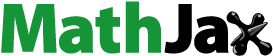
Abstract
Hybrid graphene filled glass/sisal fiber-reinforced epoxy nanocomposites were successfully synthesized with different weight percentages (0%, 0.25%, 0.5%, 0.75% and 1%) of graphene nanoplatelets. The objective of this study was to investigate the effect of weight fraction of graphene on the mechanical and physical properties of the hybrid glass/sisal fiber-reinforced epoxy nanocomposites such as tensile, flexural, hardness, density, void content, flammability, water absorption and thickness swelling. The hybrid nanocomposites were fabricated using the vacuum bag-assisted hand lay-up technique. Different layup sequences were achieved by changing the position of the glass/sisal fiber layers, maintaining a total of five layers in each composite. The obtained results suggested that the addition of 1% graphene nano filler into the sisal/glass hybrid composite enhanced the tensile strength by 49.7% and Young’s modulus by 60%. Among GNP reinforced hybrid composites, highest value of toughness (16 J) was found in case of the composite containing 1 wt.% GNP. However, when 0.5% of GNPs were added in the composite, the flexural strength and modulus reached a maximum of 306 MPa and 25 GPa, respectively, which are 228% and 362% greater than sisal/glass hybrid composites. The water absorption of the composite sample containing 0.5% GNPs was 4.62% lower than those of other hybrid glass/sisal fiber reinforced epoxy composite. Furthermore, addition of 0.5 wt% GNP resulted in a decrease in the burning rate of the hybrid composite from 11.22 mm/min to 7.6 mm/min and from 23.13 mm/min to 20.18 mm/min, respectively.
1. Introduction
In recent decades, the structural, thermal, and mechanical behavior of natural fiber reinforced composite structures have attracted much attention in various applications due to their desirable properties due to their desirable properties, which include low cost, low density, renewability, good mechanical properties and recyclability, which can aid designers in replacing hard-bound synthetic fiber-reinforced polymeric composites. However, the presence of water and biocellulose in these natural fibers makes them weaker than synthetic fibers. It has been reported in the literature that the moisture content of natural fibers can be restored by treating the fiber with an alkali solution followed by treatment in a distilled water bath, which significantly improves the elastic properties of natural fiber reinforced composites (Li et al., Citation2007). Another method of improving the interfacial bonding and adhesion properties of the natural fibers with the polymeric matrix is surface treatments and grafting techniques (Joseph et al., Citation1995; Le Thi et al., Citation1996). The use of only natural fibers with polymer matrix to make the composites is not enough to satisfy all the fundamental requirements and technical needs. Therefore, to create a superior and cost-effective composite material, it is necessary to add synthetic fiber along with natural fiber in the polymer matrix. Incorporating another fiber into a single-fiber reinforced polymer composite to take advantage of the properties of both fibers has led to the development of hybrid composites (Gupta & Deep, Citation2019). A good number of researches have been conducted on hybrid composites in which glass fibers are incorporated in single natural fiber composites to improve their mechanical properties. In the literature, it was reported that hybridization of synthetic fibers provides an enhancement in the strength of natural fiber composites composite and reduce the flammability behavior of the composite since natural fibers have a high risk of catching fire and pose a serious threat to both systems and structures (Bodduru et al., Citation2023; With et al., Citation2017). Sanadi et al. studied the effects of volume fraction of fibers on the elastic behavior of hemp fiber-reinforced laminated composites. It was observed that the elastic properties of laminate have been recorded up to 40%, followed by a decrease in the strength of the hemp fiber laminated composite with respect to increase in the fiber volume fraction (Sanadi et al., Citation1986). Jarukumjorn et al. investigated the elastic properties of glass/sisal fiber-reinforced and hybrid polymeric composite plates. It was reported that the introduction of glass fibers as an additional synthetic reinforcement on a plant fiber composite plate substantially enhanced the thermal, water absorption, and elastic properties of the laminate composite (Jarukumjorn & Suppakarn, Citation2009). Zhong et al. studied the effects of surface characteristics on the elastic and wearing behaviors of sisal fiber and sisal-aramid fiber-laminated composite plates and observed that the presence of micro fibrils on the surface of the sisal-reinforced fiber subsequently enhanced the wear, tensile, and compressive properties of the hybrid polymer laminated composites (Zhong et al., Citation2011). Alamari et al. studied the effect of the water absorption on the mechanical response of recycled cellulose fibre epoxy hybrid nanocomposites with nanoclay filled. It was noticed that water wabsorption led to a notable decrease in the flexural strength, flexural modulus, and fracture toughness (Alamri & Low, Citation2013). Venkateshwaran, N. and ElayaPerumal This study investigates the mechanical properties of woven jute/banana hybrid composite. Different layering sequences were tested using epoxy resin. Results show that the hybrid composite exhibits higher tensile and flexural strength compared to individual composites. Additionally, the impact strength of the hybrid composite surpasses other types. Fractography analysis reveals insights into the fracture behavior (Venkateshwaran & ElayaPerumal, Citation2012). Ramesh et al. performed ILSS, FTIR spectroscopy, hardness, wear behavior, and SEM analysis to investigate the physical and mechanical properties of eco-friendly hybrid composites using carbon and flax fiber reinforced epoxy composites. The authors observed that the hybridization of flax fiber with carbon fiber showed potential for enhancing certain properties (Ramesh et al., Citation2018). Gupta and Deep examines the influence of water absorption and stacking sequences on the mechanical properties of hybrid sisal/glass polyester composites. The authors observed that incorporation of glass fiber enhances the mechanical and water resistance properties, with notable improvements in tensile, flexural, and impact strengths compared to sisal composites alone. However, the authors observed that the water absorption leads to decreased mechanical performance across all composites (Gupta & Deep, Citation2019). In addition to the fiber properties, the mechanical behavior of fiber reinforced composites is influenced by matrix material, inter-laminar adhesion between the lamina, lamina orientation, and layering sequence (Fuqua et al., Citation2012). Further, in recent years, graphene nanoplalets (GNPs) filled nanocomposites have drawn much attention due to their excellent thermal, electrical and mechanical properties. Joshi et al. explored that comparatively, life cycle assessment studies on natural fiber materials as well as synthetic-glass fiber-reinforced composite plates have demonstrated the superiority of natural fiber-reinforced composite plates when considering environmental degradation compared to low-cost glass-fiber-reinforced composite plates (Joshi et al., Citation2004). Graphene nanoplatelets are 2D carbon crystals with a hexagonal nanostructure and extensive structural and elastic properties owing to the thick graphite sheet. Many researchers have reported an enhancement in the structural, mechanical and physical properties of polymer based laminated composites due to the addition of GNPs nanoplatelets. The structure and number of layers present in GNPs can affect their efficacy as reinforcement (Kamaraj et al., Citation2020). Namdev et al. studied the effects of various weight fractions of GNPs on the mechanical, thermal, and physical properties of GNPs filled hybrid carbon fiber reinforced composites and observed that 0.5 wt% GNP-filled composite showed superior strength and hardness, while the 0.25 wt% GNP-filled composite exhibited the highest impact strength (Namdev et al., Citation2022). Namdev et al. investigated the influence of various weight fractions of GNPs on the mechanical properties of carbon fiber-reinforced polymer (CFRP) composites under different environmental aging conditions. It was reported that reinforcing CFRP composites with 0.25 to 0.50 wt.