
2.4. Heat treatment
42CrMo4 steel rods were purchased and cut according to the standards and subjected to normalising heat treatment. To normalise the room-temperature grains, the samples were heated to 920 °C, soaked for 2 h at a constant temperature, and then cooled in air. Later, these normalised specimens were hardened by reheating at 890 °C, 920 and 950 °C for 2 h each, and then quenched with Pinnay seed oil, Karanja seed oil and a combination of (blend) these two oils (50 vol% of each). Oil viscosity was determined using a Saybolt viscometer (). An electric furnace with a heat capacity of up to 1000 °C was used.
Table 2. Quenchant’s oil viscosity.
2.5. Hardness and impact toughness test
The specimen’s hardness was assessed using a Rockwell hardness tester (Make: FIE, INDIA, Model: RASN) equipped with a diamond cone indenter and a load range of 150 kgf. In order to assess the specimens’ impact toughness at room temperature, the Charpy impact test (FIE, INDIA, Model: IT-30(STD)) was conducted.
2.6. Microstructure study
The polished and etched samples were analysed using a scanning electron microscope. A Scanning electron microscope (SEM Make: ZEISS, USA, Model: EVO MA 18) was equipped with a specialised detector that allowed for high-resolution imaging of the sample surface. This enables researchers to observe and analyse microstructural features such as grain boundaries and phase distributions, which are important for understanding material properties and performance. Additionally, the use of nital etching prior to imaging enhances the contrast and visibility of these microstructural features, providing valuable insights into the composition and characteristics of the specimen.
3. Results and discussions
3.1. Study of microstructure
The specimen as received () has irregularly shaped grains in its microstructure. This kind of morphology is an example of a standard cast structure. The high pouring temperature and uneven cooling process caused by variations in the mould wall interface (chilling action) and other casting areas are the causes of these irregular grains (Avner, Citation2017). Compared to the as-received specimen, which has coarser uneven grains, the normalised specimen () exhibits finer uniform grains.
The microstructures of quenched steel at 890 °C after normalising heat treatment in Pinnay oil, blend oil and Karanja oil are shown in , respectively. The heating temperature for austenitisation at 890 °C was 50 °C above the upper critical temperature (840 °C) of the steel (Avner, Citation2017). During heating to austenitisation, a complete transformation of the room-temperature structure (pearlite and proeutectoid ferrite) into single-phase austenite occurs. Hence, all three microstructures () exhibited a complete martensitic structure after quenching. The lower the austenitisation temperature (890 °C), the finer the austenite grains during heating, wherein the formed martensite contains finer needles with a lower l/d value, where l represents the length of the needles and ‘d’ is the width of the needles (Sharma et al., Citation2011). The black patches present between the needles(lath) retained austenite during the martensite transformation. If we analyse the three images (), the average l/d value is higher in than in . shows the least. Accordingly, the impact toughness of the blended oil quench in is higher, followed by that of Pinnay () and Karanja ().
Figure 2. Microstructure of (a) normalised and hardened Pinnay oil quench (b) normalised and hardened blend oil quench (c) normalised and hardened Karanja oil quench at austenitising temperature of 890 °C.

The microstructure of the same steel at a moderate austenitising temperature (920 °C) contains a more systematic orientation of the needles within the colony with a shorter needle length (lower l/d ratio), indicating lower toughness values. The needles in are coarser than those in , whereas those in are still finer. Accordingly, the hardness of the specimen (), that is, the specimen quenched in thin (low viscosity) Karanja oil, shows better hardness. Moreover, black patches (retained austenite) between the needles were minimal, as shown in (austenitising, moderate temperature), representing excellent hardness.
Figure 3. Microstructure of (a) normalised and hardened Pinnay oil quench (b) normalised and hardened blend oil quench (c) normalised and hardened Karanja oil quench at austenitising temperature of 920 °C.

shows the high-temperature (950 °C) austenitised quenched steel at 950 °C. Because the austenitisation temp. was very high, coarser needles were formed compared with the conditions mentioned in and . The improvement in toughness over that of the moderate austenitisation temperature (920 °C) is due to the increase in the aspect ratio (l/d) of the needles. Because there were more black patches within the needles in this condition () than in the other two conditions, lower hardness values were observed ().
Figure 4. Microstructure of (a) normalised and hardened Pinnay oil quench (b) normalised and hardened blend oil quench (c) normalised and hardened Karanja oil quench at austenitising temperature of 950 °C.

Table 3. Sample hardness and room temperature impact toughness of normalised 42CrMo4 steel at various conditions.
