ABSTRACT
Global urbanisation has occurred in tandem with population ageing, having implications on human cognitive health. Urban environmental factors such as air pollution are known risk factors of cognitive impairment and neurodegenerative disease. However, due to the sparse evidence base, the biological pathways by which urban environmental factors operate are not well understood. The aim of this review is to explain how exploring the epigenome (i.e. chemical modifications to the genome which do not change the underlying gene sequence) can further our understanding of these biological pathways. The epigenome is influenced by environmental factors and has implications for cognitive impairment and neurodegenerative disease. Utilising complex epigenetic analytical techniques including epigenetic clocks, Mendelian randomization and multi-omic approaches, it is possible to identify environmental consequences on underlying biology. Through better understanding of how epigenetic modifications, which can be inherited or change dynamically in response to environmental exposures, impact cognitive outcomes, we can work to encourage the development of public health policies, as well as urban planning and design policies to reduce the burden of neurodegenerative disease and encourage healthier ageing in the older adult population.
Introduction
Urbanisation and neurodegenerative disease
In 2021, 55% of the global population lived in urban areas (World Health Organisation, Citation2021), a substantial change from the 1960s when 34% of the global population was urban living (United Nations Population Division, Citation2019). Urban environmental factors (physical, natural and built aspects of towns and inner cities including environmental by-products) can be harmful to human health and contribute to disease outcomes (Flies et al. Citation2019, Fuller et al. Citation2022). However, there are urban environment factors which benefit environmental health and human health, such as provision of high-quality urban green and blue space, and high neighbourhood walkability. Supporting the health of a growing urban population requires a healthy urban environment.
In tandem, our global population is ageing, with 2.1 billion people predicted to be 60 years of age or older by 2050 (World Health Organisation, Citation2021). Cellular impairment and disease risk increases with age (Hui Citation2017, Dominguez et al. Citation2021, Liu Citation2022). Neurodegenerative diseases (e.g. Alzheimer’s disease, Parkinson’s disease) are a type of non-communicable disease of the central nervous system which come with a high likelihood of premature mortality from neurodegenerative progression (White et al. Citation2020). Due to the lack of regenerative capacity of the central nervous system (Cooke et al. Citation2022), strategies to identify and develop preventative mechanisms are required. In 2022, over 55 million older adults were living with dementia globally, projected to double to 139 million persons by 2050 (Nichols Citation2022). The longer life expectancy of populations coupled with increasing risk of disease and ill health requires strategies to help populations age well with minimal health complications. Furthermore, the economic burden of disease has also become increasingly evident. In the UK, the total cost of dementia care per year is 34.7 billion pounds (Wittenberg et al. Citation2020). This highlights a research necessity to improve the ageing experience of the population, including reducing neurodegenerative risk.
There are numerous identified risk factors for neurodegenerative diseases. Age is believed to be the largest contributor (Hou et al. Citation2019, Ju and Tam Citation2022) to neurodegenerative risk. The global ageing of the population is therefore a concern and highlights the urgency for preventive population-level interventions. Other known risk factors include sex and vascular disease (Mielke et al. Citation2014, Sumien et al. Citation2021) which can arise from atherosclerotic pathology. Socioeconomic risk factors include being from a lower income household (Wang et al. Citation2021) and having lower educational attainment (Takasugi et al. Citation2021). In addition, environmental exposures, including heavy metals, pesticides (Chin-Chan et al. Citation2015, Tang Citation2020) and air pollutants (Wu et al. Citation2022), are also known risk factors of neurodegenerative disease. Other urban environment factors such as noise (Ho et al. Citation2012) and light (Baranyi et al. Citation2022) pollution have been associated with higher risk of cognitive impairment. Conversely, urban green and blue space presence has been evidenced to be protective against cognitive impairment (Zhang et al. Citation2022b). However, the biological pathways by which urban environment factors influence cognitive impairment and neurodegenerative risk are not fully understood (Heusinkveld et al. Citation2016, Chandra et al. Citation2022, Meng et al. Citation2022).
An attractive risk factor for neurodegenerative disease is epigenetic modification (Millan Citation2014, Zhang et al. Citation2022a). The epigenome is the term used to describe chemical modifications to the genome which do not change the underlying gene sequence (Morgensztern et al. Citation2018). These modifications can alter the expression of genes, resulting in a biological cascade which could either contribute to disease risk, or protect from disease (Ho et al. Citation2012). The epigenome is modifiable by environmental exposures, with urban environment factors such as air pollution being associated with epigenetic modifications (Baranyi et al. Citation2022, Holliday et al. Citation2022). This modification may contribute to the biological risk of neurodegenerative disease and is thereby a potential mechanism by which environmental exposures influence disease risk (Jirtle and Skinner Citation2007, Wu et al. Citation2023). This makes multi-omic exposomic research on urban environment and neurodegenerative disease of interest, including investigation into the consequences of exposures at both the genetic and epigenetic level.
Exposomic research
Recently, the exposome – endogenous and exogenous factors to which the body is exposed in the lifetime (Kalia et al. Citation2022) – is being explored to identify its role in age-associated conditions such as neurodegenerative disease. It has been hypothesised that epigenetic modifications could be used as a proxy to explore the exposome (Colwell et al. Citation2023), as it is possible to analyse and visualise epigenetic modifications which are the result of environmental exposures. By investigating epigenetic modifications in urban environmental-neurodegenerative research, it is possible to explore biological pathways by which urban environment factors influence neurodegenerative disease induction and progression.
Focusing on exogenous environmental risk factors for disease is beneficial considering the ability to modify exposure to them and the potential impact at a population level. Implementation of urban health policies have the potential to reduce exposure to harmful pollutants whilst encourage exposure to urban green and blue spaces which enhance both environmental and human health (Wilson and Xiao Citation2023). These policies would be a potential therapeutic, reducing the risk of neurodegenerative disease (Besser Citation2021).
Aim of review
This review aims to summarise the evidence on the influence of key urban environment factors which are known to affect human cognitive function and highlight how they influence the biological risk of neurodegenerative disease.
The urban environment and neurodegenerative risk
The next section synthesises the evidence for environmental factors.
Air pollution
It is estimated that 91% of urban residents live within polluted air (World Health Organisation Citation2021), in an area not conforming to the global air quality guidelines provided by the WHO (World Health Organisation Citation2021). Vehicle emissions are considered the largest contributor to poor urban air quality (Kim et al. Citation2015). The most common type of air pollution included in biological research is particulate matter (PM). PM is composed of inhalable pollutants such as nitrates, sulfates, endotoxins, carbon and heavy metals (World Health Organisation Citation2013). PM is categorised by its size: PM10 is composed of coarse material, PM2.5 of fine and PM0.1 of ultra-fine (Benabed and Boulbair Citation2022). The size indicates the level of penetration into the respiratory tract, as PM10 will infiltrate the tracheal and bronchial mucosa (Atkinson et al. Citation2010) while PM0.1 is so small it can behave similar to a gas, diffusing at the level of the alveoli into the systemic circulation (Valavanidis et al. Citation2008). This makes PM0.1 a highly penetrative and potentially damaging aspect of air pollution.
There is research debate surrounding the efficiency with which pollutants can bypass and disrupt the blood–brain barrier to directly impact the brain. Multiple studies have provided evidence that the blood–brain barrier, a highly selective protective barrier to the brain parenchyma, is disrupted through pollutant exposure (Oppenheim et al. Citation2013, Marin et al. Citation2018). Nanosized PM deposits have been discovered in the frontal cortex of human brains (Maher et al. Citation2016). A study by Maher et al., specifically analysed the presence of magnetite in the brain (Maher et al. Citation2016), which has been linked to Alzheimer’s disease (Pankhurst et al. Citation2008). Barrier disruption, allowing pollutant infiltration into the brain, could increase the risk of an intercranial inflammatory response and the generation of reactive oxygen species. The nasal olfactory tract is a further proposed inhalation mechanism by which pollutants target the brain. Olfactory deficit and dysfunction are common early signs of neurodegenerative disease (Lafaille-Magnan et al. Citation2017), including Alzheimer’s disease (Lafaille-Magnan et al. Citation2017) and Parkinson’s disease (Morley et al. Citation2018). Further validation of these findings is required to confirm or disprove these theories and identify clear pathways by which air pollutants access and impact the brain.