% GNPs improve the tensile, flexural, interlaminar shear, impact, and torsional strength of the CFRP composites. In addition, it was revealed that the mechanical properties of CFRP composites reduces with increasing temperature and humidity conditions (Namdev, Telang, et al., Citation2023). Yadav et al. studied the effect of nano-silica addition on the mechanical and physical properties of Kevlar fiber/nano-silica/epoxy hybrid composites and noticed that the addition of nano-silica and Kevlar fiber improved the mechanical properties of the hybrid composites (Yadav et al., Citation2022). Namdev et al. investigated the effect of GNP on the wear behaviour of carbon fiber reinforced epoxy (CFRE) composites and observed that incorporation of GNP up to 0.5 wt.% significantly improved wear performance by strengthening the fiber-matrix interface and enhancing wear resistance (Namdev, Purohit, et al., Citation2023). Kumar et al. comprehensively reviews recent studies on the mechanical and thermal properties of GNPs-based polymer nanocomposites and the synergistic effects with other nanofillers. The authors noted that a small amount of GNPs can significantly improve mechanical properties (Kumar et al., Citation2019). Kumar et al. present a comparative analysis of CNTs and GNPs -reinforced polymer composites and also explores the synergistic effects of hybrid nanofillers. The authors emphasizing that GNPs has superior strength due to its wrinkled surface texture and dense surface defects (Kumar et al., Citation2021). Kumar et al. explored the effects of different GNPs functional groups (–NH2, –COOH, and –OH) alongside pristine GNPs on the mechanical performance of epoxy nanocomposites fabricated via in-situ polymerization. It was observed that the –OH functionalized GNPs/epoxy nanocomposites demonstrated the highest improvements in tensile and flexural strength compared to pure epoxy (Kumar et al., Citation2022). Moreover, Kumar et al. studied the influence of GNPs concentration and fiber stacking sequence on the tensile, interlaminar, and flexural behaviour of carbon fiber reinforced epoxy composites for various functionalized GNPs (–NH2, –COOH, and –OH) fillers (Kumar et al., Citation2023). Furthermore, studies have been conducted on the mechanical properties, water absorption, and thickness swelling of natural and synthetic composites (Jawaid et al., Citation2011; Khalil et al., Citation2011). Based on the above detailed literature review, it can be concluded that limited work has been carried out on the physical and mechanical properties of GNPs reinforced hybrid nanocomposites. Therefore, in the present study, an effort to investigate the mechanical, absorption, flammability and swelling properties of GNPs filled sisal/glass fiber reinforced polymer hybrid nanocomposites with varying GNPs weight percentage (i.e. 0, 0.25, 0.5, 0.75, 1 wt%)has been presented.
2. Experimental procedure
2.1. Raw materials
General purpose epoxy resin (LY 556) and hardener (HY 951) procured from CF composites Pvt. Ltd, India was used as a matrix. C500 grade GNPs nanoparticles (physical density of 2.2 g/cm−3; average surface area is 500 m2/g−1 and diameter is 2 μm) procured from XG Sciences, United States was used as a filler. E-glass fibers with grade 220 GSM and 0.2 mm thickness supplied by CF composites Pvt. Ltd, India and Sisal fibres derived from agave were used as the primary reinforcement. The specifications and physical properties of the raw materials are outlined in .
Table 1. Specification and Physical properties of sisal, E-glass and Epoxy matrix materials (Namdev, Purohit, et al., Citation2023; Kumar et al., Citation2019).
2.2. Samples preparation
Hybrid glass/sisal fiber-reinforced GNPs/epoxy nanocomposites were prepared using bidirectional woven sisal fibers and woven E-glass fabrics with GNPs filled epoxy using vacuum bag assisted hand layup method. First, the required weight fraction of GNPs in acetone to achieve the proper mixing and avoid agglomeration, and the prepared solution was kept at rest for some time until the acetone in the matrix material was completely evaporated. Subsequently, a mixture of GNPs and epoxy was prepared by mixing the epoxy resin with five different weight percentages (0%, 0.25%, 0.5%, 0.75% and 1%) of GNPs using ultrasonicator for 20 min with a rotation speed of 1200 rpm to obtain uniform dispersion. It is important to note that in the current study, the aforementioned weight percentages of GNPs were chosen to obtain positive results of GNP loading on the mechanical and physical properties of GNPs-filled sisal-glass fiber hybrid nanocomposites. After that, a hardener with a ratio of 10:1 was added to GNPs/epoxy mixture and then slowly mechanically stirred to minimize the formation of air bubbles within the GNPs/epoxy mixture. Then, the bidirectional woven fibers were cute in the size of 300 mm × 300 mm and laid on a wax-coated thin-film platform and the GNPs/epoxy mixture were poured on the surface of the fabrics and the fabrics were stacked one over the other up to five layers of fabric material. In this regard, the moisture present in the woven sisal fabric was cured by treating the fabric with an alkaline solution (NaOH) for approximately 24 h and the treated fabric was washed with distilled water. Finally, the hybrid nanocomposite laminate was covered with a perforated sheet and a resin absorbent foam material kept in a vacuum bag for up to 2 h. The detailed fabrication procedure is shown in .
Figure 1. A photograph image of materials and equipment’s used for the fabrication of the laminate. (a) sisal fiber, (b) glass fiber, (c) GNPs, (d) Stirrer, (e) vacuum bag, (f) plates, (g) hot air oven, (h) fabricated test specimens.
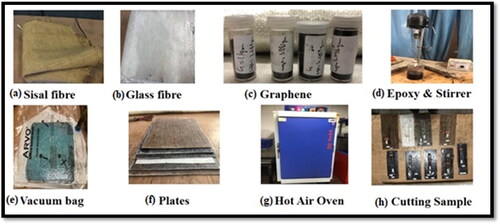
The prepared composites and their fabrication details are listed in . It is important to note that in the current study, seven composition of nanocomposites were fabricated such as pure sisal fiber reinforced composite (SFC), pure glass fiber reinforced composite (GFC), and hybridized sisal/glass fiber reinforced composite (hybrid sisal/glass fiber composites (HSGFC0), hybrid sisal/glass fiber composites with 0.25 wt% of GNPs (HSGFC0.25), hybrid sisal/glass fiber composites with 0.50 wt % of GNPs (HSGFC0.50), hybrid sisal/glass fiber composites with 0.75 wt % of GNPs (HSGFC0.75), and hybrid sisal/glass fiber composites with 1 wt % of GNPs (HSGFC1). A graphical illustration of the fabrication process is shown in .