3.2. Hardness and room temperature impact toughness test
shows the experimental mechanical characterisation results. As-received 42CrMo4 steel is harder than normalised one (32-30, equal to 2 HRc) with slightly less impact resistance (14-10, equal to 4J). This indicates that the as-received steel is as cast one. This is due to uneven cooling from high pouring temperatures in open cold mould (Avner, Citation2017). As seen from the tabulated values, the obtained mechanical test results are in line with the microstructure analysis results, as shown in . The lower the austenitisation temperature, the finer the austenite grains, while quenching the finer martensitic needle results (Sharma et al., Citation2011). The finer the grain, the higher the hardness. Accordingly, the hardness of the specimens obtained by quenching from lower and moderate austenitising temperatures is higher than that at the highest temperature (950 °C) used here. Because hardness and toughness are inversely proportional to each other, as hardness decreases, impact toughness increases (Avner, Citation2017). Hence, the microstructure supported the results obtained from the experimental mechanical property data.
shows the graphical result of data presented in . The graph shows low and moderate-temperature processed steels attaining better hardness distribution (30% increase) whereas a lot of fluctuations in impact resistance is observed at moderate temperature as compared to that at high and low temperatures. This is due to the athermal nature of distorted martensite formation.
3.3. Statistical analysis
Impact toughness and hardness were statistically examined. An analysis of variance (ANOVA) test was performed to examine how each element affected these traits. According to the ANOVA results shown in , the austenitising temperature was the most important factor affecting the hardness variation, with a relative contribution of 90%. The proportional impact of oil viscosity on hardness was found to be 0.37%. The results were comparable for the set of values used in the study. Unnamed external sources accounted for approximately 9% of the relative contribution. With a relative contribution of 95%, the austenitising temperature is the most significant factor in the analysis of impact toughness in , whereas oil viscosity has a relative influence of about 0.37% on the variation of impact toughness for the values examined in the study’s range.
Table 4. ANOVA results for the hardness of the material.
Table 5. ANOVA results for impact toughness of the material.
The impact toughness and hardness value inputs were used to create regression equations. In the range of data evaluated in this investigation, the regression equations accurately forecast the hardness and impact toughness of the components. EquationEquations (1)(1)
(1) and Equation(2)
(2)
(2) offer regression equations for hardness and impact toughness, respectively.
(1)
(1)
R-squared = 92.96%
R-sq(adj) = 81.23%
(2)
(2)
R-squared = 92.12%
R-sq(adj) = 79.00%
According to statistical data, the autenitising temperature employed during the hardening process is the most important factor influencing the hardness and impact toughness of 42CrMo4 steel. The influence of the viscosity of the quenchant on the hardness and impact toughness of steel was negligible.
4. Conclusions
Steel 42CrMo4 was successfully quenched by subjecting it to a pre-normalising treatment. Pinnay and Karanja oils were independently used for quenching, along with a continuous blend oil mixture of equal proportions obtained by these two oils. The microstructural, experimental and statistical analyses of the entire process were critically analysed, and the following conclusions were drawn.
As the quenchant viscosity decreased, the hardness of the quenched steel increased, whereas the toughness was dependent on the aspect ratio of the needles and the gap between the two consecutive needles.
Retained austenite has a detrimental effect on the hardness and impact resistance.
In all the conditions of austenitising, the blend oil quench specimen has better balanced hardness and impact toughness properties as compared to the other two independent oil quenches.
The regression equation that was generated is the most suitable for the model that is being examined, as indicated by the high R-squared values for impact toughness and hardness that were obtained using the regression equations.
Statistical analysis shows that the austenitising temperature has complete dominance in controlling the mechanical properties, as proven by the experimental results.
Impact resistance increase is more proned to treated specimen compared to hardness increase. It is approximately 30% higher as compared to hardness increase at all austenitising temperatures under study.
Blend oil and Karanja oil where viscosity is less, the variation in the hardness and impact resistance is
at all austenitising temperatures.
Heat treated with high temperature austenitising shows approximately 30% reduction in hardness whereas approximately 30% increase in impact resistance as that of low and moderate temperature processed specimen.
Author’s contribution
Bhagyalaxmi – Experiment work, Drafting of paper
Jayashree P K – Designing the methodology
Ananda Hegde – Conception and design
Rajarama Hande – Revising the manuscript
Sathyashankara Sharma – Interpretation and Analysis
Disclosure statement
Authors declare that they do not have any conflict of interests whatsoever.
Data availability statement
Authors agree to share the data upon reasonable request.