The impact of air pollutants on human health has been well documented in the literature, commonly focusing on PM2.5. In a meta-analysis by Yu et al., investigating aspects of air pollution and risk of cognitive impairment, there was a significant positive correlation between PM2.5 and the risk of cognitive impairment in population-based studies (Yu et al. Citation2020). However, there was moderate observed heterogeneity (I2 68.5%) in the 10 studies included in this meta-analysis. Visually, the funnel plot indicated publication bias; however, the Egger analysis suggested no significant evidence of this bias. In a recent systematic review by Peters et al. (Citation2019), 13 population-based and cohort studies were analysed with an overall conclusion that greater exposure to air pollutants may be associated with increased risk of cognitive decline and dementia. PM2.5 was the most commonly analysed pollutant in included studies. Included within this review was a study by Chen et al. (Citation2017a), which assessed the risk of dementia against exposure to numerous air pollutants, finding varying results. Chen et al. found an increased risk of dementia associated with PM2.5 (HR 1.04 95%CI 1.03-1.05). However, no association was found between dementia incidence and ozone. In a further study by Chen et al. (Citation2017b), the residential proximity to a roadway was assessed against the risk of dementia. Using proximity to the road can be beneficial in environmental studies as it can relate to both traffic-related air pollution and traffic-related noise pollution. This study found that living within 50 m of a roadway increased the risk of dementia (HR1.07 95%CI 1.06-1.08).
Although the literature concerning air pollution is largely PM2.5 focused; other pollutants such as NO2 have been explored. NO2 exposure has been associated with an increased risk of Parkinson’s disease (Jo et al. Citation2021) and in the beforementioned Chen et al. (Citation2017a) study NO2 exposure was associated with an increased risk of dementia (HR 1.1 95%CI 1.08-1.12). Studies have also found significant associations between NO2 and amyloid deposition (Alemany et al. Citation2021), one of the most common pathological marks of Alzheimer’s disease (Duran-Aniotz et al. Citation2021). Alemany et al. also found associations between PM2.5 and amyloid deposition, as well as PM2.5 and PM10 with cerebrospinal fluid neurofilament light, a neurofilament biomarker of neurodegeneration. An interesting study by Lin et al. compared air pollution components NO2, PM10, S02, ozone, and carbon monoxide between patients with Alzheimer’s disease in two Taiwanese cities (Lin et al. Citation2022). Patients with Alzheimer’s disease living in the city with higher pollutant exposure were shown to be experiencing faster cognitive deterioration. This would suggest air pollutants may also influence the rate of neurodegenerative progression.
Overall, these studies reflect how air pollution is evidenced to impact the risk of cognitive impairment and neurodegenerative disease and is considered a risk factor for dementia (Livingston et al. Citation2020). However, there is no universal agreement on how pollutants disrupt specific biological pathways to contribute to disease pathology. Genotoxicity from oxidative stress and inflammatory induction (Calderón-Garcidueñas et al. Citation2020, Dumax-Vorzet et al. Citation2015, Lee et al. Citation2023, Pandics et al. Citation2023) are commonly agreed general mechanisms which need further experimental exploration.
Water pollution
A further concern for urban populations is usage or consumption of contaminated water supplies. Contaminated water supplies contain toxic levels of heavy metals from contaminated soil (Ahmad et al. Citation2021) or street run-off containing traffic-related pollutants (Müller et al. Citation2020). High usage of plastics has resulted in high levels of bisphenol A being present in our global water supply (Thompson et al. Citation2009). Bisphenol A is a xenoestrogen which despite having a low affinity with oestrogen receptors, has been implicated in cognitive decline from long-term exposure and consumption (Suresh et al. Citation2022). This is suspected to be due to the role of oestrogen receptors in cognitive functions such as verbal memory (Hara et al. Citation2015) being disrupted. Recently, there has been emerging evidence on the impact of water quality on the brain (Bondy and Campbell Citation2017) and cognitive impairment risk (Liu et al. Citation2017, Pan et al. Citation2022a). Bondy and Campbell (Citation2017), specifically analyse the neurotoxicity of metals such as lead and copper, two elements abundant in brake wear emissions which can infiltrate water supplies via urban street run-off (Grigoratos and Martini Citation2015).
Early life exposure to lead is suspected to be involved in genomic imprinting which impacts the expression of Alzheimer’s related genes in later life, contributing to neurodegenerative risk (Lahiri and Maloney Citation2010). A case–control study investigating blood lead levels found that levels were higher in those with Alzheimer’s disease (OR 1.05 95%CI 1.01-1.09) in comparison to controls (Fathabadi et al. Citation2018). This study did not specifically investigate sources of lead exposure but did acknowledge that accumulative lead exposure over the life course does seem to associate with higher neurodegenerative risk. The pathogenic nature of lead in terms of Alzheimer’s disease likely involves neuroinflammation (Adekomi Citation2017) and encouraging amyloid deposition (Zhou et al. Citation2018). The study did not consider co-exposure of elements on the impact of Alzheimer’s which would be desirable. In a study by Pan et al. (Citation2022b), a sample of the China Health and Retirement Longitudinal Study (CHARLS) was used to investigate the relationship between water quality and cognitive function in the older adult Chinese population. Higher water quality was associated with higher scores in mental status (relating to orientation and numerical ability), episodic memory and global cognition. This finding is consistent with a Portuguese study examining the association between heavy metals referred to as potentially toxic elements (PTE) and cognitive function (Cabral Pinto et al. Citation2018). Higher urinary PTE levels were associated with cognitive impairment. The groundwater of the study area was examined for PTE which showed elevated levels of aluminium, iron, manganese and zinc. These elements are main contributors to both water and soil pollution.
Soil pollution
Air pollution impacts not just the quality of water but also the quality of and composition of the soil. Airborne pollutants are suspended at the soil level, resulting in their penetration and infiltration into the soil. Street run-off can further relocate air and traffic-related pollutants from the urban streets to surrounding soil. The pollutant levels in the soil can also directly impact water quality, as groundwater streams become infiltrated with pollutants. Human exposure to soil pollutants can be through inhalation, ingestion or through dermal (skin) contact (Marini et al. Citation2021, Lupolt et al. Citation2022). A systematic review by Killin et al. (Citation2016) investigated the association between environmental exposures including soil and water pollutants and dementia risk. The outcomes of studies were conflicting, showcasing the necessity for further research. In Shen et al., a study included in this review, higher levels of aluminium were associated with reduced risk of dementia (comparing highest to lowest exposure RR 0.267 95%CI 0.265-0.268) (Shen et al. Citation2014). This is conflicting to a meta-analysis (2016) of studies (n = 8) which suggested that individuals chronically exposed to aluminium were 71% more likely to develop Alzheimer’s disease (Wang et al. Citation2016). No publication bias was observed amongst these eight studies and there was low heterogeneity. A more recent (2021) meta-analysis (n = 23) confirmed this association of high aluminium exposure and cognitive impairment specifically with declines in global cognition scores, working memory and processing speed (Bagepally et al. Citation2021). In this analysis, however, heterogeneity and publication bias were significant. Aluminium is a neurotoxicant which is implicated in the promotion of amyloid deposition (Zhao et al. Citation2014). This metal has been shown to alter the expression of genes involved in the formation of amyloid and neurofibrillary tangles, a further pathological mark of Alzheimer’s disease (Duran-Aniotz et al. Citation2021) as well as decreasing expression of neurotrophins (Kawahara and Kato-Negishi Citation2011, He et al. Citation2021). Heavy metals are generally believed to contribute to cognitive impairment and neurodegenerative risk through inducing oxidative stress (Poprac et al. Citation2017) and altering gene expression (Lahiri et al. Citation2009, Bakulski et al. Citation2020).
Noise pollution
With much industrial activity, road traffic and high usage of airports in large cities, the urban area is subject to noise pollution (de Paiva Vianna et al. Citation2015). In a study by Chen et al. (Citation2017b), traffic-related noise pollution was associated with increased incidence of dementia. The relationship was strongest in urban residents, especially those within major cities and this may be mediated by traffic-related air pollution. This paper by Chen et al. was included in a systematic review and meta-analysis which aimed to investigate the relationship between chronic noise exposure and the risk of dementia (Meng et al. Citation2022). The overall finding from this review was a significant positive association between the two variables, chronic noise exposure was seen to increase the risk of dementia (>57 decibels. RR 1.47 95%CI 1.21-1.78). However, heterogeneity was observed between the nine included studies in the meta-analysis (I2 = 78.7%) and publication bias analysis was absent. The low number of included studies (quantitative synthesis = 9) within the review by Meng et al. (Citation2022) highlights the limited study with regards to noise pollution and neurodegenerative risk. Despite the suspected relationship, there are few human studies in this area. Animal experimentation has suggested that chronic high-level noise exposure promotes tau hyperphosphorylation and amyloid beta overproduction (Su et al. Citation2018). Chronic noise exposure is also associated with sleep disturbance (Le et al. Citation2017) which may increase the risk of dementia. Noise has been evidence to evoke a neuronal stress response in which oxidative stress is a downstream consequence (Daiber et al. Citation2020), a large contributor to neurodegenerative pathophysiology. A study in rats by Cui et al. (Citation2012) suggests that noise induced tau phosphorylation may be partially reversible in the absence of chronic exposure, a beneficial insight for public health personnel who can encourage the incorporation of noise prevention into urban policy.
