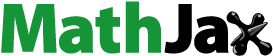
Abstract
The traditional cooking of dry maize grain for preparing either boiled maize (nifro) or roasted maize (qollo) in Ethiopia is preceded by soaking dry maize in water for a certain time. This study aimed to assess the practice of soaking dry maize grain and determine the effect of soaking water volume (0, 200 or 300 ml per 100 g), soaking time (12 or 48 h), soaking water temperature (warm or cold water) and type of cooking method (boiling and roasting) on the aflatoxin content of traditional Ethiopian cooked maize, nifro and qollo. The survey and laboratory experiments used semi-structured interview questionnaire and fractional factorial design, respectively. Aflatoxins were extracted from aflatoxigenic Aspergillus flavus spores inoculated onto raw maize, soaked in water and finally cooked by boiling or roasting of the soaked maize grain. Cleanup was done using Immuno Affinity Column and analyzed using Ultra High Performance Liquid Chromatography with fluorescence detector. Soaking dry maize grain in excess water (300 ml water/100 g maize) varying the soaking water temperature and duration before boiling and roasting had resulted in significant total aflatoxin reduction (p < .05) ranging from 33.6% to 89.9% in boiled maize and more than 89% in roasted maize compared to the control. The study results indicated that roasting of soaked maize grain resulted in a considerably lower aflatoxin content compared to boiling of soaked maize grain. Dry maize grain soaking in excess water and cold water before cooking showed a better reduction of aflatoxin.
REVIEWING EDITOR:
1. Introduction
Africans use maize grain in the preparation of whole grain foods, wet-ground maize foods, Sourdough and dumplings, porridges, beverages, bread and snacks (Ekpa et al., Citation2018). Sub-Saharan African countries consume about 450 g of maize per person per day (Ekpa et al., Citation2019). 23.3% of Kenyans consume githeri, shelled maize grain boiled in water with beans at dinner time and 40% of Zimbabweans use shelled maize grain for mutakura preparation; West Africans boil shelled maize with cow pea (Vigna unguiculata), but East Africans boil it with kidney bean (Phaseolus vulgaris), meanwhile, East Africans consume roasted maize with salt, chilies and lime, whereas West Africans eat it with coconuts, garden eggs, nuts, or African pears (Dacryodes edulis) (Ekpa et al., Citation2019). In Ethiopia, about 94 g of maize per person per day is consumed (Ranum et al., Citation2014). Maize in Ethiopia is used in the preparation of foods such as injera (Ethiopian pan cake-like flat bread), bread, porridge, nifro, shorba, and it is also included in different proportions in other foods (Mohammed et al., Citation2022). Ethiopian traditional beverages such as borde, tella and checka are also made from maize grain (Mohammed et al., Citation2022). Consuming whole maize grain foods such as boiled green maize (nifro) and roasted green maize (qollo) is common in Ethiopia during harvest. While, dry maize grain is also processed into nifro and qollo. However, maize is susceptible to aflatoxigenic Aspergillus section Flavi invasion and the subsequent aflatoxin contamination (Leslie & Logrieco, Citation2014).
Aflatoxins, in either acute or chronic forms, are highly toxic compounds and warm and humid weather conditions favor optimal mold growth and aflatoxin production; the main aflatoxin types are B1, B2, G1 and G2; aflatoxin B1 is the most powerful natural carcinogen (Kortei et al., Citation2021). Studies on aflatoxin mitigation have ranged from the method of sorting out the contaminated produce from the uncontaminated ones during the cleaning process (Yang, Citation2019) to the study of molecular genetics of toxigenic Aspergillus flavus growth and aflatoxin biosynthesis in varying environments by affecting the Mitogen-Assisted Protein Kinase (MAPK) signaling pathway with cyclic adenosine monophosphate (cAMP)-Protein Kinase A (PKA) so as to control aflatoxin contamination of agricultural produce in the field (Amare & Keller, Citation2014; Uka et al., Citation2020). In an effort to identify the role of temperature in inhibiting aflatoxin biosynthesis, apart from the suitable temperature for aflatoxin biosynthesis (30 °C), Yu et al. (Citation2011) and Chang et al. (Citation2015) noted that the regulatory genes, which are vital for aflatoxin production in the aflatoxin cluster (aflR and aflS) of the transcription process in A. flavus, are highly affected at 37 °C. Meanwhile, Worku et al. (Citation2019), Assaye et al. (Citation2016) and Yilma et al. (Citation2019) studied the aflatoxin level of stored maize collected from the different parts of Ethiopia and aflatoxigenic fungi growth using potato dextrose agar medium at 25 °C. The interactive effects of an increase in ambient temperature and grain temperature, along with increased water activity and increased carbon dioxide levels in the environment due to climate change, result in an increase in the aflatoxin content of stored maize (Medina et al., Citation2014). Several authors (Ayele et al., Citation2022; Ayelign et al., Citation2017; Boshe et al., Citation2020; Gebreegziabher et al., Citation2022) noted the aflatoxin contamination of agricultural produce in different parts of Ethiopia in relation to its possible presence in lactating women and young children, and their study results emphasize the need for awareness creation on aflatoxin mitigation methods during food processing and complementary food preparations.
Among the prevention, decontamination and detoxification methods used, the most effective and efficient strategy is to prevent the entry of aflatoxin-contaminated maize into supply chain (Leslie & Logrieco, Citation2014; Mir et al., Citation2021). Besides, good agricultural and storage practices also play a significant role in reducing the aflatoxin level of maize (Karlovsky et al., Citation2016). Use of chemical treatments in alleviating aflatoxins during food processing poses an increased health risk and diminishes organoleptic characteristics (Karlovsky et al., Citation2016). However, physical methods (cleaning, separating the screenings, washing, aqueous extraction, dehulling and milling) along with food processing minimize aflatoxin exposure significantly by removing the toxins, converting them to less toxic metabolites and adsorbing the toxins onto food matrix structures (Fandohan et al., Citation2005; Karlovsky et al., Citation2016). Food processing plays a key role in mitigating the aflatoxin content of foods, though not able to totally destroy aflatoxin in foods (Bullerman & Bianchini, Citation2014; Karlovsky et al., Citation2016; Leslie & Logrieco, Citation2014; Ryu et al., Citation2008). Food processing techniques mitigate aflatoxin in foods while maintaining nutritional and organoleptic quality (Fandohan et al., Citation2005; Karlovsky et al., Citation2016). For considerable reduction of aflatoxin in foods, heating temperature above 150 °C is required (Kabak, Citation2009; Kamimura, Citation1999; Rustom, Citation1997). However, Fandohan et al. (Citation2005) reported a partial reduction of aflatoxins during ordinary home cooking below 150 °C when processed under pressure and in moist conditions.
Survey studies in Ethiopia regarding exposure to aflatoxin contamination in foods are mainly related to poor storage and food processing practices in households (Beyene et al., Citation2016). Besrat and Gebre (Citation1981) studied injera preparation and aflatoxin contamination, and they concluded that no aflatoxin was detected in the prepared and analyzed injera samples. Geyid and Maru (Citation1987) also observed no aflatoxin in prepared injera up to three days but detected aflatoxin on the fourth day of stored injera made of maize and sorghum and on the seventh day of stored injera made of teff. In Ethiopia, consuming roasted and/or boiled maize on the cob is common during maize harvesting. Moreover, shelled maize grain is also consumed as boiled maize (nifro) and roasted maize (qollo) in households and at social ceremonies. Before it is boiled or roasted, dry maize is normally soaked in water for a certain period of time.