Table 2. Presents the sequences of the fabricated laminated plates.
3. Physical and mechanical property measurements of the hybrid nanocomposites
The water absorption, flammability, swelling, tensile, bending, impact and hardness properties of hybrid glass/sisal fiber-reinforced GNPs/epoxy nanocomposites were studied using experimental tests in accordance with ASTM standards. The following subsections provide a detailed description of each experiment.
3.1. Tensile property measurement
Three rectangular specimens of each composition with dimensions of 250 × 25 × 3.5 mm3 were cut from the fully cured samples for tensile tests in accordance with ASTM D3039 standard. The tensile properties were determined using a universal testing machine (INNOTECH UTM-505B1, maximum load 30 kN) with a constant cross-head speed of 2 mm/min. For each composition the average value of the three specimens were analyzed and presented. A photographic image of the tensile test specimens before and after the tensile test is shown in . The ultimate tensile strength and tensile strain is determined using the following equation:
(1)
(1)
(2)
(2)
where
and
denote the ultimate tensile strength and strain, respectively;
and
denote the maximum force, cross-sectional area, elongation, and gauge length of the specimen, respectively.
3.2. Bending property measurement
Three rectangular specimens of each composition with dimensions of 150 × 20 × 3.5 mm3 were cut from the fully cured tensile test specimens in accordance with ASTM D790 standard. The bending characteristics were determined based on three-point bend tests, using UTM (INNOTECH, maximum load 30 kN). The UTM cross-head speed was maintained at 3 mm/min as per the aforementioned standard as shown in . The flexural strength, modulus, and strain were calculated using the following equations:
(3)
(3)
(4)
(4)
(5)
(5)
where
and
denote the flexural strength, modulus, and strain, respectively, and L, b, M, P, and d denote the length of the support span, width of the specimen, slope of the load-deflection curve, maximum load, and depth of the specimen, respectively.
3.3. Impact properties
Izod impact test was performed using a Ceast pendulum impact tester in accordance with ASTM D256. In the impact test, the sample dimensions were 55 × 10 × 3.5 mm3, and the impact test results presented were the average of the three tests. shows the experimental setup used to analyze the impact characteristics of the tested samples.
3.4. Hardness properties
Hardness of materials is often equated with durability and wear resistance. In the current study, testing was performed according to ASTM D 785 standards using a Brinell hardness tester. The sample has a dimensions of length 20 × 20 × 3.5 mm. The Rockwell test is easy to perform and more accurate than other hardness testing methods. Hardness testing involves pushing a penetrator into a material and measuring the impact. Testing the Rockwell hardness of a material involves applying a light indentation load, followed by a heavy load, and then measuring the depth of penetration. The hardness tests consist of pushing a penetrator into the material and measuring the effects. The penetration resistance of the fabricated sisal and glass fiber reinforced hybrid composite plate was tested using the Brinell hardness test according to ASTM E10. The test was carried out with a load of 150 N applied to the test sample. Indenter mark analysis can represent the resistance behavior of the laminated composite plate against penetration. The indenter was allowed to penetrate the sample for ∼5s as a dwell time. The experimental hardness measurement setup is illustrated in .
3.5. Water absorption tests
Water absorption test was performed in accordance with ASTM D570. procedure to predict the diffusion and permeability coefficient of the sisal/glass and hybrid composite. In order to study the kinetics of water absorption of the test samples, test samples were submerged in water for 55 days at room temperature as shown in . The samples were periodically removed immediately and weighed using weight balance after wiping off the water from the surface of the sample to measure content of water absorbed was measured. Before immersion in water, all samples were dried until they reached a constant weight. Sampling intervals of seven days were used to determine the weights of the immersed samples. The test was continued for two months until convergence was obtained. The percentage of water absorption is computed as presented:
(6)
(6)
where
and
represents the measured self-weight of the samples before and after immersion and the percentage of water absorption, respectively.
3.6. Thickness swelling
Natural fiber-based composites can absorb a certain amount of moisture and water when exposed to humidity. Therefore, the thickness swelling test was carried out in accordance with ASTM D570. The thickness of each sample was measured before immersion in distilled water. The thickness of the samples was measured at different time intervals and testing continued for several days until constant thickness values for the hybrid composite samples were obtained. The percentage of thickness swelling was calculated as follows:
(7)
(7)
where
denotes the swelling thickness of the sample as well as the before soaking thickness of the samples, and the after-immersion thickness is represented as
and
respectively.
3.7. Flammability test
Natural fibers are highly susceptible to fire and pose significant risks to systems and structures. Hence, the flammable behavior of the fabricated laminated composite plates must be investigated to identify the possible applications of the proposed composite plates in various sectors. In the current study, the flammability test was performed using a horizontal and vertical flammability burning test in accordance with ASTM D635 and ASTM 3801, respectively. shows the experimental horizontal and vertical flammability test setup used in the present study. In the experimental test, the cantilevered specimens shown in were continuously exposed to a split burner for approximately 30 ± 2 s and 10 ± 2 s for both flammability tests setups, respectively. If the sample showed no signs of combustion, the material is not suitable for this test method. After 30 s or when the flame reaches a height of 25 mm, the flame moved away from the sample and the timing is reset. After this, a new time, designated t1, begins and is counted until the fire reaches the 100 mm mark or fire extinguishing. The burning rate of materials was calculated using the following equation:
(8)
(8)
In EquationEquation (8)(8)
(8) , the length of the sample burnt (L) is the amount of fire spread across the specimen after a 25 mm wide line; for example, if the flame reaches the 100 mm mark, it will be 75 mm. It is important to mention that, in accordance with ASTM D635 and ASTM 3801, the flammability test results will not be reported, If the flame did not reach 25 mm, no combustion occurred after the burner was removed from the sample (or) If flame did not reach 100 mm after passes the 25 mm (or)The flame reached a 100 mm mark, but the burner speed did not exceed 40 mm/min.
3.8. Void content
Void contents for pure glass/sisalfiber reinforced composites and GNPs filled glass/sisal fiber reinforced hybrid composites were calculated according to the ASTM D2734-94. EquationEquation (9)(9)
(9) was then used to calculate the theoretical density, while the experimental measured density (
) was used to calculate the void content by using the EquationEquation (10)
(10)
(10) .
(9)
(9)
where
and
denote the weight of the glass fiber, polymer matrix, and GNPs, respectively;
and
denote the theoretical density of the composite, density of fiber, matrix, and GNP, respectively. The void volume fraction of the fabricated samples was calculated using the following equation:
(10)
(10)
where,
is denote the void content % and
denote the experimental density.