Light pollution
Light pollution, in particular outdoor light at night (OLAN), is a further issue in urban areas, as artificial light is used extensively in cities after sunset. These high levels of light can disrupt circadian rhythms, which may have numerous consequences as hormone levels, cognitive function, and several other homeostatic mechanisms, are modulated by the circadian clock (Xu et al. Citation2021). Compromised homeostasis makes the body vulnerable to disease induction (Escobar et al. Citation2011) from further exogenous or endogenous stressors. The circadian rhythm disruption itself can also contribute to multiple neurological issues such as sleep disorders (contributed to by noise pollution), depression and anxiety (Walker et al. Citation2020), which could serve as a predeterminant to future cognitive impairment and neurodegenerative disease. Sleep disorders and ageing are both associated with decreased pineal melatonin production (Hardeland Citation2012, Chen et al. Citation2022). Decreased melatonin production at night is associated with neurodegenerative diseases including Alzheimer’s (Nous et al. Citation2021), likely impacting neurodegenerative risk due to its neuroprotective properties (Alghamdi Citation2018). Pineal melatonin has been described as anti-amyloidogenic (Shukla et al. Citation2017), and has been evidenced to encourage neuroplasticity (Stefanova et al. Citation2015) and reduce oxidative stress in Alzheimer’s disease (Shukla et al. Citation2017). There is limited literature on the effect of OLAN on cognitive impairment and neurodegenerative disease risk. A recent study of veterans in China found that long-term exposure to OLAN was associated with increased risk of mild cognitive impairment (OR 1.44 95%CI 1.36-1.52), considered a transitional state before dementia (Chen et al. Citation2022). A further study in Italy found that high exposure to OLAN was associated with an increased risk of late-onset dementia (OR 3.5 95%CI 0.32-38.87) (Mazzoleni et al. Citation2023).
Urban green and blue space
Lack of good-quality natural environments including green and blue spaces can be an issue in urban areas. Urban green space includes public parks, gardens, greenways, and other types of open and recreational spaces that are present in urban areas. Similarly, blue space describes natural areas of water within the urban area, such as a river. These features serve to positively impact the health of urban populations through their perceived benefits, for example, through encouragement of physical and social activity (Beyer et al. Citation2014, Besser et al. Citation2021). Physical activity is associated with reduced risk of dementia, including Alzheimer’s disease (Iso-Markku et al. Citation2022), with one of the suggested reasons for this being that it reduces the risk of future cardiometabolic disorders associated with Alzheimer’s disease risk (Tai et al. Citation2022). Social activity can encourage neural stimulation, increasing cognitive reserve which is considered protective of Alzheimer’s disease (Liu et al. Citation2019). There are also more specific neurological benefits of being within the natural landscape, such as decreased sympathetic nerve activity (Mueller et al. Citation2022). A recent cohort study has found associations between having a ‘healthy lifestyle’ (including engaging in physical and social activity and cognitive stimulation) with reduced risk of memory decline in both APOE4 carriers and non-carriers (Jia et al. Citation2023). This study had a 10-year follow-up period, allowing memory loss to be examined over a substantial time period; however, lifestyle behaviour measurements were self-assessed, potentially decreasing reliability of study results.
Urban green and blue spaces have been suggested to decrease risk of cognitive impairment highlighted by the results of intervention studies such as Gidlow et al. (Citation2016b). In this study, walking within green and blue spaces resulted in improvements in cognitive function when compared to walking in an urban environment. Cognitive improvements were most significant after walking in blue space. Studies have also been carried out to investigate residential proximity to these green and blue spaces and cognitive measures, with these studies suggesting a reduction in the risk of cognitive impairment when urban green and blue spaces are present close to residence (Sylvers et al. Citation2022, Rodriguez-Loureiro et al. Citation2022). However, due to high levels of within-study and between-study variations, results from studies in this area have provided conflicting evidence. Wu et al. investigated the association between residential distance to an urban park and risk of dementia finding that living further from a park was associated with lower odds of dementia (OR 0.64 95%CI 0.42-0.95) (Wu et al. Citation2020). There is also a smaller amount of literature concerning blue space and its relationship with cognitive measures (White et al. Citation2020). Considering the potential for urban green and blue space to reduce the risk of cognitive impairment, issues can arise in urban areas if these spaces are of limited presence or are not seen as safe areas for people to spend time in, due to associations with crime. The accessibility of urban green and blue spaces is also important if they are to be used by all individuals in the population despite their age or disability status. In the creation of healthy cities, urban green and blue space development and maintenance will be integral for improving environmental health through mitigating temperature rises (Hasan et al. Citation2022) and pollution levels (Junior et al. Citation2022) and will also have impacts on population health including enhancing the walkability of the city (Zuniga-Teran et al. Citation2019, Zhang et al. Citation2020).
Walkability and cyclability
Neighbourhood walkability, the ability to walk to desired locations without a car, is an important characteristic of the urban area. The walkability of the urban area will depend partly on urban green spaces, as well other features such as proximity to and density of roads, the number of pedestrian walkways and crossings (Knapskog et al. Citation2019). Limited neighbourhood walkability can lead to issues in terms of physical inactivity of urban residents and increased usage of private transport, exacerbating the issue of traffic-related air pollution in urban areas. Neighbourhood walkability has been associated with improved global cognitive scores and attention psychomotor speed at baseline in a recent study (Timmermans et al. Citation2023), especially in individuals with vascular cognitive impairment. However, these associations were not found to be significant in the subsequent 2-year follow-up period. Another study investigating access to amenities through neighbourhood walkability found no significant association between walkability and cognition (Katayama et al. Citation2020). This study did find other interesting significant associations, as study participants engaging in a more active lifestyle were less likely to have mild or global cognitive impairment. Neighbourhood walkability was hypothesised to be protective of this association.
A high degree of cyclability in a city is beneficial in numerous ways including traffic-related emissions (Scorza and Fortunato Citation2021) and noise accumulation. Cycling also impacts an individual’s physical activity which is one of the modifiable risk factors for cognitive impairment and neurodegenerative disease (Iso-Markku et al. Citation2022). A study by Wittfield et al. (Citation2020) found that increased cardiorespiratory fitness (achieved through walking, cycling and running) was associated with higher grey matter volumes in specified brain regions. Grey matter atrophy is a common occurrence in neurodegenerative diseases such as Alzheimer’s due to neuronal loss (Wu et al. Citation2021). Cycling may be an activity which could be encouraged to protect from brain atrophy. However, Williams et al. found that cortical volumes were dependent on a further factor, mean diffusivity, when assessing if higher cortical volume was associated with higher or lower longitudinal memory performance (Williams et al. Citation2023). Further study is therefore required with a more complex assessment of brain volumes to identify if physical activity through cycling for example can protect from brain atrophy. Current issues in cities resulting in poor cyclability include absent or inadequate cycling infrastructure (Iwińska et al. Citation2018, Caicedo et al. Citation2021). Addressing these issues will work to improve the health of cities and potentially protect residents of the city from future cognitive impairment and neurodegenerative disease.
As urban environment factors and associated health behaviours, including physical and social activity discussed above, have been associated with cognitive impairment and neurodegenerative disease in the literature, it is of interest to explore the potential biological mediators of these associations. The epigenome is a modifiable and measurable mechanism which could provide insight to the biological pathways implicated in how urban environment factors of interest impact the risk of cognitive impairment and neurodegenerative disease.
The urban environment and epigenetic influence
The epigenome helps regulate gene expression to enable required cellular functions and to help people respond to environmental stimuli (Gibney and Nolan Citation2010). The epigenome is modified throughout the human lifespan to regulate development (Skinner Citation2011) and ageing (Wang et al. Citation2022). These modifications are commonly reversible and induced by environmental factors (Kanherkar et al. Citation2014). Epigenetic modifications include DNA methylation (DNAm), histone modification, and non-coding RNAs. DNAm commonly occurs when a methyl group is transferred to a cytosine of a CpG site of DNA (Zeng et al. Citation2022), while histones are structural proteins with roles in nucleosome remodelling and chromatin structure (Millán-Zambrano et al. Citation2022). Non-coding RNAs such as microRNAs and long-ncRNAs can also alter the epigenome through post translational gene regulation and influence the level of DNAm and histone modification (Panni et al. Citation2020).