However, the role of traditional Ethiopian maize-based food processing techniques in reducing aflatoxin content was not reported in published research as to the authors’ knowledge. There is an information gap on the effect of traditional cooking of maize grain, either as boiled maize (nifro) or roasted maize (qollo), on the fate of aflatoxin. Thus, this study aimed to assess the practice of soaking dry maize grain and to determine the effect of processing parameters (soaking water volume, soaking time and soaking water temperature) and cooking methods (boiling and roasting) on the fate of aflatoxin and to produce important information regarding the level of aflatoxin exposure in consumers of traditionally cooked maize.
2. Materials and methods
2.1. Study sites
A survey on the practices of boiled dry maize (nifro) and roasted dry maize (qollo) processing was conducted at Bako Tibe district (9° 00′ 0.00’’ N Latitude and 37° 09′ 60.00’’ E Longitude), Walmara district (8°50’–9°15’N Latitude and 38°25’–38°45’ E Longitude), Tiyo district (7° 45’ 55’’ to 8° 02’ 02’’ N Latitude and 38° 56’ 42’’ to 39° 18’ 31’’ E Longitude) and Adama district (8.14°-8.44° N Latitude and 39.04°-39.25° E Longitude), Oromia National Regional State (ONRS), Ethiopia ().
Figure 1. Map of study districts (a) Bako Tibe district (b) Walmara district (c) Tiyo district (d) Adama district, ONRS, Ethiopia.
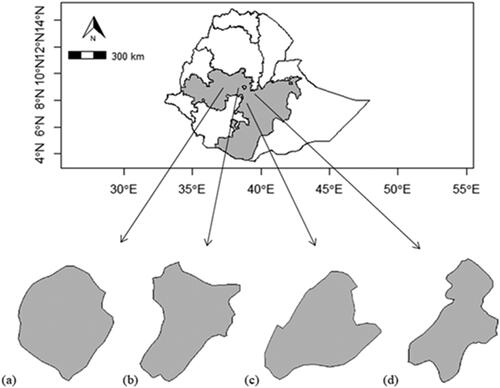
Aflatoxigenic A. flavus isolation, inoculum preparation and inoculation, soaking, boiling and roasting procedures of the experiment were done at Holeta Agricultural Research Center, Holeta, Ethiopia, which has an annual mean relative humidity, minimum temperature and maximum temperature of 60.6%, 6.1 °C and 22 °C, respectively (Galano et al., Citation2008; Gebremedhin et al., Citation2022). Total aflatoxin content was analyzed at the Ethiopian Conformity Assessment Enterprise laboratory, Addis Ababa, Ethiopia.
2.2. Survey study and laboratory experiment procedures
Women (n = 150) were selected and interviewed based on a systematic random sampling technique using semi-structured questionnaire (). Then, based on the survey results, a laboratory experiment was carried out in accordance with the practices of dry maize grain soaking, boiling and roasting in the study areas (). Dry maize grain of Bako-Hybrid 661 maize variety was used for the traditional cooking methods studied. Aspergillus flavus inoculated but not soaked maize grain in sterilized distilled water was used as a control for comparing the reduction in the total aflatoxin content after soaking and cooking maize grain. All maize grain samples were sterilized before A. flavus inoculation through autoclaving.
Figure 2. Flow chart of the study design (a) survey on the practices of boiled dry maize (nifro) and roasted dry maize (qollo) processing in the study areas (b) laboratory experiment procedure onto Aspergillus flavus inoculated maize viz. soaking in sterilized distilled water and then boiling in sterilized distilled water or soaking in sterilized distilled water and then roasting based on the study areas practice.
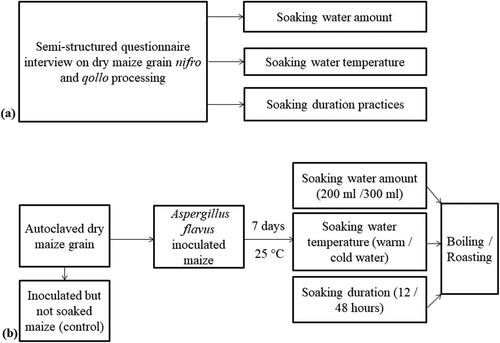
The survey study and laboratory experiment used semi-structured questionnaire interview and fractional factorial design, respectively. The replicated treatments (T) in the laboratory experiment were:
T1: maize grain was only autoclaved but neither A. flavus inoculated nor soaked in sterilized distilled water.
T2: maize grain was inoculated with A. flavus but not soaked in sterilized distilled water.
T3: maize grain was inoculated and then soaked in 200 ml of warm water for 12 h (warm water refers to boiled sterilized distilled water whose temperature decreased to 25–30 °C) and then it was boiled.
T4: maize grain was inoculated and then soaked in 200 ml of warm water for 12 h and then it was roasted.
T5: maize grain was inoculated and then soaked in 200 ml of cold water for 48 h (cold water refers to temperature of the sterilized distilled water used which decreased up to 14 °C, along with the lower ambient temperature at Holeta, Ethiopia) and then it was boiled.
T6: maize grain was inoculated and then soaked in 200 ml of cold water for 48 h and then it was roasted.
T7: maize grain was inoculated and then soaked in 300 ml of warm water for 48 h and then it was boiled.
T8: maize grain was inoculated and then soaked in 300 ml of warm water for 48 h and then it was roasted.
T9: maize grain was inoculated and then soaked in 300 ml of cold water for 12 h and then it was boiled.
T10: maize grain was inoculated and then soaked in 300 ml of cold water for 12 h and then it was roasted.
2.3. Aflatoxigenic Aspergillus flavus isolation and inoculum preparation
Direct plating method was used for the isolation of aflatoxigenic A. flavus. Maize grains were surface sterilized with 10% sodium hypochlorite solution for 1 min, followed by rinsing in sterile distilled water for 1 min. Surface sterilized maize grains were then placed on freshly prepared potato dextrose agar (PDA) media. Five maize grains were put on PDA media per plate. In the freshly prepared PDA media, 50 mg/L streptomycin was added to suppress the growth of bacteria in petri plates. The plates were then incubated for three days at 25 °C. A pure culture of A. flavus was obtained by transferring the colonies to new PDA plates using sterile toothpicks and incubating the plates for seven days at 25 °C (Assaye et al., Citation2016) and identified according to Klich (Citation2002). After incubation at 25 °C, spores were harvested by adding sterilized distilled water (10 ml for each plate). The spore suspension obtained was filtered using three layers of sterilized cheesecloth and then the spores were counted using a hemocytometer and brought to a final concentration of 106 conidia per milliliter (Reddy et al., Citation2011).
Several researchers used one medium (Potato Dextrose Agar) for isolating A. flavus (Assaye et al., Citation2016; Chandrasekharan et al., Citation2014; Reddy et al., Citation2011; Shekhar et al., Citation2017), whereas researchers aiming at the identification and characterization of the wide diversity of fungi use two or more media (favoring the specific substrate composition for the growth of each fungi) to isolate and characterize the different species of fungi.