4. Results and discussion
In the current experimental study, GNPs filled sisal/glass fiber reinforced hybrid composites reinforced with glass fibers were fabricated and their properties such as tensile strength, flexural, void content, thickness swelling, and water absorption characteristics were evaluated. The SEM micrograph of the hybridized composite with 0.5 wt.% of GNPs giller reinforcement is shown in . It shows a sufficiently homogeneous dispersion of GNPs in the matrix, a condition that is inherent in our preceding discussion. The experimental results are presented in the following subsections. The sisal and glass fiber volume fraction in the matrix phase was set to 50:50 for each specimen.
Figure 9. SEM micrograph of GNPs filled sisal/glass fiber reinforced nanocomposite with filler content of 0.5 wt.%.
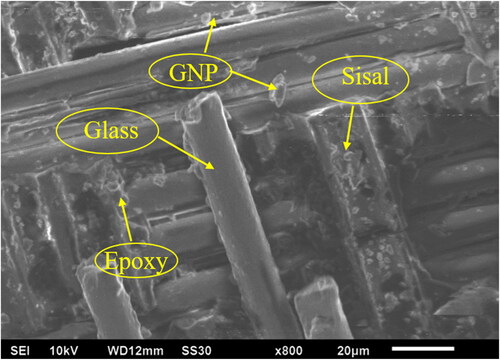
4.1. Tensile properties
The tensile properties of sisal/glass and hybrid fiber-reinforced laminated composites with various concentrations of GNPs are discussed in this section. shows the tensile load-deflection curves of the sisal/glass giber reinforced nanocomposite and hybridized sisal/glass fiber nanocomposites (HSGFC0, HSGFC0.25, HSGFC0.50, HSGFC0.75, and HSGFC1) with various weight fractions of GNPs. It can be noticed from that the ultimate failure load and maximum load carried by hybrid laminated composites with 1% GNPs filler reinforcement (HSGFC1) compared to the other GNPs reinforced hybrid composite sisal/glass reinforced. Moreover, the tensile the tensile strength and modulus of pure sisal fiber and glass fiber reinforced composites and the hybridized composites are shown in . It can also be observed form that the tensile strength of the hybrid composite did not show a regular pattern with the layering sequence variation. The highest and lowest tensile strength and composite modulus were recorded to be 91.1 MPa and 2.6 GPa and 28.5 MPa and 0.66 GPa, respectively, for GFC and SFC. The hybrid composites had strength in the range of 55.9–83.7 MPa and tensile modulus of 1.0–1.6 GPa in which their tensile strength increase by 49.7% and their modulus by 60% due to the incorporation of GNPs filler reinforcement. The highest tensile strength and tensile modulus was recorded for the hybrid composite with 1 wt% GNPs nano filler reinforcement. This could be attributed to the superior stiffness of the hybridization with GNPs reinforcement and effective load transfer between the fibre and matrix through the strong interfacial bonding. This could be attributed to the development of cross-linked sites owing to the increased surface bonding between the matrix material and GNPsnanoparticles. In addition, it was observed that GNPs affected the elongation behavior of the composites. This is due to interfacial bonding, which plays a vital role in strengthening. Further it can be observed from that the three most influential parameters on the elongation behavior of the composites were the elongation at yield, elongation at break, and elongation at peak. Furthermore, it can be seen from that the three force parameters, such as peak force, force at break, and yield force influences the force response of the tested composite specimens. It can be concluded that with increasing GNPs content, the strength properties of hybrid composites increase. It can also be concluded that the pure sisal fiber reinforced composites (SFC) exhibited the least tensile modulus values and GFC showed exhibited the highest tensile modulus. Further, it can be concluded that hybridizing the sisal fiber with the glass fiber (HSGFC0, HSGFC0.25, HSGFC0.50, HSGFC0.75, and HSGFC1) led to a slight increase in the tensile properties for various weight fractions of GNPs nano filler, which shows that one type of fiber can be compensated for by the lack of the other fiber, giving them improved properties.
Figure 10. Tensile load-elongation plot of GNPs filled sisal/glass fiber reinforced hybrid nanocomposites.
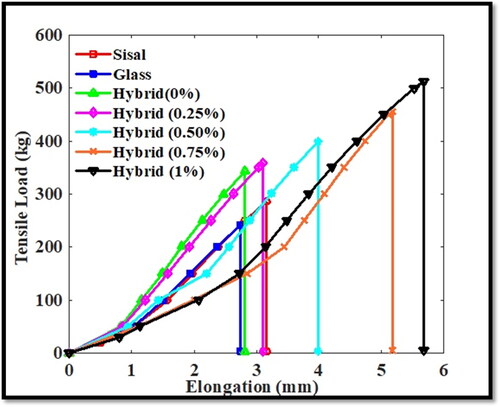
4.2. Flexural properties
To study the effect of nanofiller reinforcement on the flexural properties of the hybrid composites, the flexural test was carried out. The flexural load-deflection plot obtained is presented in . It is evident from that the highest ultimate failure load and load carried by 0.5 wt% GNPs -reinforced hybrid laminated composites compared to the other GNPs-reinforced hybrid composites. The general trend is that with increasing GNPs content in the hybrid composite, a nonlinear load behavior occurs. The flexural strength of the tested samples can be seen in . It can be observed that as GNPs content increases up to 0.5 wt%, flexural properties increase linearly, and as GNPs content increases further, flexural properties of laminated composites decrease. This may be due to the improvement in load carrying capacity of the hybrid glass/sisal fiber reinforced epoxy composites composite sample due to the incorporation of GNP nanoparticles. The bending strength increases due to the toughness and rigidity when GNPs are introduced into the nanocomposite and shows that there is a uniform dispersion of GNPs in the epoxy matrix, whereas the content of GNPs increases above 0.5 wt.% and the dispersion of GNPs may be uneven.Furthermore, to understand flexural modulus trends as well as flexural strength trends, it is important to look at the force at break and peak force of the hybrid nanocomposite specimens in relation to sample thickness. shows that the force at break and peak force of the composite specimens. It can be observed that the breaking force and peak force are higher for pure grass fiber reinforced composites compared to hybrid composites and sisal fiber reinforced composites. This is because of the thinness of the pure glass fiber reinforced composites which exhibit a better load-bearing capacity.
4.3. Hardness properties
The hardness of the GNPs filled sisal/glass fiber reinforced nanocomposite for various weight fraction of GNPs have been evaluated by using Brinell hardness tester at 150 N load and 2.5 mm indentation diameter and the values are presented in . From it can be observed that the range of hardness for GNPs filled hybrid nanocomposites varies from 19 (HRC) to 55 (HRC), and the average Rockwell hardness of this composite is calculated and the value is 70.85 (HRC). It can also be observed that the maximum hardness value was obtained for 0.75w% GNPs filled hybrid glass/sisal fiber reinforced polymer nanocomposites. This is due to the properties of interfacial adhesion between the fiber, polymer matrix, GNPs nanoparticles and ductility. The trend of increasing hardness can be attributed to the high modulus and aspect ratio of GNPs. In addition, from the hardness tests, it can be observed that the hardness values decrease when the content of GNPs is higher than 0.75 wt. % .This may be due to the increase in voids created during manufacturing.