The epigenome is modifiable through exogenous influences, including an individual’s surrounding environment. Urban environment factors have been evidenced to modify the epigenome (Skinner Citation2011, Wang et al. Citation2022), and epigenetic modifications are known to be cognitively influential and be implicated in neurodegenerative disease (Hwang et al. Citation2017, Zhang et al. Citation2022a). It is of interest to identify if a biological link exists between urban environment factors and neurodegenerative risk, via epigenetic modification. There is a small amount of research dedicated to the investigation of this area. Focusing research on this link is beneficial considering the potential for the epigenome to be modified and epigenetic modifications to potentially be reversed (Gibney and Nolan Citation2010). It is hoped that the dynamic nature of the epigenome (Cazaly et al. Citation2019) will allow us to alter exposure to influential environmental factors, to reverse epigenetic modifications which increase risk of disease or alternatively, encourage protective modifications to alleviate neurodegenerative risk. To enable beneficial alterations of environmental exposures, further depth of knowledge is required with regards to the consequences of the urban environment on the epigenome. Developing health recommendations backed up by this additional biological research would potentially encourage policymakers to take action and create interventions to enhance environmental and population health, thereby reducing the risk of neurodegenerative disease.
Identifying epigenetic modifications which occur as a result of environmental exposures, and exploring how these modifications are involved in the causation of neurodegenerative disease, requires complex analytical techniques. Epigenetic analysis mainly involves epigenetic clock analysis and epigenome-wide association studies (EWAS), additionally utilising Mendelian randomization (MR) as a technique to assess causation. Harnessing these techniques will be greatly beneficial in future research, with the aim of finding biological links between urban environment factors and disease. These techniques aid the identification and visualisation of epigenetic markers which may be implicated in causal pathways and are crucial to begin developing further biological pathway knowledge.
Epigenetic analytical techniques
Current literature has identified epigenetic markers associated with urban environment factors, helping explain the underlying biological pathways by which these environment factors influence disease risk. The majority of research in this area has focused on DNAm, with a particular focus on epigenetic clocks and EWAS.
Epigenetic clocks
Epigenetic clocks are often used to capture accelerated biological ageing, predicting risk of morbidity and premature mortality (Hannum et al. Citation2013, Levine et al. Citation2015, Citation2018, Horvath and Raj Citation2018, Bell et al. Citation2019, Noroozi et al. Citation2021). Epigenetic clocks use patterns of CpG methylation, where a methyl group is placed in the genomic region of a cytosine followed by a guanine nucleotide, to predict biological age (Li et al. Citation2022). When biological age exceeds that of an individual’s chronological age, this indicates biological age acceleration has occurred (Jain et al. Citation2022). Biological ageing is linked to cellular senescence (Yang and Sen Citation2018), a process in which cells exit the cell cycle and no longer have proliferative functions (Di Micco et al. Citation2021). High numbers of senescent cells can disrupt homeostasis and leave the body vulnerable to disease induction, a potential pathway as to how epigenetic modification influences disease risk (Crouch et al. Citation2022).
Current findings utilising epigenetic clocks
Studies have found that accelerated biological age is associated with cognitive impairment (Levine et al. Citation2015), Parkinson’s disease age of onset (Tang et al. Citation2022) and dementia (Wu et al. Citation2021). However, many additional studies have failed to find significant associations between accelerated biological age and neurodegenerative disease. A detailed review concerning studies investigating biological age acceleration and dementia has been carried out by Zhou et al. (Citation2022). Studies utilising epigenetic clocks have associated urban environment factors with biological ageing. A recent study (Yannatos et al. Citation2022) focused on identifying racial differences in DNA methylation age with socioeconomic status and air pollution exposure in a large US cohort. Black participants were exposed to greater levels of air pollution than White participants, with lower socioeconomic status and education level partially explaining the accelerated biological age observed in Black participants for Dunedin Pace of Ageing; however, it was hypothesised that air pollution, specifically PM2.5 exposure, was also a contributor. PM2.5 components have been associated with biological ageing in other studies (White et al. Citation2019, Wang et al. Citation2020, Gao et al. Citation2022), suggesting this is one of the mechanisms which mediate air pollution related ill health and potentially neurodegenerative risk.
Important considerations regarding epigenetic clocks
Multiple generations and types of epigenetic clocks have been developed. First-generation clocks Hannum and Horvath were designed to be predictors of chronological age (Hannum et al. Citation2013, Horvath Citation2013) however, second-generation clocks PhenoAge and GrimAge were trained with the inclusion of more clinical-based markers such as C-reactive protein (Lu et al. Citation2019). Second-generation clocks are considered better predictors of mortality and morbidity (Noroozi et al. Citation2021, McCrory et al. Citation2021) Third-generation clocks consider longitudinal and cross-species data (Belsky et al. Citation2020, Lu et al. Citation2023). There is substantial transcriptional overlap for some clocks, for example in the dorsolateral prefrontal cortex with Horvath1, Horvath2, Levine, Hannum, and Lin (Liu et al. Citation2020). Considering differences between clock generations and individual clock formations, clocks demonstrate different associations with underlying biological pathways. Utilising appropriate clocks in future urban environmental-neurogenerative disease research will be important. Focusing analyses using second- and third-generation clocks may be more reliable in the prediction of neurodegenerative risk. Further exploration of what type of underlying pathological pathways each clock may best associate with will be additionally helpful.
Epigenome-wide association studies
EWAS primarily analyse DNAm levels across the genome with single-site resolution (Campagna et al. Citation2021), taking either a cross-sectional or longitudinal approach. EWAS can identify epigenetic markers, epigenetic modifications which are associated with a statistically significant higher risk of disease. Alternatively, EWAS can uncover markers of resilience, epigenetic modifications associated with statistically significant lower risk of disease. These resilience markers are of considerable interest as they indicate a protective mechanism which could be explored in preventative policy of disease risk (Rakyan et al. Citation2011).
Current findings in epigenome-wide association studies
Neurodegenerative disease focused EWAS have located differentially methylated loci in cortex derived DNA (Dashtipour et al. Citation2017, Li et al. Citation2020, Smith et al. Citation2021) which serve as markers for the diseases. EWAS have also been carried out with a specified focus on urban environment factors. Long term exposure to air pollution, including PM and NO2, has been associated with differentially methylated genes and regions (Lee et al. Citation2019, Chi et al. Citation2022). Chi et al., for example, found that PM2.5 exposure was associated with cg05926640 (false discovery rate (FDR) <0.05) in monocytes (Chi et al. Citation2022), with this CpG site being commonly associated with the TOMM20 protein-coding gene (Translocase of Outer Mitochondrial Membrane 20). The TOM20 protein synthesised from TOMM20 transcription has been implicated in Parkinson’s disease through alteration of mitochondrial protein import (Li et al. Citation2021). Noise exposure related to different modes of transport and air pollution was investigated in a study by Eze et al. (Citation2020), who found that PM2.5 increased methylation of a FARS2 gene region (Phenylalanyl-TRNA Synthetase 2). The FARS2 gene has been implicated in methylation-related changes in Multiple Sclerosis lesions (Schirmer et al. Citation2019, Kular and Jagodic Citation2020). Some EWAS complete additional pathway-based enrichment analysis which gives an indicator of functional biological pathways which may be influenced by modifications. In Lee et al., pathway analysis highlighted enrichment in inflammatory and cardiovascular pathways from PM2.5 and NO2 exposure (Lee et al. Citation2019).
Other urban factors which have been examined through EWAS include greenness. Residential greenness measured using the normalised difference vegetation index and enhanced vegetation index was associated with differential methylation in a study by Xu et al. (Citation2021). A significant association was found for the CNP gene promoter (2‘,3’-Cyclic Nucleotide 3’ Phosphodiesterase), a gene previously considered to play a role in Multiple Sclerosis (Parade et al. Citation2017, Kao et al. Citation2020). A separate study (Alfano et al. Citation2023) analysing maternal exposure to green space and the methylation levels in umbilical cord blood found associations with green space and the methylation of genes including HTR2A (5-Hydroxytryptamine Receptor 2A), implicated in major depressive disorder (Parade et al. Citation2017, Kao et al. Citation2020).
Issues to overcome to maximise epigenome-wide association study usage in urban environmental-neurodegenerative research
Authors of EWAS concerning urban environment factors are in universal agreement that more studies are required in this field, including multiple validation of findings and further exploration of associations and biological pathways (Mostafavi et al. Citation2018, Chi et al. Citation2022, Freydenzon et al. Citation2022). EWAS results are most convincing when the studies are performed robustly using adequately powered cohorts and including replication of top-ranked findings. However, EWAS projects are expensive and, as they are relatively newly employed in this field, truly independent datasets that explore the same variables are rarely available for replication. Advancing the available data to perform EWAS will be highly beneficial to the field, enabling the identification and validation of epigenetic markers which can be further used in causative analysis. EWAS focused on exploring the neurodegenerative disease outcome as a consequence of urban environmental exposures are required.