2.4. Aspergillus flavus inoculation onto maize grain
Before inoculating the aflatoxigenic A. flavus onto maize grain, it was ascertained using an ammonia vapor test (Shekhar et al., Citation2017) and a UV light test (Chandrasekharan et al., Citation2014). Each 100 g of maize grain (16% moisture content on a wet basis) was sterilized in an Erlenmeyer flask at 121 °C for 20 min before being inoculated with 1 ml of A. flavus spore suspension (106 conidia/ml) and incubated at 25 °C for seven days (Atanda & Olopade, Citation2013; Reddy et al., Citation2011; Sultana et al., Citation2015).
2.5. Preparation of boiled (nifro) and roasted (qollo) maize
Boiled maize (nifro): after discarding the soaking water, each 100 g of soaked maize grain was cooked in a glass beaker containing sterilized distilled water (1000 ml) for 90 min (Fufa et al., Citation2003).
The boiled maize grain was aseptically transferred to a drying oven and dried at 40 °C for 48 h; in the meantime, the roasted maize was kept aseptically, sealed in a polyethylene bag and stored at 4 °C until analysis (Pérez-Flores et al., Citation2011).
Roasted maize (qollo) was prepared with modification from Fufa et al. (Citation2003): after discarding the soaking water, each 100 g of soaked maize grain was roasted as a thin layer on WASS® digital grill (mitad) (WASS Electronics Inc., Virginia, USA) at 150 °C for 30 min while being stirred to achieve uniformity.
2.6. Extraction of aflatoxins
Acetonitrile, methanol, sodium chloride, ultrapure water and phosphate-buffered saline (PBS) were used. PBS, pH 7.4 was prepared using NaCl 8 g l−1, KCl 0.2 g l−1, anhydrous Na2HPO4 1.16 g l−1 and KH2PO4 0.2 g l−1 with 900 ml of water and then adjusting the pH with HCl (0.1 M) or NaOH (0.1 M). Aflatoxin standards were purchased from Sigma Aldrich (St. Louis, MO, USA). Reagents for UHPLC separation were filtered through 0.45 µm and 0.2 µm membrane (Merck Chemicals, Darmstadt, Germany).
The aflatoxin extraction, detection and quantification were carried out based on Mamo et al. (Citation2023) with some modifications at Ethiopian Conformity Assessment Enterprise, Addis Ababa, Ethiopia.
Maize grain (25 g) was ground and homogenized in a polytron homogenizer for 10 min at 6000 rpm. 125 ml of methanol: water (80:20) was then added, and the mixture was filtered through fast-fluted filter paper. Then 15 ml of the extract was mixed well with 30 ml of phosphate buffered saline (PBS). 15 ml of the diluted extract was then passed through the Immuno Affinity Column (AflaTest® IAC) and washed with 10 ml of deionized water and then dried by passing air through it. It was then eluted onto a 4 ml vial with 3 ml of methanol and evaporated with a nitrogen stream at 40 °C. Derivatization: 200 µl hexane and 200 µl TFA (triflouroacetic acid) vortexed for 30 s then incubated at 60 °C for 10 min. Then, it was dried using a gentle stream of nitrogen at 40 °C and reconstituted using the mobile phase, deionized water: methanol: acetonitrile (60: 25: 15 v/v/v) at a flow rate of 1 ml/min and vortexed. Eventually, it was filtered using a syringe filter and transferred to an Ultra High Performance Liquid Chromatography (UHPLC) vial. The filtered sample was then injected into Waters® e2695 Ultra-High Performance Liquid Chromatography (UHPLC) connected to multi wavelength fluorescence detector, eclipse plus C18 column (100 mm × 4.6 mm; particle size: 3.5 μm) (Waters, Milford, USA), having a quaternary solvent delivery system, an auto-sampler and Waters Empower data software.
2.7. Statistical analysis
Design of experiment (DOE) was used to create and analyze fractional factorial design in Minitab® (Minitab version 17 statistical software; Minitab Inc., Pennsylvania, USA). Map of survey study districts was done using GADM database of Global administrative areas by R statistical software version 4.0.3 (The R Foundation for Statistical Computing). Descriptive statistics were used to summarize the data. Both dry maize grain processing share a common process of soaking in water () before boiling and roasting; when the value falls below the detection limit, the percentage reduction of the aflatoxin content compared to the control is calculated based on the values obtained on one of the food processes and regressed proportionally onto the encountered value below the detection limit of the other dry maize process studied, the lower bound (Zero) and upper bound (the detection limit) based on the appropriate statistical method with respect to the number of samples analyzed. Minitab statistical software was used to draw graphs and to test homogeneity of variance using Levene test. All data were subjected to one way & two way analysis of variance (ANOVA); where data normality and equal variance assumptions were met, Tukey test was used, if not, Games-Howell test was used to separate the means when significant differences was found among treatments at 5% level of significance.
3. Results and discussion
The method validation of Ultra High Performance Liquid Chromatography (UHPLC) for aflatoxin analysis of maize samples was done according to the Association of Official Analytical Chemists (Citation2006) and U.S. Food and Drug Administration (USFDA, Citation2002). The limit of detection were 0.05 ppb for AFB1, 0.02 ppb for AFB2, 0.05 ppb for AFG1 and 0.02 ppb for AFG2. The limit of quantification values were 0.165 ppb for AFB1, 0.066 ppb for AFB2, 0.165 ppb for AFG1 and 0.066 ppb for AFG2. The limit of detection and limit of quantification of the UHPLC were found using a seven-point calibration curve by injecting aflatoxins standard in the range of 0.5 µg kg−1 to 10 µg kg−1 (0.5, 1, 2, 3, 5, 7 and 10 µg kg−1). Calibration curves were separately prepared for each aflatoxin, obtained using the linear least squares regression procedure of peak area versus concentration. The regression coefficients (R2) of the line were 0.9989 for AFB1, 0.9987 for AFB2, 0.9986 for AFG1 and 0.9987 for AFG2, which is in line with the USFDA’s (2002) regression coefficient value of acceptable fit (>0.998) for aflatoxin analysis. The percentage recovery of the aflatoxin standard was found by injecting 2 µg kg−1 aflatoxin into the maize sample. The spike volume is calculated based on EquationEquation (1)(1)
(1) (Kortei et al., Citation2021).
(1)
(1)
The percentage recovery of the aflatoxin standard is calculated using EquationEquation (2)(2)
(2) (Kortei et al., Citation2021).
(2)
(2)
The percentage recoveries were 94.1% for AFB1, 97.9% for AFB2, 89.8% for AFG1 and 89.4% for AFG2, which agrees well with the acceptable percentage of recovery (80 to 120%) described by the USFDA (2002). Moreover, the relative standard deviation of repeatability and reproducibility of the method were acceptable based on the USFDA standard.
Based on the survey study questionnaire (the types of maize-based foods frequently consumed in the study areas, boiled dry maize (nifro) and roasted dry maize (qollo) processing, along with dry maize grain soaking water amount, soaking water temperature and soaking duration practices in the study areas during dry maize grain processing to nifro and qollo), the laboratory experiment of this study was carried out following the practices of dry maize grain soaking, boiling and roasting in the survey study areas (). The results of the survey study revealed that the majority of the study participants (68.7%, n = 150) used 200 ml of cold water for soaking 100 g of dry maize grain for a duration of 12 h, which is significantly different (p < .05) from 300 ml of cold water soaking for 48 h (13.3%, n = 150) (). Soaking maize grain in hot/warm water for 12 to 48 h for softening the kernels results in increased growth of pathogenic micro-organisms (Adisa & Enujiugha, Citation2020).