4.4. Impact properties
An impact test was conducted to investigate how the weight percentage of GNPs nanoparticles affects the impact strength of sisal/glass fiber reinforced hybrid composites to withstand impact. Results of Izoid impact test can be seen in . It can be observed that sisal fiber by itself has high toughness (26 J), while glass fiber has low strength owing to low strain rates and less composite thickness. It was also observed that the toughness of the hybrid composites was improved by 16 J at 1 wt.% GNPs filled hybrid sisal/glass fiber reinforced composite compared to the other GNPs filled hybridized composites. It can also be noted that the breakage of the specimen begins with fracture propagation owing to the loss of adhesion between the fibers and matrix, which then initiates fiber breakage and pull-out. It can be concluded that the addition of GNPs and glass fibers into sisal fibers significantly improved the impact properties of the hybridized composites.
4.5. Horizontal and vertical flammability properties
The flammability resistance of the fabricated GNPs filled sisal/glass fiber reinforced nanocomposite sample was investigated in vertical and horizontal burning test in terms of flame time and flame travel. The test results are presented in . It important to mention that the burning rate of the material should be less than that of a material suitable for multiple applications, and the material should be resistant to ignition. shows the experiments during horizontal and vertical flammability test of the composite plates. Further, shows delaminated hybrid composites after the flammability test was not fully burned. It can be observed that the natural sisal fiber composite was completely ignited, but took more time to burn. The addition of GNPs nano filler to the hybrid composite reduced the burning rate (mm/min) of the laminated composites. Furthermore, it can be seen from that the composite’s burning rate decreases in the order of (GFC, Hybrid 0%, hybrid 0.25%, hybrid 0.75%, hybrid 1%, hybrid 0.5%, and SFC for horizontal tests and vertical flammability tests, respectively. The horizontal and vertical flammability followed the same trend. It was also noticed that the SFC fiber-reinforced composites exhibited a lower burning rate of 7.35 mm/min for horizontal flammability and burning rate of 20.0 mm/min for vertical flammability. depicts the flammability of the burning rate (mm/min) of all the composite trends and the disparities between the vertical and horizontal tests. It was also observed that the hybridized composite with 0.5 wt.% GNPs showed a decrease in burning rate from 11.22 mm/min to 7.6 mm/min and from 23.13 mm/min to 20.18 mm/min in horizontal and vertical flammability test, respectively, compared to other hybrid composites. It can be concluded that the burning rate decreased when GNPs were added to the hybrid sisal/glass fiber reinforced composites due to the promising flame-retardant properties of GNPs, which suppresses flame propagation and helps to extinguish flames. Similar observation was reported in Kamaraj et al. (Citation2020), Naveen et al. (Citation2019), Sahoo and Jena (Citation2022), and Ary Subagia et al. (Citation2014).
Figure 19. Experimental results of (a) horizontal, (b) vertical test, and (c) delamination of composites.
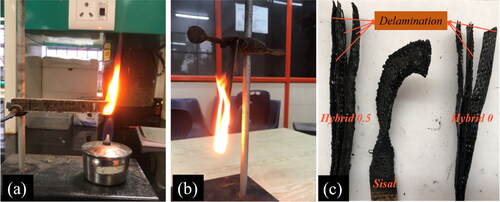
Table 3. Results of the horizontal and vertical flame tests.
4.6. Water absorption
Natural fiber reinforced composites are susceptible to moisture absorption for many reasons: (ii) fiber volume fraction, (i) matrix viscosity, (iii) voids, and (iv) humidity and temperature (Seretis et al., Citation2018). The water absorption characteristics of GNPs filled sisal/glass fiber reinforced hybrid nanocomposites presented in show a linear increase during the first days of soaking in water. This is due to the fact that the water molecules quickly diffused into micro-voids that exist in the composites. Upon increasing the soaking period, the water uptake by the sisal/hemp fiber composites reduced and finally reached a state of saturation. As the soaking period increased, the water absorption of sisal/glass fiber reinforced hybrid nanocomposites decreased and finally reached a saturation state. As expected, among fiber reinforced composites, SFC has the highest water absorption of nearly 8% after 35 days, followed by HSGFC0.This can be explained by the higher content of hemicellulose and cellulose in sisal fiber. HSGFC0, HSGFC0.25, HSGFC0.75, HSGFC1, GFC, and SGFC0.50 showed the lowest water absorption, respectively, especially at the saturation stage. The weight gains due to water absorption are shown in . It is evident from that the weight gains due to water absorption increased in the order: HSGFC0.50, GFC, HSGFC1, HSGFC0.75, HSGFC0.25, HSGFC0, and SFC. Further, it can be seen that the water absorption of the tested samples decreased in the order of (SFC, HSGFC0, HSGFC0.25, HSGFC0.75, SGFC1, GFC, and SGFC0.50) hybrid reinforced composite samples. Sisal fiber composites were observed to have higher water content.
Table 4. Sample weight change of composite and hybrid composites on water absorption test.
The sample weight changes of the composite and hybrid composites in the water absorption test are shown in . It was noticed from table that the water absorption percentage of 0.50% GNPs hybrid reinforced composite sample is very low compared to other hybrid laminated composites. The weight of the GNPs -reinforced composite sample decreased by 7.40% and 4.62%, respectively, upon water absorption. This demonstrates that the nano filler have a water barrier capacity that reduces water absorption in glass epoxy and hybrid composites reinforced with GNPs. This is due to the ability of the nano filler to restrict the intermolecular movement of the encircling glass/epoxy hybrid-reinforced composites, thereby delaying the relaxation of polymer chains and decreasing the diffusion of minute molecules through the composites. Nano filler in glass fibers and hybrid reinforced epoxy composites can create an effective water barrier that reduces water absorption, according to the findings (Balan et al., Citation2022; Bulut, Citation2017; Huang et al., Citation2011).
4.7. Thickness swelling
In an attempt to study the effect of moisture diffusion on the thickness of GNPs -filled sisal/glass hybrid nanocomposite samples, a thickness swelling test was conducted. The results obtained are presented in . It can be observed from that the GFC showed the higher dimensional stability, while the hybridized nanocomposite showed a higher rate of thickness swelling. Among the investigated GNPs filled sisal/glass hybrid composite specimens, SGFC50 showed higher stability and the SGFC0 showed the highest thickness swelling. This may be due to the high porosity and presence of voids on the surfaces of composites which leads to the change in dimensional stability. It was found that a lowest swelling thickness was obtained at SGFC50 of 4.58%. This is because GNPs has fewer pores, fewer cavities, and better fiber-matrix bonding, resulting in less thickness expansion and improved dimensional stability. The same trend was observed for water absorption. It can be concluded that the hybridized composites showed better resistance to water absorption and thickness swelling compared to pure sisal fiber reinforced composites. It can also be concluded that the weight gain of all hybrid composites was below 12% during the entire testing period.