Additional information
Notes on contributors
Bhagya Laxmi
Mrs. Bhagyalaxmi is working as Assistant Professor Senior Scale in the Department of Mechanical & Industrial Engineering, MIT, MAHE, Manipal. She holds B.E. (Industrial & Production Engg.) and M.Tech. (Manufacturing Engg. & Technology). She has more than 13 years of teaching experience. She has published 8 papers in journals and conferences. Her area of interest includes heat treatment of metals and manufacturing.
Jayashree P.K.
Dr. Jayashree P K is working as Associate Professor in the Department of Mechanical & Industrial Engineering, MIT, MAHE, Manipal. She holds B.E. (Industrial & Production Engg.), M.Tech. (Production Engineering) and Ph.D. (Materials Engineering) degrees. She has more than 20 years of teaching experience. Her area of interest includes materials engineering, heat treatment of metals. She has published more than 20 papers in journals and conferences.
Ananda Hegde
Dr. Ananda Hegde is working as Associate Professor in the Department of Mechanical & Industrial Engineering, MIT, MAHE, Manipal. He holds B.E. (Mechanical), M.Tech (Materials Engineering) and Ph.D. (Heat treatment) degrees. He has more than 10 years of teaching experience. His area of interest includes materials engineering, heat treatment of metals. He has published more than 25 papers in journals and conferences.
Rajarama Hande
Mr. Rajarama Hande is working as Additional Professor in the Department of Mechanical & Industrial Engineering, MIT, MAHE, Manipal. He holds B.E. (Mechanical), M.Tech. (Engineering Management). He has more than 35 years of teaching experience. His area of interest includes materials engineering. He has published 3 papers in journals.
Sathyashankara Sharma
Dr. Sathyashankara Sharma is working as Senior Professor and Head in the Department of Mechanical & Industrial Engineering, MIT, MAHE, Manipal. He holds B.E. (Mechanical), M.Tech. (Materials Engineering) and Ph.D. (Materials Engineering) degrees. He has more than 35 years of teaching experience. His area of interest includes materials engineering, heat treatment of metals and composites and deformation behavior of metals and composites. He has published more than 150 papers in journals and conferences.
References
- Agboola, J. B., Abubakre, O. K., Mudiare, E., & Adeyemi, M. B. (2016). Performance assessment of vegetable oil and mineral oil blends during heat treatment of medium carbon steel. International Journal of Microstructure and Materials Properties, 11(3–4), 203–213. https://doi.org/10.1504/IJMMP.2016.079141
- Agboola, J. B., Kamardeen, O. A., Mudiare, E., Adeyemi, M. B., & Afolabi, S. A. (2015). Performance assessment of selected Nigerian vegetable oils as quenching media in hardening process for medium carbon steel. Journal of Minerals and Materials Characterization and Engineering, 03(02), 85–93. https://doi.org/10.4236/jmmce.2015.32011
- Avner, S. H. (2017). Introduction to physical metallurgy (2nd ed.). Mc Graw Hill education.
- Bhagyalaxmi, Sharma, S, & Shetty, A. (2019). Quench hardening and mechanical characterization of AISI 4140 Steel. International Journal of Mechanical and Production Engineering Research and Development (IJMPERD), 9(2), 589–594. https://doi.org/10.24247/ijmperdapr201958.
- Çalık, A., Dokuzlar, O., & Uçar, N. (2020). The effect of heat treatment on mechanical properties of 42crmo4 steel. Journal of Achievements in Materials and Manufacturing Engineering, 1(98), 5–10. https://doi.org/10.5604/01.3001.0014.0811
- Chandrakar, J. K., & Suhane, A. (2014). The prospects of vegetable based oils as metal working fluids in manufacturing application –A review. International Journal of Engineering Research and Technology, 3(5), 2196–2203. https://doi.org/10.17577/IJERTV3IS051543
- Chuaiphan, W., Srijaroenpramong, L., & Pinpradub, D. (2013). The effects of heat treatment on microstructure and mechanical properties of AISI 4140 for base cutter cane harvester. Advanced Materials Research, 774–776, 1059–1067. https://doi.org/10.4028/www.scientific.net/AMR.774-776.1059
- Fernandes, P., & Prabhu, K. N. (2008). Comparative study of heat transfer and wetting behaviour of conventional and bioquenchants for industrial heat treatment. International Journal of Heat and Mass Transfer. 51(3–4), 526–538. https://doi.org/10.1016/j.ijheatmasstransfer.2007.05.018