Figure 3. Percentage of the dry maize grain soaking practice while varying the amount of water used (ml), soaking water temperature and time (hours (h)) before boiling or roasting (n = 150). Means followed by the same letter are not significantly different (p > .05).
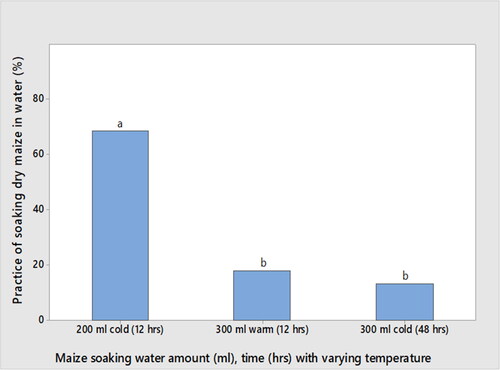
3.1. Effect of soaking water volume
The study result showed that soaking dry maize grain in water (300 ml water/100 g of maize) varying the soaking water temperature and duration before boiling and roasting had resulted in significant aflatoxin reduction (p < .05) ranging from 33.6% to 89.9% in boiled maize and more than 89% (p < .05) in roasted maize compared to the control (100% total aflatoxin remained in the inoculated but not soaked maize grain). 82% aflatoxin reduction was observed during rice cooking with an excess amount of water (Fasiha et al., Citation1979). Approximately 39-42% aflatoxin removal was found during wet milling from steeped maize into the steeping water (Scott, Citation1984; Yahl et al., Citation1971). Washing wheat was able to remove about 40% of aflatoxin B1 (Hwang & Lee, Citation2006). Adding excess water during maize grits boiling removed 34–53% of aflatoxin in boiled maize grits (Stoloff & Trucksess, Citation1981). Increasing the water content during heating wheat grain reduced the aflatoxin content higher than dry heating; an increase in moisture content at 85–95 °C destroyed aflatoxin with an opening at its lactone ring (Hwang & Lee, Citation2006; Mann et al., Citation1967; Torres et al., Citation2001). Soaking and steaming reduced the aflatoxin B1 content of foods (Fardiaz, Citation1997). Traditional food processing techniques lower the aflatoxin content of processed food products during washing (Fandohan et al., Citation2005; Jalili, Citation2016) and steeping (Leslie & Logrieco, Citation2014; Matumba et al., Citation2009; Ryu et al., Citation2008) before further processing.
Soaking maize in excess of cold water (300 ml/100 g maize) for 12 h significantly reduced (p < .05) the total aflatoxin percentage remained (7.6%) compared to soaking maize in excess of warm water (300 ml/100 g maize) for 48 h, which retained 66.4% total aflatoxin in boiled maize ().
Figure 4. Mean (±SD) of total aflatoxin percentage remained in boiled maize (nifro) compared to the control. Means displayed with different letters are significantly different (p < .05). T5: inoculated and soaked maize with 200 ml of cold water for 48 h and then boiled; T7: inoculated and soaked maize with 300 ml of warm water for 48 h and then boiled; T9: inoculated and soaked maize with 300 ml of cold water for 12 h and then boiled.
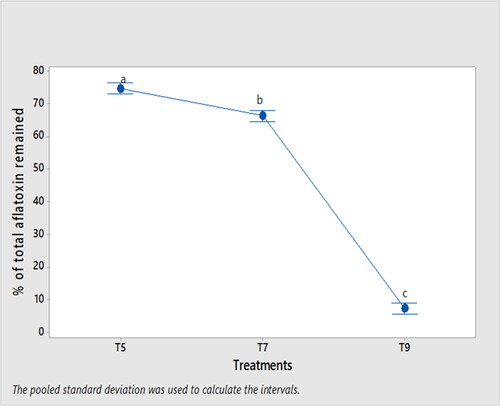
3.2. Effect of soaking time
The soaking period (12 h or 48 h) before maize boiling did not show a significant effect (p = .892) in decreasing the total aflatoxin content, though there was 5.6% decrease in total aflatoxin content after 48 h of soaking compared to 12 h of soaking. However, increasing the soaking warm water volume from 200 ml to 300 ml along with increasing the soaking time from 12 h to 48 h significantly reduced (p < .05) the total aflatoxin content of boiled maize by 53.3%. Soaking tigernuts in water for 48 h reduced the aflatoxin content by 12.95% (Roby & Samah, Citation2018). Maize grain soaked for more than 12 h removed aflatoxin during maize dehulling (Mutungi, Citation2006). Meanwhile, fumonisin B1 was also found in the steeping water after 48 h of maize soaking period (Fandohan et al., Citation2005). Moreover, increasing maize soaking duration in water to 72 h reduced the aflatoxin content of maize by 80% during the maize dehulling process (Matumba et al., Citation2009). 72% total aflatoxin reduction was found after two days of maize soaking in water (Assohoun et al., Citation2013).
shows that soaking maize in excess of cold water (300 ml/100 g maize) for 12 h significantly reduced the total aflatoxin percentage remaining (4.2%) compared to soaking maize in excess of warm water (300 ml/100 g maize) for 48 h, which retained 7.6% total aflatoxin in roasted maize (p < .05).
Figure 5. Mean (±SD) of total aflatoxin percentage remained in roasted maize (qollo). Means displayed with different letters are significantly different (p < .05). T4: inoculated and soaked maize with 200 ml of warm water for 12 h and then roasted; T8: inoculated and soaked maize with 300 ml of warm water for 48 h and then roasted; T10: inoculated and soaked maize with 300 ml of cold water for 12 h and then roasted.
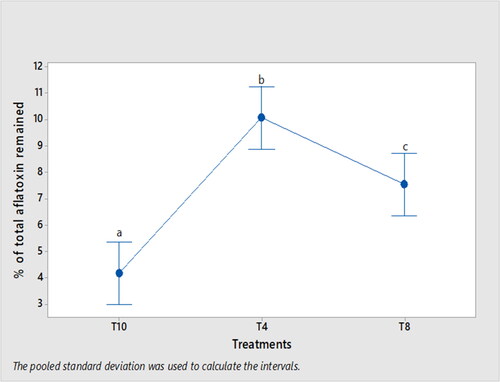
3.3. Effect of soaking water temperature
Soaking dry maize grain in 300 ml of cold water before maize boiling significantly decreased (p < .05) the total aflatoxin content by 88.6% and 89.9% compared to the aflatoxin content of soaked maize in 300 ml of warm water and 200 ml of cold water, respectively. For optimum growth of A. flavus, a temperature of 30 °C is required (Samapundo et al., Citation2007), but A. flavus growth rate is retarded at a temperature ≤20 °C (Liu et al., Citation2017). Thus, the increased soaking water temperature in warm water could favor higher A. flavus growth and subsequent aflatoxin production than the lower soaking water temperature of the cold water used for this experiment in the study area. Wanjiru et al. (Citation2020) reported that both cold water and warm water (50 to 60 °C) reduced the aflatoxin content of naturally contaminated peanuts as soaking minutes increased with a 2 min interval through agitation of soaked peanuts in 250 ml of water for a total of 8 min, but with 57.6% aflatoxin reduction in warm water soaking after 6 min of duration compared to cold water soaking.
shows that increasing the soaking period to 48 h, increasing the soaking water volume to 300 ml and using cold water during nifro making had shown significant impact in reducing the total aflatoxin content.