To minimize false positives from future large-scale EWAS, appropriate adjustment for multiple testing is essential, ideally followed by validation or replication from independent cohorts. Both single site resolution and combined estimators/composite biomarkers should be considered for the phenotype and environmental exposures.
Harnessing Mendelian randomization to explore causation in the pathway to disease
While a person’s genetic risk of disease is largely fixed at conception, due to the modifiable nature of epigenetic changes, their epigenetic risk score alters across the life course (Relton and Smith Citation2015). Challenges exist in determining if epigenetic changes are a cause or consequence of disease; however, strategies have been developed to tease this out through functional studies or MR (Rakyan et al. Citation2011, Paul and Beck Citation2014).
MR has been adopted as an epidemiological research technique, effectively acting as in silico randomised control trials (RCTs). RCTs are a gold standard research technique (Cartwright Citation2009) but can be unethical and impractical for the requirements of epidemiological study. Observational studies on the other hand have the limitation of being unable to measure all possible confounders. MR overcomes this problem through the use of genetic variants (single nucleotide polymorphisms) as proxies (instrumental variables) for specific exposures. Because genetic variants are defined at conception, they are independent of confounders, allowing the investigation of causation.
For analysis concerning epigenetic modifications such as DNAm, a methylation site cannot be used directly as an instrumental variable in the MR process, as they are modified throughout the life course and therefore have potential to be influenced by confounders. Genetic variants significantly associated with certain DNAm changes, which occur due to specific environmental exposures, are therefore used as proxies to determine the causal effect of these risk-associated DNAm changes on disease outcomes (Smith and Ebrahim Citation2003, Thanassoulis and O’Donnell Citation2009) (). For a variant to be used as an instrumental variable within MR, it is assumed that the variant only has a relationship with the disease outcome through its relationship with the risk factor (Didelez and Sheehan Citation2007). MR can provide strong statistical evidence for causal relationships, which can then be explored in biological pathway research to identify how urban environment factors are influencing the epigenome to contribute to neurodegenerative risk.
Figure 1. Directed acyclic graph to explain how causative investigation through Mendelian randomization could be used in urban environment-neurodegenerative research.
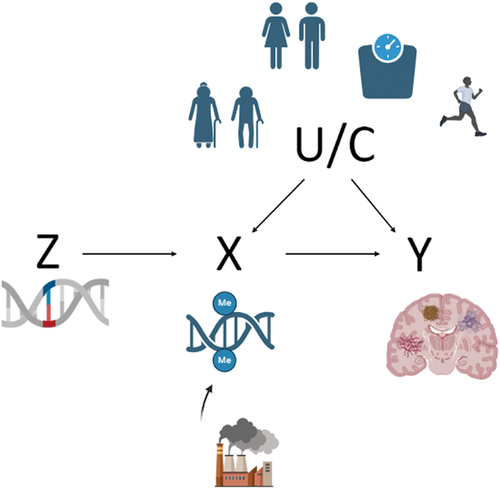
Multiple variations on the traditional MR approach have been developed. Bidirectional MR evaluates the direction of causation (Zheng et al. Citation2017) through performing MR in both the forward and reverse direction of the acyclic graph. Two-sample MR has been developed which allows the risk factor and outcome instruments to be taken from two different sample populations (Pierce and Burgess Citation2013) in both GWAS and EWAS meta-consortia (Burgess et al. Citation2015, Walker et al. Citation2019). A recent study aimed to test the causal effect of alcohol-related DNAm on colon cancer risk (Zhou et al. Citation2022). This study used a two-sample MR approach by selecting genetic instruments of predisposition to alcohol drinking from previously published GWAS and assessing the relationship of these variants with colon cancer risk using separate previously published GWAS.
One of the difficulties faced by researchers using MR is acquiring appropriate genetic variants (used as instrumental variable Z) for CpG sites considering methylation at CpG sites is altered by both underlying genetics and the environment (Hannon et al. Citation2018). To enable causal links to be assessed, it is important that genetic variants account for enough variability at CpG sites of interest and have an F statistic > 10, considered acceptable instrument strength (Burgess et al. Citation2011).
For urban environmental-neurodegenerative research, MR is a useful technique as the downstream consequences (epigenetic modifications) of urban environment factors can be assessed. Assessing causative relationships between methylation changes and neurodegenerative disease will enable the identification of environmental exposures which can be modified.
Future research
There is a growing need for identifying urban environmental–neurodegenerative relationships. Recent analyses have independently associated epigenetic modifications and urban environment factors with neurodegenerative diseases. This review highlights the need to integrate both these variables in analysis. Through identifying relationships between urban environmental exposures and epigenetic modifications, it will then be possible to expand on biological pathways, which may have cognitive consequences. This will enable opportunities to collaborate with those in environmental health policymaking to alleviate molecular risk of disease.
Using epigenetic analytical techniques to analyse causal relationships
Combining EWAS and MR enables causative relationships to be identified between urban environment factors and neurodegenerative diseases. Identifying these relationships is an important starting point. In other fields, MR studies have made use of genetic variants to investigate disease causation related to epigenetics (Dekkers et al. Citation2016). It is rare that urban environment exposures and their influence on neurodegenerative diseases are explored in this way. A multi-omic approach, exploring genetic and epigenetic variation, as well as harnessing methods such as MR, will be greatly beneficial in beginning to understand the complex interactions to help translate research into preventative policy. It would be beneficial for future work to identify epigenetic markers through EWAS analysis associated with important urban environment factors and also consider co-exposure. These epigenetic markers enable MR analyses to explore complex causal associations which can be interpreted with appropriate caution.
Epigenetic clocks are useful in the prediction of biological age which has been associated with neurodegenerative risk (Gonzales et al. Citation2022). Formulating clocks traditionally utilises blood samples; however, recently, the Cortical clock (Shireby et al. Citation2020) has been developed using human post-mortem cortical tissue as opposed to the traditional systemic blood samples. The Cortical clock identified 347 DNAm sites which can predict age in the human cortex (Shireby et al. Citation2020) and may have higher sensitivity to specific brain pathological epigenetic changes (Grodstein et al. Citation2021, Gonzales et al. Citation2022). Further validation and replication of the Cortical clocks sensitivity could make it a prominent feature of biological and specifically cortical ageing. Expanding work on both cortical and traditional blood made epigenetic clocks will aid in identifying underlying epigenetic mechanisms of ageing in the brain, providing useful data in which urban environment methylation-related changes can be linked.
Disentangling complex interactions
It is important that future research does not solely focus on more commonly researched urban environment factors. For example, there is a larger volume of research including EWAS centred around air pollution, commonly PM2.5, while the literature on noise and light pollution is sparse. Moreover, from our understanding, there are very limited published EWAS concerning noise or light pollution using human samples. This limits the knowledge within the field on the potential impact of these exposures at the epigenetic level. The inclusion of these lesser researched factors will aid in filling in the bigger picture as to how the whole urban environment impacts disease risk. The inclusion of more recently implicated and novel urban environmental pollutants, including microplastics (Meng et al. Citation2020) and particulates as a consequence of wildfires (Li et al. Citation2023), would further bridge the knowledge gap. Additionally, current research commonly investigates a singular factor, such as PM or chronic noise exposure, and the impact on neurodegenerative disease. These studies are important in building an understanding of how these individual factors influence disease risk. However, urban environmental exposure is multifactorial, which can impact both the epigenome and neurodegenerative risk simultaneously. Despite the added complexity, it would be beneficial for future research to begin the investigation of co-exposures.
Additionally, social factors such as socioeconomic status and education can confound and mediate relationships between urban environment factors and disease (Clifford et al. Citation2016, Peters et al. Citation2019, Hajat et al. Citation2021). Social factors have also been associated with similar epigenetic mechanisms to urban environment factors such as accelerated biological ageing (Fiorito et al. Citation2017). Researchers should consider this overlap when interpreting their results and always consider the relationship between environmental and social factors.
Disentangling the interactions as to how environmental exposures interact with each other and other social factors to influence the modification of the epigenome, and ultimately impact neurodegenerative disease susceptibly, will be a complex future goal in the field.
Biological pathways
Biological pathways tract the body’s cellular progression from exposure to disease states. They often involve complex molecular mechanisms including epigenetic modifications (McGowan and Roth Citation2015, Alameda et al. Citation2022). Few papers at present have focused on exploring the biological pathways which explain the link between the urban environment and neurodegenerative disease through epigenetics. This lack of research has left multiple unanswered questions and hypotheses. Through reviewing the literature, a summary of the proposed biological pathways can be seen in .
Figure 2. The biological pathways involving epigenetic mechanisms which link urban environment pollutants and neurodegenerative disease.