Table 5. Thickness swelling of GNPs-filled sisal/glass hybrid nanocomposite.
4.7. Void content and density
It is well known that the trapped air or volatiles are present in the composites during the impregnation of fibre into the matrix or during the fabrication (Jawaid et al., Citation2011; Priyadarshini et al., Citation2023). During the fabrication and curing process, resin flow through the fibre layer affects: (i) fibre wetting in the composite, (ii) void content, (iii) fiber distribution. These factors can affect the properties and performance of composites (Alamri & Low, Citation2012). Owing to the presence of cavities, the mechanical and physical properties of the composites are expected to decrease. Therefore, it is essential to investigate the void content of composite specimens. shows the theoretical density, measured density, and % of voids of composites for various fiber compositions with different GNPs weight fraction.
Table 6. Details of voids content and density of composites.
Among the composite specimens examined, GFC exhibited (2.25%) the lowest void percentage compared to the other laminated composite plates. It was also observed that the hybrid composites with the lowest void content (7.75%) were 0.5% GNPs-reinforced composites, where air volatility and void content were less. However, the fabrication of the hybrid composites resulted in voids. Although it has been reported in the literature that the geometry and morphology of the filler material play a significant role in determining the void content, the nanoparticle-containing samples had comparable void contents. This can be attributed to the nanoparticles’ relatively uniform dispersion, because agglomeration leads to the formation of cavities in the sample (Chua et al., Citation1992; Kamaraj et al., Citation2020; Mallick, Citation2007; Mohan & Kishore, Citation1985; Starkova et al., Citation2013; Thiagamani et al., Citation2019).
The variation in the content with respect to the stacking order of the fibrous layers in the hybrid composites may be related to the percentage weight reduction of the composite from the matrix. Therefore, it is clear that the void content of the hybrid composites varied between 8% and 12% owing to the difference in fiber reinforcement geometry (diameter and lumen size). It is well understood that air bubbles can be trapped between the matrix and fibers during the hand layup technique used for impregnation. This can affect the flow of resin, void content, saturation of the fibers, and their distribution, all of which can have an impact on the mechanical and physical properties and performance of the composite material (Bodduru et al., Citation2023).
5. Conclusion
In this study, sisal, glass, GNPs-filled sisal/glass fiber reinforced hybrid composites reinforced with glass fibers were fabricated and their properties such as tensile strength, flexural, void content, thickness swelling, and water absorption characteristics were examined. The hybrid nanocomposite was fabricated using a vacuum bag-assisted hand lay-up technique. Different layup sequences are achieved by changing the position of the glass/sisal fiber layers, maintaining a total of five layers in each hybrid composite. The main conclusions are summarized and presented as follows:
The addition of 1wt.% GNPs nano filler to hybrid composites enhanced their tensile strength by 49.7% and their Young’s modulus by 60%. The interaction between the GNPs nanoparticles and the matrix material increases owing to improved surface bonding, which results in the dispersion of nanoparticles and the formation of cross-linked points. This led to an increase in the tensile strength of the composite material.
It was found that among the GNP reinforced hybrid composites, highest toughness of 16 J was reported by the composite containing 1 wt.% GNP.
Moreover, for 0.5 wt.% GNPs, the flexural strength and modulus reached a maximum of 306 MPa and 25 GPa, respectively, which were 228% and 362% greater than sisal/glass hybrid composites.
It was found that the water absorption percentage of 0.50 wt.% GNPs hybrid reinforced composite sample is very less water absorption by 4.62%, compared to other hybrid composites.
A lower void content was obtained for GNP reinforced hybrid containing; being lowest (7.75%) for 0.5 wt.% GNPs filled sisal/glass fiber reinforced polymer hybrid nanocomposite.
Furthermore, 0.5 wt.% GNPs hybrid composites demonstrate a decrease in burning rate from 11.22 mm/min to 7.6 mm/min and from 23.13 mm/min to 20.18 mm/min for horizontal and vertical flammability, respectively, compared to the hybrid composite containing no GNP. The addition of nano fillers to the materials was found to be beneficial because of the flame-retardant properties of GNPs, which not only prevented flame propagation but also aided in extinguishing the flames.
Authors’ contributions
The experiments were conducted by B.K and G. R, who also conceptualized the article and authored the initial draft of the paper. A. M and M.K.K were analysed the experimental data and was responsible for performing editing tasks, composing the review, and engaging in correspondence about the article. L.K.S were contributed the final draft and frame the paper and provided technical guidance and contributed to the experimental work during the writing process of the article. A.B.A were contributed in writing – review and editing, validation. All authors have read and approved the manuscript.
Data available statement
The data that support the findings of this study are available from the corresponding author upon reasonable request.
Disclosure statement
The authors declare that they have no known competing financial interests or personal relationships that could have influenced the work reported in this study.
Additional information
Notes on contributors
Bodduru Kamesh
Bodduru Kamesh is Assistant Professor of the Department of Mechanical Engineering (Design and Manufacturing) in the School of Engineering and Technology, Sharda University, India. He holds a full time Ph.D. from Sharda University, specializing in Honeycomb Cored Sandwich Composite on Static and Structural Dynamics. He earned his M. Tech. in Mechanical Engineering (Computer Aided Design and Manufacturing) from Gayatri Vidya Parishad College of Engineering (Autonomous), Visakhapatnam, and B. Tech in Mechanical Engineering from Kakinada Institute of Engineering & Technology affiliated to JNTU University Kakinada, Andhra Pradesh. His interests encompass Static, Mechanical and Dynamics of composite structures, Honeycomb Structures and Hybrid sandwich modelling. He has an impressive publication record with over twelve research papers in SCI indexed high-impact factor international journals.
Ravali Gude
Ravali Gude Currently, Ms. Gude is pursuing B.Tech. in Mechanical Engineering from Gayatri Vidya Parishad College of Engineering, India. She has done her schooling in Amar English medium school with 9.8 GPA. Her education involved projects investigating composite materials and mini projects like designing a Drum Brake in CATIA V5. Also, she has volunteered for the National Service Scheme (NSS). Her area of interest is in Material Science, Strength of Materials and Thermodynamics.
Amrita Maddamsetty
Amrita Maddamsetty Currently, Dr. Amrita is working as Associate Professor in the Department of Mechanical Engineering at Gayatri Vidya Parishad College of Engineering (Autonomous) (GVPCE), Visakhapatnam, India. She has completed her PhD from Jawaharlal Nehru Technological University Hyderabad. Dr. Amrita has 20+ tears of teaching and research experience and her area of specialization includes metal cutting, composites, nano-fluids and optimization techniques.