3.4. Effect of cooking method
This study found that roasting of soaked maize grain resulted in a considerably lower aflatoxin content compared to boiling of soaked maize grain ( and ). Boiling and roasting processes reduce 50 to 70% of aflatoxin in foods (Jalili, Citation2016). Meanwhile, Sipos et al. (Citation2021) found that high-moisture cooking and roasting reduced aflatoxin levels by 51 to 85%. Adegoke et al. (Citation1994) observed 39% reduction in the aflatoxin content of cereal-based foods after steam cooking and boiling. The higher the moisture content of the food, the higher the heat inactivation of aflatoxin by opening the lactone ring of aflatoxin and heat induced decarboxylation of the terminal carboxylic acid (Fandohan et al., Citation2005; Jalili, Citation2016; Kabak, Citation2009; Rustom, Citation1997). Boiling the naturally contaminated maize had destroyed 28% of aflatoxin B1 (Bullerman & Bianchini, Citation2014; Kabak, Citation2009; Leslie & Logrieco, Citation2014; Mutungi, Citation2006; Ryu et al., Citation2008; Scott, Citation1984; Stoloff & Trucksess, Citation1981). Boiling maize and peanut in water resulted in a moderate reduction of aflatoxins (Diedhiou et al., Citation2012; Njapau et al., Citation1998) and slightly more than moderate removal of aflatoxins (Jalili, Citation2016; Méndez-Albores et al., Citation2004). In polished rice, Aflatoxin B1 was reduced by 34% during the boiling process (Leslie & Logrieco, Citation2014; Ryu et al., Citation2008). Park and Kim (Citation2006) stated that 31 to 36% of aflatoxin B1 is lost by ordinary cooking and 78 to 88% through pressure cooking of rice. Besides, boiling aflatoxin contaminated macaroni and noodles in water for 10 min removed 29% aflatoxin with the drained water (Scott, Citation1984; Stoloff et al., Citation1978), but Kamimura (Citation1999) reported only 10 to 12% aflatoxin removal from boiled noodles migrating to the boiling water.
Roasting is a drying process which significantly reduces the aflatoxin content of foods through high temperature dry heating (Bullerman & Bianchini, Citation2014; Farahmandfar & Tirgarian, Citation2020; Kaushik, Citation2015; Leslie & Logrieco, Citation2014; Méndez-Albores et al., Citation2004; Yazdanpanah et al., Citation2005), which agrees with this study results. The increased heat during roasting enhances degradation of aflatoxins via chemical reactions between the toxins and chemical compounds in foods, which ultimately changes the structure of aflatoxins and possible thermolability of aflatoxins (Farahmandfar & Tirgarian, Citation2020). Aflatoxin B1 was destroyed by roasting maize at temperatures ranging from 145 to 165 °C (Mutungi, Citation2006; Scott, Citation1984; Yazdanpanah et al., Citation2005) and Kabak (Citation2009) observed 45 to 83% aflatoxin reduction after peanut dry roasting. Meanwhile, Arzandeh and Jinap (Citation2011) found 78% aflatoxin B1 reduction in artificially contaminated peanuts after roasting at 150 °C. Pistachio nut roasting destroyed 17 to 63% of aflatoxin while heating at 90 to 150 °C for 30 to 120 min; coffee bean roasting removed 42 to 56% of aflatoxin depending on heat penetration and heating temperature (Bullerman & Bianchini, Citation2014; Kabak, Citation2009; Leslie & Logrieco, Citation2014; Yazdanpanah et al., Citation2005). Roasting naturally contaminated peanuts at 150 °C for 30 min reduced aflatoxin B1 content by 30 to 45%, whereas roasting artificially contaminated peanuts reduced aflatoxin B1 content by 48 to 61% (Kabak, Citation2009; Pluyer et al., Citation1987). Meanwhile, Andrew et al. (Citation2011) reported 51% reduction in total aflatoxin content and Diedhiou et al. (Citation2012) described 64% aflatoxin destruction while roasting peanuts at 140 °C for 60 min. Ogunsanwo et al. (Citation2004) found 70% aflatoxin B1 reduction after roasting peanuts at 150 °C for 30 min. Roasting peanuts at 150 °C for 40 min reduced aflatoxin B1 by 38% (Yazdanpanah et al., Citation2005). Farahmandfar and Tirgarian (Citation2020) reported a reduction of 37 to 40% in aflatoxin B1, B2, G1 and G2 during roasting at 140 °C for 40 min. Dry and oil roasting of peanuts reduced 52 to 100% aflatoxin B1 (Scott, Citation1984). Hwang and Lee (Citation2006) found 60% reduction in aflatoxin B1 content of heated wheat at 150 °C for 30 min. The rate of aflatoxin degradation increases as the initial concentration of aflatoxin increases (Farahmandfar & Tirgarian, Citation2020; Martins et al., Citation2017). During food processing, factors such as heating temperature, time, moisture content, initial concentration, aflatoxin type, food matrix, ionic strength and pH affect the rate of aflatoxin reduction in foods (Jalili, Citation2016; Kabak, Citation2009; Rustom, Citation1997).
Aflatoxin decontamination and detoxification methods are categorized into physical (sorting out contaminated produce during cleaning, heating with higher moisture content, treating food with microwave, irradiation, electrolyzed water treatment and use of pulsed light), chemical (such as ammonia, citric acid and ozone) and biological (including the use of yeast and lactic acid bacteria) methods (Yang, Citation2019). In the hot and humid climate of Sub-Saharan Africa, assessing the traditional method of food processing in relation to its impact on the health of society and using the research outputs to improve knowledge, attitude and practice renders a complementary option to other aflatoxin control methods; meanwhile, the availability of A. flavus contamination-resistant maize varieties will have an immense role in controlling aflatoxin. Foremost, preventing aflatoxin-contaminated produce from entering the supply chain through a rapid, low-cost aflatoxin testing mechanism from the farm to food processing factories ensures food safety and improves livelihood.
4. Conclusion
Completely eliminating aflatoxin from the food chain is challenging, though lowering it to a safe level is possible through good agricultural practices, disease control, processing and storage facilities. Preventing aflatoxin entry into the food supply chain is the ultimate solution to mitigating the adverse effects of aflatoxin on health, food security and trade. Using chemicals to remove aflatoxin in foods may affect consumer health and deteriorate the organoleptic characteristics of treated foods. Hence, a safe, cost-effective and practical method is sought to remove aflatoxin from foods. Thus, aflatoxin detoxification through food processing serves as a complementary measure in the efforts to reduce aflatoxin below the maximum permissible limit. Food processing methods mitigate the aflatoxin content of foods while maintaining the nutritional content and sensory acceptability of foods. Consuming roasted dry maize grain (qollo) considerably reduces aflatoxin exposure. In the meantime, dry maize grain soaked in excess water and cold water before cooking showed a better reduction of aflatoxin. Integrating effective and efficient traditional food processing techniques with aflatoxin mitigation strategies ensures food safety.
Acknowledgments
The authors thank Ethiopian Institute of Agricultural Research (EIAR) for the financial support to this research. The authors would also like to thank survey study participants, Holeta Agricultural Research Center, Holeta, Ethiopia, for the provision of research laboratory, as well as Ethiopian Conformity Assessment Enterprise laboratory, Addis Ababa, Ethiopia, for the total aflatoxin content analysis.
Disclosure statement
No potential conflict of interest was reported by the author(s).