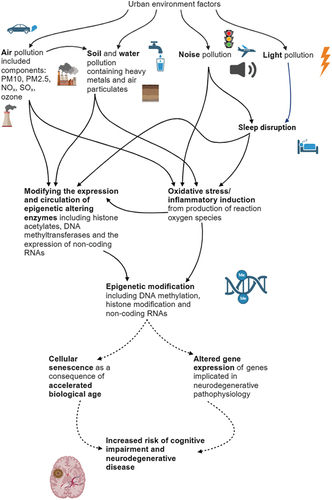
Figure 3. Resilience pathways involving epigenetic mechanisms which link urban environment factors and associated behaviours with neurodegenerative disease.
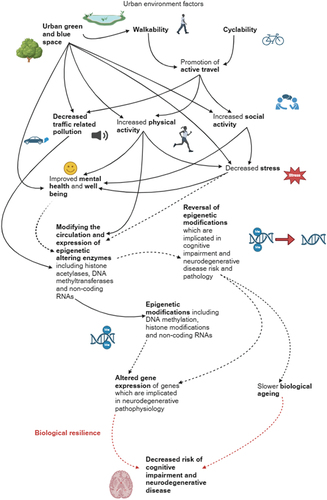
Pathways involving urban environment pollutants
Urban pollutants can influence the activity of the enzymes involved in epigenetic modification (epigenetic machinery) including DNA methyltransferase (DNMT). PM has been evidenced to decrease DNMT expression, facilitating hypomethylation in skin keratinocytes (Ryu et al. Citation2019). PM has also been associated with hypomethylation via DNMT3B down regulation in blood (Wang et al. Citation2020). Histone post-translational modifying enzymes have been associated with air pollution exposure in post-mortem brain tissue of urban residents with pathological hallmarks of Alzheimer’s disease. Global expression of H3K9me2 and H3K9me3 (involved in histone methylation) was decreased in those with pathological hallmarks of the disease (neurofibrillary tangles and amyloid deposits) when compared to the controls (Calderón-Garcidueñas et al. Citation2020). Heavy metals implicated as soil and water pollutants have also been evidenced to alter DNTM expression (Ryu et al. Citation2015). For example, Cadmium has been shown to facilitate DNA hypomethylation and hypomethylation through altering the activity of DNMT (Takiguchi et al. Citation2003, Ryu et al. Citation2015, Athanasopoulos et al. Citation2016).
The number of studies investigating the influence of environment factors on epigenetic machinery is not extensive and still leaves questions unanswered. It is not clear how or why pollutants target these specific enzymes, nor how many enzymes pollutants interact with, subsequently altering their activity. The influence of urban environment factors beyond air pollutants and heavy metal constitutes of soil and water pollution remains largely unexplored in this context, highlighting an interesting area of future research. An additional research gap in the field is how dysregulation of epigenetic machinery enzymes, due to urban environmental exposures, disrupts gene expression and regulation, and the potential implications on cognitive impairment and neurodegenerative diseases.
Oxidative stress is an additional mechanism which has been evidenced to influence cognitive impairment and neurodegenerative risk which has been summarised in a review by Kandlur et al. (Citation2020). Various pollutants, including air (Hahad et al. Citation2020, Murata et al. Citation2022), noise (Daiber et al. Citation2020), water and soil (Münzel et al. Citation2022) pollutants, are involved in inflammatory induction resulting in oxidative stress. Indeed, the potential for oxidative stress to itself induce these epigenetic changes is briefly discussed by Kandlur et al. (Citation2020). Oxidative stress has been evidenced to influence DNA methylation and is suspected to alter the activity of epigenetic machinery (Guillaumet-Adkins et al. Citation2017); however, the literature is lacking on the mechanism(s) by which it specifically contributes to neurodegenerative risk. Further exploration of this pathway will provide much needed insight. Furthermore, oxidative stress has also been evidenced to be associated with both cellular senescence (Holmström and Finkel Citation2014) and biological ageing (Warraich et al. Citation2020); processes which are related with progressive epigenetic modification.
Current evidence suggests that epigenetic modifications influence the risk of cognitive impairment and neurodegenerative disease through either accelerated biological ageing (Levine et al. Citation2015, Wu et al. Citation2021, Tang et al. Citation2022), which can be linked to cellular senescence, or through gene expression changes (Berson et al. Citation2018, Sharma et al. Citation2020) having downstream molecular consequences which contribute to pathophysiology. Research surrounding which epigenetic modifications are consistently involved in neurodegenerative pathophysiology and specific outlines of molecular cascades affected by these modifications for example, is currently not comprehensive enough for sound understanding (Landgrave-Gómez et al. Citation2015, Delgado-Morales et al. Citation2017).
Other proposed biological pathways may involve circadian and sleep disruption, a consequence of noise and light pollution (Halperin Citation2014, Cho et al. Citation2015, Patel Citation2019). The epigenome has the ability to influence circadian cycles (Hudec et al. Citation2020), while sleep deprivation is suggested to be epigenetically modifying and have implications on epigenetic ageing, summarised in a review by Gaine et al. (Citation2018). This review provides several examples of the implications of sleep deprivation on neurodegenerative diseases, including decreased histone acetylation observed in a Drosophila model of Alzheimer’s disease (Pirooznia et al. Citation2012, Gaine et al. Citation2018). Additionally, sleep deprivation has been evidenced to contribute to oxidative stress and inflammatory induction (Villafuerte et al. Citation2015, Kempf et al. Citation2019, Daiber et al. Citation2020). Current evidence has not been able to provide a comprehensive understanding of the impact of noise and light pollution on the human epigenome. Studying the impact of sleep deprivation using human epigenetic profiles provides an opportunity to deepen our understanding of this potential pathway.
Resilience pathways
Urban environment factors also have the capacity to operate in biological pathways in which epigenetic reversal and biological resilience may decrease the risk of cognitive impairment and neurodegenerative disease. Walkability and cyclability contribute to active travel (Fraser and Lock Citation2011, Kim et al. Citation2020), thereby increasing the physical activity levels of individuals. Literature has also directly associated urban green and blue space with increased physical activity levels (Richardson et al. Citation2013, Besser Citation2021). Physical activity has been shown to impact various aspects of health, namely cardiometabolic health (Rao et al. Citation2022). With atherosclerotic complications being a risk factor for neurodegenerative diseases, especially vascular dementia (Huang et al. Citation2021), improving heart and circulatory health has a potentially direct impact on reducing neurodegenerative risk.
Physical activity has also been evidenced to modify the epigenome (Plaza-Diaz et al. Citation2022). Physical activity has been found to influence the expression of multiple genes which are involved in neurodegenerative risk and pathophysiology (Panes et al. Citation2022), including PGC-1a (Peroxisome Proliferator-activated Receptor-gamma Coactivator 1 alpha) which is a key regulatory gene in mitochondrial biogenesis (Plaza-Diaz et al. Citation2022). PGC-1a hypomethylation occurs post-exercise (Rasmussen et al. Citation2014, Maasar et al. Citation2021), upregulating transcription. PGC-1a has been implicated in multiple neurodegenerative diseases and its upregulation is considered neuroprotective in neurodegenerative disease (Panes et al. Citation2022). In Alzheimer’s disease for example, PGC-1a downregulation is a critical risk factor for early onset of disease (Terada et al. Citation2020, Dong et al. Citation2020). PGC-1a hypomethylation through physical activity therefore suggests a resilience mechanism. This mechanism likely involves synaptic and neural protection from oxidative stress, although a confirmed pathway is yet to be identified.
Urban green and blue space have been associated with other health enhancing factors including promoting social activity (Maas et al. Citation2009, Jennings and Bamkole Citation2019), reducing stress (Roe et al. Citation2013, Gidlow et al. Citation2016a, Slawsky et al. Citation2022), improving mental health and well-being (Gascon et al. Citation2015, Hannah et al. Citation2015, Hystad et al. Citation2019), and mitigating the effects of traffic-related pollution (Gidlöf-Gunnarsson and Öhrström Citation2007, Dadvand et al. Citation2012). Health enhancing factors are interconnected, for example, social activity aids in stress reduction (Umberson and Karas Montez Citation2010); with active travel and physical activity also aiding their promotion (Carek et al. Citation2011, Cheng et al. Citation2019, Zukowska et al. Citation2022). Similar to physical activity, it is suggested that health enhancing factors may contribute to epigenetic modifications through altering epigenetic machinery. Stress (Hing et al. Citation2018), social and physical activity (Fernandes et al. Citation2017, Światowy et al. Citation2021), mental health conditions (Crawford et al. Citation2018, Wiegand et al. Citation2021) and pollutant exposure (Gondalia et al. Citation2019, Chi et al. Citation2022) have all been associated with differential methylation profiles. Chronic unpredictable stress in animal models for example, has been evidenced to alter DNMT3a activity in the pre-frontal cortex. Wei et al. study found that stressed rats displayed overexpression of DNMT3a, exhibited differential methylation profiles and cognitive changes including impaired memory (Wei et al. Citation2021). As some health enhancing behaviours can act similarly to discussed urban environmental pollutants, altering epigenetic machinery (Hunter et al. Citation2019, Rey et al. Citation2019), there is potential for promotion or reversal of epigenetic modifications from different exposure levels of these behaviours. Increasing physical activity or reducing stress may promote modifications related to a resilience pathway, decreasing the risk of cognitive impairment or neurodegenerative disease. Altering exposure from low to higher physical activity levels may also reverse epigenetic modifications which contribute to cognitive impairment of neurodegenerative risk. Epigenetic reversal has the additional potential benefit of slowing the effect of biological age acceleration, potentially providing a further resilience pathway.