- Ismail, N. M., Khatif, N. A. A., Kecik, M. A. K. A., & Shaharudin, M. A. H. (2016). The effect of heat treatment on the hardness and impact properties of medium carbon steel. IOP Conference Series: Materials Science and Engineering, 114(1), 012108. https://doi.org/10.1088/1757-899X/114/1/012108
- Kurt, H. I., Berk Akyuz, U. & Ergul, E. (2023). Mechanical changes and analysis of heat-treated 4140 steel with Taguchi method and ANOVA. The International Journal of Materials and Engineering Technology (TIJMET), 6(1), 1–6. http://dergipark.gov.tr/tijmet
- Otero, R. L. S., Canale, L. C. F., & Totten, G. E. (2012). Use of vegetable oils and animal oils as steel quenchants: A historical review-1850–2010. Journal of ASTM International, 9(1), 1–38. https://doi.org/10.1520/JAI103534
- Owuna, F. J. (2020). Stability of vegetable based oils used in the formulation of ecofriendly lubricants – a review. Egyptian Journal of Petroleum, 29(3), 251–256. https://doi.org/10.1016/j.ejpe.2020.09.003
- Prathviraj, M. P., Samuel, A., & Narayan Prabhu, K. (2020). Reprocessed waste sunflower cooking oil as quenchant for heat treatment. Journal of Cleaner Production, 269, 122276. https://doi.org/10.1016/j.jclepro.2020.122276
- Ramesh, G., & Narayan Prabhu, K. (2014). Effect of thermal conductivity and viscosity on cooling performance of liquid quench media. International Heat Treatment and Surface Engineering, 8(1), 24–28. https://doi.org/10.1179/1749514813Z.00000000082
- Rana Bhavesh, R., Rana Kamlesh, M., & Rao Vandana, J. (2020). Study the effect of various quenching media on hardening behavior of en 9 steel. International Journal of Engineering Applied Sciences and Technology, 04(10), 253–259. http://www.ijeast.com https://doi.org/10.33564/IJEAST.2020.v04i10.048
- Rooprai, R. S., Singh, H., Singh, T., & Singla, Y. K. (2022). Analysis of the wear properties of through hardened AISI-4140 alloy steel using Taguchi technique. Materials Today: Proceedings, 50, 661–664. https://doi.org/10.1016/j.matpr.2021.04.196
- Sharma, A., Sharma, C. P., & Rajan, T. V. (2011). Heat treatment principles and techniques. Prentice Hall of India Private Limited.
- Sakkaki, M., Sadegh Moghanlou, F., Parvizi, S., Baghbanijavid, H., Babapoor, A., & Shahedi Asl, M. (2020). Phase change materials as quenching media for heat treatment of 42CrMo4 steels. Journal of Central South University, 27(3), 752–761. https://doi.org/10.1007/s11771-020-4328-8
- Salvetr, P., Školáková, A., Kotous, J., Drahokoupil, J., Melzer, D., Jansa, Z., Donik, Č., Gokhman, A., & Nový, Z. (2023). Effect of double-step and strain-assisted tempering on properties of medium-carbon steel. Materials, 16(5), 2121. https://doi.org/10.3390/ma16052121
- Samadian, P., Parsa, M. H., & Shakeri, A. (2014). Determination of proper austenitization temperatures for hot stamping of AISI 4140 steel. Journal of Materials Engineering and Performance, 23(4), 1138–1145. https://doi.org/10.1007/s11665-014-0896-9
- Shaikh, K. A., Inamdar, S., Qureshi, H., Bidri, U., & Zaid, S. (2016). To study the behaviour of materials AISI 1050, 1090, 4140 using conventional quenching and nano quenching. International Journal of Engineering Trends and Technology, 38(2), 81–84. https://doi.org/10.14445/22315381/IJETT-V38P216
- Tecer, M. M. (2020). Effects of various heat treatment procedures on the toughness of aisi 4140 low alloy steel. The International Journal of Materials and Engineering Technology, 003, 131–149. http://dergipark.gov.tr/tijmet
- Zafra, A., Peral, L. B., Belzunce, J., & Rodríguez, C. (2018). Effect of hydrogen on the tensile properties of 42CrMo4 steel quenched and tempered at different temperatures. International Journal of Hydrogen Energy, 43(18), 9068–9082. https://doi.org/10.1016/j.ijhydene.2018.03.158
- Zhang, Y., Zhao, H., Liu, P., Wei, M., Lu, Y., & Liu, Z. (2023). Effect of tempering temperature on the microstructure and mechanical properties of 42CrMo4 steel. Journal of Physics: Conference Series, 2459(1), 012044. https://doi.org/10.1088/1742-6596/2459/1/012044
- Zhao, S., Cheng, X., Li, S., & Zhang, Y. (2013). Effect of austenitising temperature on microstructure and mechanical properties of an ultrahigh strength high alloy G33 steel. Materials Research Innovations, 17(1), 277–281. https://doi.org/10.1179/1432891713Z.000000000230