Data availability statement
Data will be made available on request.
Additional information
Notes on contributors
Habtamu Gebremichael Daba
Habtamu Gebremichael Daba, is a researcher at the Ethiopian Institute of Agricultural Research in the Food Science and Nutrition research sector, based at Kulumsa Agricultural Research Center, Asella, Ethiopia.
Mulugeta Admasu Delele
Mulugeta Admasu Delele (currently an Associate Professor), Solomon Workneh Fanta (Professor), Nigus Gabbiye Habtu (Professor), and Metadel Kassahun Abera (currently an Associate Professor) work at Bahir Dar University, Institute of Technology, Bahir Dar, Ethiopia, and have immense experience in research and teaching at the faculty of chemical and food engineering.
References
- Adegoke, G. O., Otumu, E. J., & Akanni, A. O. (1994). Influence of grain quality, heat, and processing time on the reduction of aflatoxin B1 levels in ‘tuwo’ and ‘ogi’: Two cereal-based products. Plant Foods for Human Nutrition, 45(2), 1–13. https://doi.org/10.1007/BF01088468
- Adisa, A. M., & Enujiugha, V. N. (2020). Microbiology and safety of ogi fermentation: A review. European Journal of Nutrition & Food Safety, 12(5), 90–100. https://doi.org/10.9734/ejnfs/2020/v12i530231
- Amare, M. G., & Keller, N. P. (2014). Molecular mechanisms of Aspergillus flavus secondary metabolism and development. Fungal Genetics and Biology: FG & B, 66, 11–18. https://doi.org/10.1016/j.fgb.2014.02.008
- Andrew, H. S., Kudzayishe, J. M., & Nozipo, N. (2011). Aflatoxin carryover during large scale peanut butter production. Food and Nutrition Sciences.
- Arzandeh, S., & Jinap, S. (2011). Effect of initial aflatoxin concentration, heating time and roasting temperature on aflatoxin reduction in contaminated peanuts and process optimisation using response surface modelling. International Journal of Food Science & Technology, 46(3), 485–491. https://doi.org/10.1111/j.1365-2621.2010.02514.x
- Assaye, M. A., Gemeda, N., & Weledesemayat, G. T. (2016). Aspergillus species and aflatoxin contamination of pre and post-harvest maize grain in west Gojam, Ethiopia. Journals of Food Science and Nutrition, 2, 13–18.
- Association of Official Analytical Chemists. (2006). Natural toxins. Official methods of analysis. Current through Revision (Vol. 1, 18th ed.). AOAC International.
- Assohoun, M. C., Djeni, T. N., Koussémon-Camara, M., & Brou, K. (2013). Effect of fermentation process on nutritional composition and aflatoxins concentration of doklu, a fermented maize based food. Food and Nutrition Sciences, 04(11), 1120–1127. https://doi.org/10.4236/fns.2013.411146
- Atanda, O. O., & Olopade, T. A. (2013). Effect of lemon grass (Cymbopogon citratus (DC.) Stapf.) treatments on Aspergillus flavus (SGS-421) infestation and aflatoxin B1 content of maize grains. International Food Research Journal, 20(4), 1933–1939.
- Ayele, M., Haile, D., Alonso, S., Sime, H., Abera, A., Balcha, K. H., Roba, K. T., Guma, G. T., & Endris, B. S. (2022). Aflatoxin exposure among children of age 12–59 Months in Butajira District, South-Central Ethiopia: A community based cross-sectional study. BMC Pediatrics, 22(1), 326. https://doi.org/10.1186/s12887-022-03389-w
- Ayelign, A., Woldegiorgis, A. Z., Adish, A., De Boevre, M., Heyndrickx, E., & De Saeger, S. (2017). Assessment of aflatoxin exposure among young children in Ethiopia using urinary biomarkers. Food Additives & Contaminants. Part A, Chemistry, Analysis, Control, Exposure & Risk Assessment, 34(9), 1606–1616. https://doi.org/10.1080/19440049.2017.1350290
- Besrat, A., & Gebre, P. (1981). A preliminary study on the aflatoxin content of selected Ethiopian foods. Ethiopian Medical Journal, 19(2), 47–52.
- Beyene, A. A., Woldegiorgis, A. Z., Adish, A. A., De Saeger, S., & Tolossa, A. L. (2016). Assessment of mothers’ knowledge and practice towards aflatoxin contamination in complementary foods in Ethiopia: From pre-harvest to household. World Mycotoxin Journal, 9(4), 535–544. https://doi.org/10.3920/WMJ2016.2088
- Boshe, B., Gebremedhin, S., Alemayehu, F., Eshete, M., Taye, M., & Stoecker, B. J. (2020). Aflatoxin exposure among lactating women in southern Ethiopia. Food Science & Nutrition, 8(12), 6738–6745. https://doi.org/10.1002/fsn3.1968
- Bullerman, L. B., & Bianchini, A. (2014). Good food-processing techniques: Stability of mycotoxins in processed maize-based foods. In: J. F. Leslie & A. F. Logrieco (Eds.), Mycotoxin Reduction in Grain Chains (pp. 89–100). Ames, Iowa, USA: Wiley Blackwell.
- Chandrasekharan, N. S., Kumar, B. R., Kesavan, N., Kalaigandhi, V., & Menon, K. R. K. (2014). Detection of aflatoxin production by fungi in spice samples using HPLC and direct visual cultural methods. Innovative Romanian Food Biotechnology, 14, 1–12.
- Chang, P. K., Hua, S. S. T., Sarreal, S. B. L., & Li, R. W. (2015). Suppression of aflatoxin biosynthesis in Aspergillus flavus by 2-phenylethanol is associated with stimulated growth and decreased degradation of branched-chain amino acids. Toxins, 7(10), 3887–3902. https://doi.org/10.3390/toxins7103887
- Diedhiou, P. M., Ba, F., Kane, A., & Mbaye, N. (2012). Effect of different cooking methods on aflatoxin fate in peanut products. African Journal of Food Science and Technology, 3(12), 53–58.
- Ekpa, O., Palacios-Rojas, N., Kruseman, G., Fogliano, V., & Linnemann, A. R. (2018). Sub-Saharan African maize-based foods: Technological perspectives to increase the food and nutrition security impacts of maize breeding programmes. Global Food Security, 17, 48–56. https://doi.org/10.1016/j.gfs.2018.03.007
- Ekpa, O., Palacios-Rojas, N., Kruseman, G., Fogliano, V., & Linnemann, A. R. (2019). Sub-Saharan African maize-based foods-processing practices, challenges and opportunities. Food Reviews International, 35(7), 609–639. https://doi.org/10.1080/87559129.2019.1588290
- Fandohan, P., Zoumenou, D., Hounhouigan, D. J., Marasas, W. F. O., Wingfield, M. J., & Hell, K. (2005). Fate of aflatoxins and fumonisins during the processing of maize into food products in Benin. International Journal of Food Microbiology, 98(3), 249–259. https://doi.org/10.1016/j.ijfoodmicro.2004.07.007
- Farahmandfar, R., & Tirgarian, B. (2020). Degradation of aflatoxins and tocopherols in peanut (Arachis hypogaea): Effect of aflatoxin type, time and temperature of roasting. Drying Technology, 38(16), 2182–2189. https://doi.org/10.1080/07373937.2019.1687513
- Fardiaz, S. (1997). Mycotoxin contamination of grains–A review of research in Indonesia. In E. Highley & G. I. Johnson (Eds.), Proc. of the 17th ASEAN Technical Seminar on Grain Post-Harvest Technology in Lumut, Perak Malaysia AGPP, Bangkok (pp. 112–119). ACIAR Technical Reports Series.