Mesfin Kebede Kassa
Mesfin Kebede Kassa has worked extensively in the area of computational structural mechanics and computational materials sciences. He did his Ph. D. from Sharda University and is currently associated with Kombolcha Institute of Technology, Wollo University, Kombolcha, Ethiopia and Ethiopian Aviation University, Addis Ababa, Ethiopia. He has publication is several reputed journals including Polymer Composites, International Journal of Structural Stability and Dynamics, Journal of Vibration Engineering & Technologies, Fibres and Polymers etc.
Lavish Kumar Singh
Lavish Kumar Singh has completed his B. Tech. in Mechanical Engineering from NIT Patna in 2012 and M. Tech. from NIT Calicut in 2014. Subsequently, he completed his Ph.D. from IIT Kharagpur. Currently, Dr. Singh is working as Assistant Professor in the School of Engineering, Jawaharlal Nehru University, India. He has more than 40 publications in different SCI/Scopus indexed journals. Dr. Singh has also presented his research work in several reputed conferences held in different IITs and abroad (Ireland and USA). His research of interest includes composite materials, nanomaterials, mechanical testing and microstructural analysis.
Ananda Babu Arumugam
Ananda Babu Arumugam is a Professor and Head of the Department of Mechanical Engineering in the School of Engineering and Technology, Sharda University, India. Dr. Ananda Babu obtained his doctorate from Vellore Institute of Technology (VIT), Vellore in the area of composite structural dynamics. He obtained his B.E-Manufacturing Engineering in College of Engineering, Guindy, Chennai, and his M.E- Engineering Design in PSG College of Technology, Coimbatore from Anna University. He has experience of about thirteen years in both teaching and research. His area of research interest includes dynamics of composite structures, damage modeling, hybrid materials modeling, and failure analysis of structures. He has published more than forty research papers in peer-reviewed international journals with high impact factors.
References
- Alamri, H., & Low, I. M. (2012). Effect of water absorption on the mechanical properties of nano-filler reinforced epoxy nanocomposites. Materials & Design, 42, 214–222. https://doi.org/10.1016/j.matdes.2012.05.060
- Alamri, H., & Low, I. M. (2013). Effect of water absorption on the mechanical properties of nanoclay filled recycled cellulose fibre reinforced epoxy hybrid nanocomposites. Composites Part A: Applied Science and Manufacturing, 44, 23–31. https://doi.org/10.1016/j.compositesa.2012.08.026
- Ary Subagia, I. D. G., Tijing, L. D., Kim, Y., Kim, C. S., Vista Iv, F. P., & Shon, H. K. (2014). Mechanical performance of multiscale basalt fiber–epoxy laminates containing tourmaline micro/nano particles. Composite Part B, 58, 611–617. https://doi.org/10.1016/j.compositesb.2013.10.034
- Balan, G. S., Balasundaram, R., Chellamuthu, K., Gopan, S. N., Dinesh, S., Vijayan, V., Sathish, T., & Rajkumar, S. (2022). Flame resistance characteristics of woven jute fiber reinforced fly ash filled polymer composite. Journal of Nanomaterials, 2022, 1–12. https://doi.org/10.1155/2022/9704980
- Bodduru, K., Singh, L. K., Amrita, M., Anbumani, P., & Singh, A. P. (2023). Influence of MXene and carbon nanotube on the mechanical properties of sisal fibre/glass fibre reinforced epoxy composite laminates. Materials Today: Proceedings. https://doi.org/10.1016/j.matpr.2023.02.446
- Bodduru, K., Singh, L. K., Kassa, M. K., Maddamsetty, A., & Arumugam, A. B. (2023). Influence of MXene, graphene nanoplatelet and multi-walled carbon nanotube on mechanical properties, swelling and flammability behaviour of hybrid sisal/glass fibre reinforced epoxy laminate composites. Materials Research Express, 10(4), 045002. https://doi.org/10.1088/2053-1591/acc82e
- Bulut, M. (2017). Mechanical characterization of basalt/epoxy composite laminates containing graphene nano pellets. Composite Part B, 122, 71–78. https://doi.org/10.1016/j.compositesb.2017.04.013
- Chua, P. S., Dai, S. R., & Piggott, M. R. (1992). Mechanical properties of the glass fibre-polyester interphase. Journal of Materials Science, 27(4), 913–918. https://doi.org/10.1007/BF01197641
- Fuqua, M. A., Huo, S., & Ulven, C. A. (2012). Natural fiber reinforced composites. Polymer Reviews, 52(3), 259–320. https://doi.org/10.1080/15583724.2012.705409
- Gupta, M. K., & Deep, V. (2019). Effect of water absorption and stacking sequences on the properties of hybrid sisal/glass fibre reinforced polyester composite. Proceedings of the Institution of Mechanical Engineers, Part L: Journal of Materials: Design and Applications, 233(10), 2045–2056. https://doi.org/10.1177/1464420718811867
- Huang, G., Gao, J., Wang, X., Liang, H., & Ge, C. (2011). How can graphene reduce the flammability of polymer nanocomposites? Materials Letters, 66(1), 187–189. https://doi.org/10.1016/j.matlet.2011.08.063
- Jarukumjorn, K., & Suppakarn, N. (2009). Effect of glass fiber hybridization on properties of sisal fiber–polypropylene composites. Composites Part B: Engineering, 40(7), 623–627. https://doi.org/10.1016/j.compositesb.2009.04.007
- Jawaid, M. H. P. S., Abdul Khalil, H. P. S., Noorunnisa Khanam, P., & Abu Bakar, A. (2011). Hybrid composites made from oil palm empty fruit bunches/jute fibres: Water absorption, thickness swelling and density behaviours. Journal of Polymers and the Environment, 19(1), 106–109. https://doi.org/10.1007/s10924-010-0203-2
- Joseph, K., Thomas, S., & Pavithran, C. (1995). Sisal fibre reinforced polyethylene composites: Effect of isocyanate treatment. SB Academic Review, Changanacherry, 6, 85.