Current research is able to elucidate biological pathways; however, it is not possible to confirm full pathways from environmental exposure to the disease outcome. Few studies have focused on investigating epigenetic modifications as the consequence of urban environmental exposures and the impact of this molecular change on cognitive impairment and neurodegenerative pathophysiology. Overall, current research has not provided a comprehensive understanding of biological resilience and epigenetic reversal in the context of cognitive impairment and neurodegenerative disease. Further work is required to explore how resilience can potentially be promoted and the extent to which epigenetic modifications can be reversed through altering environmental exposures.
An integral future direction in the field is to not only identify epigenetic markers, including those which indicate resilience mechanisms, but also exploring their influence on biological pathways. Multi-omic approaches will be especially important to explore this research area, considering individual urban environment factors likely contribute to multiple molecular risk pathways (Wu et al. Citation2023). Few studies have used multi-omic approaches, incorporating multiple types of environmental data to understand their interaction and subsequent impact on molecular biology. Maitre et al. recently used multi-omic approaches to explore the early life exposome (Maitre et al. Citation2022) for example. Utilising this multi-omic approach will create a more comprehensive understanding of whole complex pathways and system interconnections. Translating this molecular knowledge into both urban and public policy interventions has future potention for therapeutic protection from neurodegenerative diseases.
Concluding remarks
Urban environmental exposures have been associated with cognitive impairment and neurodegenerative disease. The biological pathways by which urban environment factors contribute to neurodegenerative disease have been summarised in this review. However, there are many hypothesised pathways that have yet to be tested and confirmed, and many unanswered questions remain. Further molecular analysis is required to tease out exactly how urban environment factors alter underlying molecular cascades to result in the disease outcome. Epigenetics is a research field which can aid the development of biological pathways considering that epigenetic modification contributes to neurodegenerative risk, and urban environment factors can modify the epigenome. Through utilising epigenetic analytical techniques discussed in this review it will be possible to expand research of the environmental consequences on human cognition and neurodegenerative status. It is hoped that developing knowledge of molecular risk from environmental exposures will aid in the creation and development of public and urban policy to prevent cognitive impairment and neurodegenerative disease.
Author contributions
SG: conceptualization, interpretation, writing (original draft, review & editing) and visualization. CH: interpretation, writing (review & editing) and visualization. BM: conceptualization, interpretation, writing (review & editing) and visualization. AJM: conceptualization, interpretation, writing (review & editing) and visualization. RH: conceptualization, interpretation, writing (review & editing) and visualization. All authors have read and approved the manuscript for submission.
Disclosure statement
No potential conflict of interest was reported by the authors.
Additional information
Funding
Notes on contributors
Sophie Glover
The SPACE (Supportive Environments for Physical and Social Activity, Healthy Ageing and Cognitive Health) research project at Queen’s University Belfast aims to assess the impact of the urban environment on brain health, namely relationships between the urban environment and mild cognitive impairment. The project has an interest in complex systems research to advance our universal understanding of how physical urban pollutants, physical and social activity, and green environments can impact our brain health as we age. The project has an interest in engaging with the public and policy makers to increase understanding on the topic and ensure policy takes health implications into consideration when creating environments.
References
- Adekomi, D., 2017. Lead induces inflammation and neurodegenerative changes in the rat medial prefrontal cortex. Anatomt-an international journal of experimental and clinical anatomy, 11 (2), 79–86. doi:10.2399/ana.17.015.
- Ahmad, W., et al., 2021. Toxic and heavy metals contamination assessment in soil and water to evaluate human health risk. Scientific reports, 11 (1), 17006. doi:10.1038/s41598-021-94616-4.
- Alameda, L., et al., 2022. Can epigenetics shine a light on the biological pathways underlying major mental disorders? Psychological medicine, 52 (9), 1645–1665. doi:10.1017/S0033291721005559.
- Alemany, S., et al., 2021. Associations between air pollution and biomarkers of Alzheimer’s disease in cognitively unimpaired individuals. Environment international, 157, 106864. doi:10.1016/j.envint.2021.106864.
- Alfano, R., et al., 2023. Epigenome-wide analysis of maternal exposure to green space during gestation and cord blood DNA methylation in the ENVIRONAGE cohort. Environmental research, 216, 114828. doi:10.1016/j.envres.2022.114828.
- Alghamdi, B.S., 2018. The neuroprotective role of melatonin in neurological disorders. Journal of neuroscience research, 96 (7), 1136–1149. doi:10.1002/jnr.24220.
- Athanasopoulos, D., Karagiannis, G., and Tsolaki, M., 2016. Recent findings in Alzheimer disease and nutrition focusing on epigenetics. Advances in nutrition, 7 (5), 917–927. doi:10.3945/an.116.012229.
- Atkinson, R.W., et al., 2010. Urban ambient particle metrics and health: a time-series analysis. Epidemiology, 21 (4), 501–511. doi:10.1097/EDE.0b013e3181debc88.
- Bagepally, B.S., et al., 2021. Association between aluminium exposure and cognitive functions: a systematic review and meta-analysis. Chemosphere, 268, 128831. doi:10.1016/j.chemosphere.2020.128831
- Bakulski, K.M., et al., 2020. Heavy metals exposure and Alzheimer’s disease and related dementias. Journal of Alzheimer’s disease: JAD, 76 (4), 1215–1242. doi:10.3233/JAD-200282.
- Baranyi, G., et al., 2022. Life-course exposure to air pollution and biological ageing in the Lothian birth cohort 1936. Environment international, 169, 107501. doi:10.1016/j.envint.2022.107501.
- Bell, C.G., et al., 2019. DNA methylation aging clocks: challenges and recommendations. Genome biology, 20 (1), 249. doi:10.1186/s13059-019-1824-y.
- Belsky, D.W., et al., 2020. Quantification of the pace of biological aging in humans through a blood test, the DunedinPoAm DNA methylation algorithm. Elife, 9. doi:10.7554/eLife.54870.
- Benabed, A. and Boulbair, A., 2022. PM10, PM2.5, PM1, and PM0.1 resuspension due to human walking. Air quality, atmosphere, & health, 15 (9), 1547–1556. doi:10.1007/s11869-022-01201-3.
- Berson, A., et al., 2018. Epigenetic Regulation in Neurodegenerative Diseases. Trends in neurosciences, 41 (9), 587–598. doi:10.1016/j.tins.2018.05.005.
- Besser, L., 2021. Outdoor green space exposure and brain health measures related to Alzheimer’s disease: a rapid review. BMJ open, 11 (5), e043456. doi:10.1136/bmjopen-2020-043456.
- Besser, L.M., et al., 2021. Associations between neighborhood park access and longitudinal change in cognition in older adults: the multi-ethnic study of atherosclerosis. Journal of Alzheimer’s disease, 82 (1), - 233. doi:10.3233/JAD-210370.
- Beyer, K.M., et al., 2014. Exposure to neighborhood green space and mental health: evidence from the survey of the health of Wisconsin. International journal of environmental research and public health, 11 (3), 3453–3472. doi:10.3390/ijerph110303453.
- Bondy, S.C. and Campbell, A., 2017. Water quality and brain function. International journal of environmental research and public health, 15 (1), 2. doi:10.3390/ijerph15010002.
- Burgess, S., et al., 2015. Using published data in Mendelian randomization: a blueprint for efficient identification of causal risk factors. European journal of epidemiology, 30 (7), 543–552. doi:10.1007/s10654-015-0011-z.
- Burgess, S., Thompson, S.G., and Collaboration, C.C.G., 2011. Avoiding bias from weak instruments in Mendelian randomization studies. International journal of epidemiology, 40 (3), 755–764. doi:10.1093/ije/dyr036.
- Cabral Pinto, M.M.S., et al., 2018. Human predisposition to cognitive impairment and its relation with environmental exposure to potentially toxic elements. Environmental geochemistry and health, 40 (5), 1767–1784. doi:10.1007/s10653-017-9928-3.