- Fasiha, R., Basappa, S. C., & Murthy, V. S. (1979). Destruction of aflatoxin in rice by different cooking methods. Journal of Food Science and Technology, India, 16(3), 111–112.
- Fufa, H., Akalu, G., Wondimu, A., Taffesse, S., Gebre, T., Schlosser, K., Noetzold, H., & Henle, T. (2003). Assessment of protein nutritional quality and effects of traditional processes: A comparison between Ethiopian quality protein maize and five Ethiopian adapted normal maize cultivars. Die Nahrung, 47(4), 269–273. https://doi.org/10.1002/food.200390063
- Galano, T., Fininsa, C., & Bultosa, G. (2008). Effects of net blotch (Pyrenophora teres) on malt barley yield and grain quality at Holeta. Central Ethiopia. East African Journal of Science, 2(2), 150–158.
- Gebreegziabher, T., Dean, M., Elias, E., Tsegaye, W., & Stoecker, B. J. (2022). Urinary aflatoxin M1 concentration and its determinants in school-age children in Southern Ethiopia. Nutrients, 14(13), 2580. https://doi.org/10.3390/nu14132580
- Gebremedhin, E. Z., Ararso, A. B., Borana, B. M., Kelbesa, K. A., Tadese, N. D., Marami, L. M., & Sarba, E. J. (2022). Isolation and identification of Staphylococcus aureus from milk and milk products, associated factors for contamination, and their antibiogram in Holeta, Central Ethiopia. Veterinary Medicine International, 2022, 6544705–6544713. https://doi.org/10.1155/2022/6544705
- Geyid, A., & Maru, A. (1987). A survey of aflatoxin contents in maize, sorghum. Ethiopian Journal of Health Development, 2(1), 59–70.
- Hwang, J. H., & Lee, K. G. (2006). Reduction of aflatoxin B1 contamination in wheat by various cooking treatments. Food Chemistry, 98(1), 71–75. https://doi.org/10.1016/j.foodchem.2005.04.038
- Jalili, M. (2016). A review on aflatoxins reduction in food. Iranian Journal of Health, Safety and Environment, 3(1), 445–459.
- Kabak, B. (2009). The fate of mycotoxins during thermal food processing. Journal of the Science of Food and Agriculture, 89(4), 549–554. https://doi.org/10.1002/jsfa.3491
- Kamimura, H. (1999). Removal of mycotoxins during food processing. Mycotoxins, 1999(Suppl2), 88–94. https://doi.org/10.2520/myco1975.1999.Suppl2_88
- Karlovsky, P., Suman, M., Berthiller, F., De Meester, J., Eisenbrand, G., Perrin, I., Oswald, I. P., Speijers, G., Chiodini, A., Recker, T., & Dussort, P. (2016). Impact of food processing and detoxification treatments on mycotoxin contamination. Mycotoxin Research, 32(4), 179–205. https://doi.org/10.1007/s12550-016-0257-7
- Kaushik, G. (2015). Effect of processing on mycotoxin content in grains. Critical Reviews in Food Science and Nutrition, 55(12), 1672–1683. https://doi.org/10.1080/10408398.2012.701254
- Klich, M. A. (2002). Identification of common Aspergillus species. Centralbureau voor Shimmelcultures.
- Kortei, N. K., Annan, T., Akonor, P. T., Richard, S. A., Annan, H. A., Kyei-Baffour, V., Akuamoa, F., Akpaloo, P. G., & Esua-Amoafo, P. (2021). The occurrence of aflatoxins and human health risk estimations in randomly obtained maize from some markets in Ghana. Scientific Reports, 11(1), 4295. https://doi.org/10.1038/s41598-021-83751-7
- Leslie, J. F., & Logrieco, A. (Eds.). (2014). Mycotoxin reduction in grain chains. John Wiley & Sons.
- Liu, X., Guan, X., Xing, F., Lv, C., Dai, X., & Liu, Y. (2017). Effect of water activity and temperature on the growth of Aspergillus flavus, the expression of aflatoxin biosynthetic genes and aflatoxin production in shelled peanuts. Food Control, 82, 325–332. https://doi.org/10.1016/j.foodcont.2017.07.012
- Mamo, K., Shewarega, Y., & Melese, A. (2023). Determination of levels of aflatoxin in maize produced from Shebelle Zone, Somali Regional State, Eastern Ethiopia using UHPLC. Chemical Sciences Journal, 14(1), 1–6.
- Mann, G. E., Codifer, L. P., & Dollear, F. G. (1967). Effect of heat on aflatoxins in oilseed meals. Journal of Agricultural and Food Chemistry, 15(6), 1090–1092. https://doi.org/10.1021/jf60154a013
- Martins, L. M., Sant’Ana, A. S., Iamanaka, B. T., Berto, M. I., Pitt, J. I., & Taniwaki, M. H. (2017). Kinetics of aflatoxin degradation during peanut roasting. Food Research International, 97, 178–183. https://doi.org/10.1016/j.foodres.2017.03.052
- Matumba, L., Monjerezi, M., Chirwa, E., Lakudzala, D., & Mumba, P. (2009). Natural occurrence of AFB1 in maize and effect of traditional maize flour production on AFB1 reduction, in Malawi. African Journal of Food Science, 3(12), 413–425.
- Medina, A., Rodriguez, A., & Magan, N. (2014). Effect of climate change on Aspergillus flavus and aflatoxin B1 production. Frontiers in Microbiology, 5, 348. https://doi.org/10.3389/fmicb.2014.00348
- Méndez-Albores, A., De Jesús-Flores, F., Castañeda-Roldan, E., Arámbula-Villa, G., & Moreno-Martínez, E. (2004). The effect of toasting and boiling on the fate of B-aflatoxins during pinole preparation. Journal of Food Engineering, 65(4), 585–589. https://doi.org/10.1016/j.jfoodeng.2004.02.024
- Mir, S. A., Dar, B. N., Shah, M. A., Sofi, S. A., Hamdani, A. M., Oliveira, C. A. F., Hashemi Moosavi, M., Mousavi Khaneghah, A., & Sant’Ana, A. S. (2021). Application of new technologies in decontamination of mycotoxins in cereal grains: Challenges, and perspectives. Food and Chemical Toxicology: An International Journal Published for the British Industrial Biological Research Association, 148, 111976. https://doi.org/10.1016/j.fct.2021.111976
- Mohammed, A., Seid, A., Terefe, H., Cervini, C., & Verheecke-Vaessen, C. (2022). Harvest and post-harvest handling practices associated with fumonisin B1 contamination in maize (Zea mays L.): Dietary exposure and risk characterization in eastern Ethiopia. Mycotoxin Research, 38(4), 275–287. https://doi.org/10.1007/s12550-022-00468-w
- Mutungi, C. M. (2006). [Effects of dehulling maize grains and treatment with chemical additives on the levels of aflatoxins during muthokoi making and preparation] [Doctoral dissertation]. University of Nairobi.