- Joshi, S. V., Drzal, L. T., Mohanty, A. K., & Arora, S. (2004). Are natural fiber composites environmentally superior to glass fiber reinforced composites. Composites Part A: Applied Science and Manufacturing, 35(3), 371–376. https://doi.org/10.1016/j.compositesa.2003.09.016
- Kamaraj, M., Dodson, E. A., & Datta, S. (2020). Effect of graphene on the properties of flax fabric reinforced epoxy composites. Advanced Composite Materials, 29(5), 443–458. https://doi.org/10.1080/09243046.2019.1709679
- Khalil, A., Khalil, H. P. S. A., Jawaid, M., & Abu Bakar, A. (2011). Woven hybrid composites. BioResources, 6(2), 1043–1052. https://doi.org/10.15376/biores.6.2.1043-1052
- Kumar, A., Sharma, K., & Dixit, A. R. (2019). A review of the mechanical and thermal properties of graphene and its hybrid polymer nanocomposites for structural applications. Journal of Materials Science, 54(8), 5992–6026. https://doi.org/10.1007/s10853-018-03244-3
- Kumar, A., Sharma, K., & Dixit, A. R. (2021). A review on the mechanical properties of polymer composites reinforced by carbon nanotubes and graphene. Carbon Letters, 31(2), 149–165. https://doi.org/10.1007/s42823-020-00161-x
- Kumar, A., Sharma, K., & Dixit, A. R. (2022). Effects of various functional groups in graphene on the tensile and flexural properties of epoxy nanocomposites: a comparative study. Fullerenes, Nanotubes and Carbon Nanostructures, 30(11), 1123–1133. https://doi.org/10.1080/1536383X.2022.2077332
- Kumar, A., Sharma, K., & Dixit, A. R. (2023). Tensile, flexural and interlaminar shear strength of carbon fiber reinforced epoxy composites modified by graphene. Polymer Bulletin, 80(7), 7469–7490. https://doi.org/10.1007/s00289-022-04413-w
- Le Thi, T. T., Gauthier, H., Gauthier, R., Chabert, B., Guillet, J., Luong, B. V., & Nguyen, V. T. (1996). Realization of polypropylene/sisal fiber composites by reactive extrusion. Journal of Macromolecular Science, Part A: Pure and Applied Chemistry, 33(12), 1997–2004. https://doi.org/10.1080/10601329608011024
- Li, X., Tabil, L. G., & Panigrahi, S. (2007). Chemical treatments of natural fiber for use in natural fiber-reinforced composites: a review. Journal of Polymers and the Environment, 15(1), 25–33. https://doi.org/10.1007/s10924-006-0042-3
- Mallick, P. K. (2007). Fiber-reinforced composites: materials, manufacturing, and design. CRC Press.
- Mohan R., & Kishore. (1985). Jute-glass sandwich composites. Journal of Reinforced Plastics and Composites, 4(2), 186–194. https://doi.org/10.1177/073168448500400203
- Namdev, A., Purohit, R., & Telang, A. (2023). Impact of graphene nano particles on tribological behaviour of carbon fibre reinforced composites. Advances in Materials and Processing Technologies, 9, 1–18. https://doi.org/10.1080/2374068X.2023.2189670
- Namdev, A., Telang, A., & Purohit, R. (2022). Synthesis and mechanical characterization of epoxy hybrid composites containing graphene nanoplatelets. Proceedings of the Institution of Mechanical Engineers, Part C: Journal of Mechanical Engineering Science, 236(14), 7984–7998. https://doi.org/10.1177/09544062221081286
- Namdev, A., Telang, A., & Purohit, R. (2023). Investigation of mechanical behavior of graphene nanoplatelets and carbon fiber-reinforced hybrid laminate composites under different environment aging conditions. Proceedings of the Institution of Mechanical Engineers, Part E: Journal of Process Mechanical Engineering, 095440892211507. https://doi.org/10.1177/09544089221150731
- Naveen, J., Jawaid, M., Zainudin, E. S., Thariq Hameed Sultan, M., & Yahaya, R. (2019). Improved mechanical and moisture-resistant properties of woven hybrid epoxy composites by graphene nanoplatelets (GNP). Materials, 12(8), 1249. https://doi.org/10.3390/ma12081249
- Priyadarshini, S., Singh, L. K., Kaithwas, C. K., & Soren, S. (2023). A review on thermo‐mechanical durability of glass fiber/polymer composites in water. In Macromolecular Symposia, 407(1), 2200124. https://doi.org/10.1002/masy.202200124
- Ramesh, M., Bhoopathi, R., Deepa, C., & Sasikala, G. (2018). Experimental investigation on morphological, physical and shear properties of hybrid composite laminates reinforced with flax and carbon fibers. Journal of the Chinese Advanced Materials Society, 6(4), 640–654. https://doi.org/10.1080/22243682.2018.1534609
- Sahoo, S., & Jena, P. C. (2022). Preparation and characterization of hybrid laminated composite beams. Advances in Materials and Processing Technologies, 8(sup2), 899–912. https://doi.org/10.1080/2374068X.2021.1953924
- Sanadi, A. R., Prasad, S. V., & Rohatgi, P. K. (1986). Sunhemp fibre-reinforced polyester. Journal of Materials Science, 21(12), 4299–4304. https://doi.org/10.1007/BF01106545
- Seretis, G. V., Theodorakopoulos, I. D., Manolakos, D. E., & Provatidis, C. G. (2018). Effect of sonication on the mechanical response of graphene nanoplatelets/glass fabric/epoxy laminated nanocomposites. Composite Part B, 147, 33–41. https://doi.org/10.1016/j.compositesb.2018.04.034
- Starkova, O., Chandrasekaran, S., Prado, L. A. S. A., Tölle, F., Mülhaupt, R., & Schulte, K. (2013). Hydrothermally resistant thermally reduced graphene oxide and multi-wall carbon nanotube-based epoxy nanocomposites. Polymer Degradation and Stability, 98(2), 519–526. https://doi.org/10.1016/j.polymdegradstab.2012.12.005
- Thiagamani, S. M. K., Krishnasamy, S., Muthukumar, C., Tengsuthiwat, J., Nagarajan, R., Siengchin, S., & Ismail, S. O. (2019). Investigation into mechanical, absorption and swelling behaviour of hemp/sisal fibre reinforced bioepoxy hybrid composites: Effects of stacking sequences. International Journal of Biological Macromolecules, 140, 637–646. https://doi.org/10.1016/j.ijbiomac.2019.08.166
- Venkateshwaran, N., & ElayaPerumal, A. (2012). Mechanical and water absorption properties of woven jute/banana hybrid composites. Fibers and Polymers, 13(7), 907–914. https://doi.org/10.1007/s12221-012-0907-0
- With, R., Fiber, C., & Trihydrate, A. (2017). Flammability behavior of green polyurethane composite [Paper presentation]. Meeting on Aeronautical Composite Materials and Structures – MACMS, São Carlos School of Engineering – University of São Paulo. http://soac.eesc.usp.br/index.php/macms/macms2017.
- Yadav, P. S., Purohit, R., & Namdev, A. (2022). Physical and mechanical properties of hybrid composites using Kevlar fibre and nano-SiO2. Advances in Materials and Processing Technologies, 8(sup4), 2057–2069. https://doi.org/10.1080/2374068X.2022.2034312
- Zhong, L. X., Fu, S. Y., Zhou, X. S., & Zhan, H. Y. (2011). Effect of surface microfibrillation of sisal fibre on the mechanical properties of sisal/aramid fibre hybrid composites. Composites Part A: Applied Science and Manufacturing, 42(3), 244–252. https://doi.org/10.1016/j.compositesa.2010.11.010