- Njapau, H., Muzungaile, E. M., & Changa, R. C. (1998). The effect of village processing techniques on the content of aflatoxins in corn and peanuts in Zambia. Journal of the Science of Food and Agriculture, 76(3), 450–456. https://doi.org/10.1002/(SICI)1097-0010(199803)76:3<450::AID-JSFA970>3.0.CO;2-L
- Ogunsanwo, B. M., Faboya, O. O. P., Idowu, O. R., Lawal, O. S., & Bankole, S. A. (2004). Effect of roasting on the aflatoxin contents of Nigerian peanut seeds. African Journal of Biotechnology, 3(9), 451–455. https://doi.org/10.5897/AJB2004.000-2096
- Park, J. W., & Kim, Y. B. (2006). Effect of pressure cooking on aflatoxin B1 in rice. Journal of Agricultural and Food Chemistry, 54(6), 2431–2435. https://doi.org/10.1021/jf053007e
- Pérez-Flores, G. C., Moreno-Martínez, E., & Méndez-Albores, A. (2011). Effect of microwave heating during alkaline-cooking of aflatoxin contaminated maize. Journal of Food Science, 76(2), 48–52. https://doi.org/10.1111/j.1750-3841.2010.01980.x
- Pluyer, H. R., Ahmed, E. M., & Wei, C. I. (1987). Destruction of aflatoxins on peanuts by oven-and microwave-roasting. Journal of Food Protection, 50(6), 504–508. https://doi.org/10.4315/0362-028X-50.6.504
- Ranum, P., Peña-Rosas, J. P., & Garcia-Casal, M. N. (2014). Global maize production, utilization, and consumption. Annals of the New York Academy of Sciences, 1312(1), 105–112. https://doi.org/10.1111/nyas.12396
- Reddy, K. R. N., Raghavender, C. R., Salleh, B., Reddy, C. S., & Reddy, B. N. (2011). Potential of aflatoxin B1 production by Aspergillus flavus strains on commercially important food grains. International Journal of Food Science & Technology, 46(1), 161–165. https://doi.org/10.1111/j.1365-2621.2010.02468.x
- Roby, M. H., & Samah, A. (2018). Aflatoxin contamination, phenolic contents concentration in tigernuts as affected by traditional household processes. Egyptian Journal of Food Science, 46, 189–196.
- Rustom, I. Y. (1997). Aflatoxin in food and feed: Occurrence, legislation and inactivation by physical methods. Food Chemistry, 59(1), 57–67. https://doi.org/10.1016/S0308-8146(96)00096-9
- Ryu, D., Bianchini, A., & Bullerman, L. B. (2008). Effects of processing on mycotoxins. Stewart Postharvest Review, 4(6), 1–7.
- Samapundo, S., Devlieghere, F., Geeraerd, A. H., De Meulenaer, B., Van Impe, J. F., & Debevere, J. (2007). Modelling of the individual and combined effects of water activity and temperature on the radial growth of Aspergillus flavus and A. parasiticus on corn. Food Microbiology, 24(5), 517–529. https://doi.org/10.1016/j.fm.2006.07.021
- Scott, P. M. (1984). Effects of food processing on mycotoxins. Journal of Food Protection, 47(6), 489–499. https://doi.org/10.4315/0362-028X-47.6.489
- Shekhar, M., Singh, N., Dutta, R., Kumar, S., & Mahajan, V. (2017). Comparative study of qualitative and quantitative methods to determine toxicity level of Aspergillus flavus isolates in maize. PLoS One, 12(12), e0189760. https://doi.org/10.1371/journal.pone.0189760
- Sipos, P., Peles, F., Brassó, D. L., Béri, B., Pusztahelyi, T., Pócsi, I., & Győri, Z. (2021). Physical and chemical methods for reduction in aflatoxin content of feed and food. Toxins, 13(3), 204. https://doi.org/10.3390/toxins13030204
- Stoloff, L., Trucksess, M., Anderson, P. W., Glabe, E. F., & Aldridge, J. G. (1978). Determination of the potential for mycotoxin contamination of pasta products. Journal of Food Science, 43(1), 228–230. https://doi.org/10.1111/j.1365-2621.1978.tb09778.x
- Stoloff, L., & Trucksess, M. W. (1981). Effect of boiling, frying, and baking on recovery of aflatoxin from naturally contaminated corn grits or cornmeal. Journal - Association of Official Analytical Chemists, 64(3), 678–680.
- Sultana, B., Naseer, R., & Nigam, P. (2015). Utilization of agro-wastes to inhibit aflatoxins synthesis by Aspergillus parasiticus: A biotreatment of three cereals for safe long-term storage. Bioresource Technology, 197, 443–450. https://doi.org/10.1016/j.biortech.2015.08.113
- Torres, P., Guzmán-Ortiz, M., & Ramírez-Wong, B. (2001). Revising the role of pH and thermal treatments in aflatoxin content reduction during the tortilla and deep frying processes. Journal of Agricultural and Food Chemistry, 49(6), 2825–2829. https://doi.org/10.1021/jf0007030
- Uka, V., Cary, J. W., Lebar, M. D., Puel, O., De Saeger, S., & Diana Di Mavungu, J. (2020). Chemical repertoire and biosynthetic machinery of the Aspergillus flavus secondary metabolome: A review. Comprehensive Reviews in Food Science and Food Safety, 19(6), 2797–2842. https://doi.org/10.1111/1541-4337.12638
- U.S. Food and Drug Administration. (2002). Investigative operations manual.
- Wanjiru, J. W., Imungi, J. K., & Njue, L. G. (2020). Moisture and aflatoxin contents of Kenyan market peanuts and decontamination with water, lime and ultraviolet radiation treatments. Asian Food Science Journal, 17(1), 48–57. https://doi.org/10.9734/afsj/2020/v17i130185
- Worku, A. F., Abera, M., Kalsa, K. K., Subramanyam, B., & Habtu, N. G. (2019). Occurrence of mycotoxins in stored maize in Ethiopia. Ethiopian Journal of Agricultural Sciences, 29(2), 31–43.
- Yahl, K. R., Watson, S. A., Smith, R. J., & Barabolok, R. (1971). Laboratory wet-milling of corn containing high levels of aflatoxin and a survey of commercial wet-milling products. Cereal Chemistry, 48(4), 385–391.
- Yang, Q. (2019). Decontamination of aflatoxin B1. In X. D. Long (Ed.), Aflatoxin B1 occurrence, detection and toxicological effects (pp. 177–190). London, UK: InTechOpen.
- Yazdanpanah, H., Mohammadi, T., Abouhossain, G., & Cheraghali, A. M. (2005). Effect of roasting on degradation of aflatoxins in contaminated pistachio nuts. Food and Chemical Toxicology: An International Journal Published for the British Industrial Biological Research Association, 43(7), 1135–1139. https://doi.org/10.1016/j.fct.2005.03.004
- Yilma, S., Sadessa, K., & Kebede, D. (2019). Fungal infections and aflatoxin contamination in maize grains collected from west showa and east wallega zones, Ethiopia. International Journal of Current Research and Review, 11(21), 16–22. https://doi.org/10.31782/IJCRR.2019.11213
- Yu, J., Fedorova, N. D., Montalbano, B. G., Bhatnagar, D., Cleveland, T. E., Bennett, J. W., & Nierman, W. C. (2011). Tight control of mycotoxin biosynthesis gene expression in Aspergillus flavus by temperature as revealed by RNA-Seq. FEMS Microbiology Letters, 322(2), 145–149. https://doi.org/10.1111/j.1574-6968.2011.02345.x