- Caicedo, A., Mayorga, M., and Estrada, M., 2021. Modelling individual perception of barriers to bike use. Transportation Research Procedia, 58, 293–300. doi:10.1016/j.trpro.2021.11.040
- Calderón-Garcidueñas, L., et al., 2020. Reduced repressive epigenetic marks, increased DNA damage and Alzheimer’s disease hallmarks in the brain of humans and mice exposed to particulate urban air pollution. Environmental research, 183, 109226. doi:10.1016/j.envres.2020.109226.
- Campagna, M.P., et al., 2021. Epigenome-wide association studies: current knowledge, strategies and recommendations. Clinical epigenetics, 13 (1), 214. doi:10.1186/s13148-021-01200-8.
- Carek, P.J., Laibstain, S.E., and Carek, S.M., 2011. Exercise for the treatment of depression and anxiety. The international journal of psychiatry in medicine, 41 (1), 15–28. doi:10.2190/PM.41.1.c.
- Cartwright, N., 2009. What are randomised controlled trials good for? Philosophical studies, 147 (1), 59. doi:10.1007/s11098-009-9450-2.
- Cazaly, E., et al., 2019. Making sense of the epigenome using data integration approaches. Frontiers in pharmacology, 10, 126. doi:10.3389/fphar.2019.00126.
- Chandra, M., et al., 2022. Air pollution and cognitive impairment across the life course in humans: a systematic review with specific focus on income level of study area. International journal of environmental research and public health, 19 (3), 1405. doi:10.3390/ijerph19031405.
- Chen, H., et al., 2017a. Exposure to ambient air pollution and the incidence of dementia: a population-based cohort study. Environment international, 108, 271–277. doi:10.1016/j.envint.2017.08.020.
- Chen, H., et al., 2017b. Living near major roads and the incidence of dementia, Parkinson’s disease, and multiple sclerosis: a population-based cohort study. Lancet, 389 (10070), 718–726. doi:10.1016/S0140-6736(16)32399-6.
- Chen, Y., et al., 2022. Long-term exposure to outdoor light at night and mild cognitive impairment: a nationwide study in Chinese veterans. Science of the total environment, 847, 157441. doi:10.1016/j.scitotenv.2022.157441.
- Cheng, L., et al., 2019. Active travel for active ageing in China: the role of built environment. Journal of transport geography, 76, 142–152. doi:10.1016/j.jtrangeo.2019.03.010.
- Chi, G.C., et al., 2022. Epigenome-wide analysis of long-term air pollution exposure and DNA methylation in monocytes: results from the multi-ethnic study of atherosclerosis. Epigenetics, 17 (3), 1900028. doi:10.1080/15592294.2021.1900028.
- Chin-Chan, M., Navarro-Yepes, J., and Quintanilla-Vega, B., 2015. Environmental pollutants as risk factors for neurodegenerative disorders: Alzheimer and Parkinson diseases. Frontiers in cellular neuroscience, 9, 124. doi:10.3389/fncel.2015.00124
- Cho, Y., et al., 2015. Effects of artificial light at night on human health: a literature review of observational and experimental studies applied to exposure assessment. Chronobiology international, 32 (9), 1294–1310. doi:10.3109/07420528.2015.1073158.
- Clifford, A., et al., 2016. Exposure to air pollution and cognitive functioning across the life course – a systematic literature review. Environmental research, 147, 383–398. doi:10.1016/j.envres.2016.01.018.
- Colwell, M.L., et al., 2023. Epigenetics and the exposome: DNA methylation as a proxy for health impacts of prenatal environmental exposures. Exposome, 3 (1). doi:10.1093/exposome/osad001.
- Cooke, P., Janowitz, H., and Dougherty, S.E., 2022. Neuronal redevelopment and the regeneration of neuromodulatory axons in the adult mammalian central nervous system. Frontiers in cellular neuroscience, 16, 872501. doi:10.3389/fncel.2022.872501
- Crawford, B., et al., 2018. DNA methylation and inflammation marker profiles associated with a history of depression. Human molecular genetics, 27 (16), 2840–2850. doi:10.1093/hmg/ddy199.
- Crouch, J., et al., 2022. Epigenetic regulation of cellular senescence. Cells, 11 (4), 672. doi:10.3390/cells11040672.
- Cui, B., et al., 2012. Chronic noise exposure causes persistence of tau hyperphosphorylation and formation of NFT tau in the rat hippocampus and prefrontal cortex. Experimental neurology, 238 (2), 122–129. doi:10.1016/j.expneurol.2012.08.028.
- Dadvand, P., et al., 2012. Surrounding greenness and exposure to air pollution during pregnancy: an analysis of personal monitoring data. Environmental health perspectives, 120 (9), 1286–1290. doi:10.1289/ehp.1104609.
- Daiber, A., et al., 2020. Oxidative stress and inflammation contribute to traffic noise-induced vascular and cerebral dysfunction via uncoupling of nitric oxide synthases. Redox biology, 34, 101506. doi:10.1016/j.redox.2020.101506.
- Dashtipour, K., et al., 2017. Hypermethylation of Synphilin-1, Alpha-Synuclein-Interacting Protein (SNCAIP) gene in the cerebral cortex of patients with sporadic Parkinson’s disease. Brain sciences, 7 (7), 74. doi:10.3390/brainsci7070074.
- Dekkers, K.F., et al., 2016. Blood lipids influence DNA methylation in circulating cells. Genome biology, 17 (1), 138. doi:10.1186/s13059-016-1000-6.
- Delgado-Morales, R., et al., 2017. Epigenetic mechanisms during ageing and neurogenesis as novel therapeutic avenues in human brain disorders. Clinical epigenetics, 9 (1), 67. doi:10.1186/s13148-017-0365-z.
- de Paiva Vianna, K.M., Alves Cardoso, M.R., and Rodrigues, R.M., 2015. Noise pollution and annoyance: an urban soundscapes study. Noise & health, 17 (76), 125–133. doi:10.4103/1463-1741.155833.
- Didelez, V. and Sheehan, N., 2007. Mendelian randomization as an instrumental variable approach to causal inference. Statistical methods in medical research, 16 (4), 309–330. doi:10.1177/0962280206077743.
- Di Micco, R., et al., 2021. Cellular senescence in ageing: from mechanisms to therapeutic opportunities. Nature reviews molecular cell biology, 22 (2), 75–95. doi:10.1038/s41580-020-00314-w.
- Dominguez, L.J., et al., 2021. Nutrition, physical activity, and other lifestyle factors in the prevention of cognitive decline and dementia. Nutrients, 13 (11), 4080. doi:10.3390/nu13114080.
- Dong, Y.T., et al., 2020. Silent mating-type information regulation 2 homolog 1 attenuates the neurotoxicity associated with Alzheimer disease via a mechanism which may involve regulation of peroxisome proliferator-activated receptor gamma coactivator 1-α. The American journal of pathology, 190 (7), 1545–1564. doi:10.1016/j.ajpath.2020.03.015.
- Dumax-Vorzet, A.F., et al., 2015. Cytotoxicity and genotoxicity of urban particulate matter in mammalian cells. Mutagenesis, 30 (5), 621–633. doi:10.1093/mutage/gev025.
- Duran-Aniotz, C., et al., 2021. Amyloid pathology arrangements in Alzheimer’s disease brains modulate in vivo seeding capability. Acta neuropathologica communications, 9 (1), 56. doi:10.1186/s40478-021-01155-0.
- Escobar, C., et al., 2011. Circadian disruption leads to loss of homeostasis and disease. Sleep disorders, 2011, 1–8. doi:10.1155/2011/964510
- Eze, I.C., et al., 2020. Genome-wide DNA methylation in peripheral blood and long-term exposure to source-specific transportation noise and air pollution: the SAPALDIA study. Environmental health perspectives, 128 (6), 67003. doi:10.1289/EHP6174.
- Fathabadi, B., et al., 2018. Comparison of blood lead levels in patients with Alzheimer’s disease and healthy people. American journal of Alzheimer’s disease and other dementias, 33 (8), 541–547. doi:10.1177/1533317518794032.
- Fernandes, J., Arida, R.M., and Gomez-Pinilla, F., 2017. Physical exercise as an epigenetic modulator of brain plasticity and cognition. Neuroscience & biobehavioral reviews, 80, 443–456. doi:10.1016/j.neubiorev.2017.06.012
- Fiorito, G., et al., 2017. Social adversity and epigenetic aging: a multi-cohort study on socioeconomic differences in peripheral blood DNA methylation. Scientific reports, 7 (1), 16266. doi:10.1038/s41598-017-16391-5.
- Flies, E.J., et al., 2019. Urban-associated diseases: candidate diseases, environmental risk factors, and a path forward. Environment international, 133, 105187. doi:10.1016/j.envint.2019.105187.
- Fraser, S.D.S. and Lock, K., 2011. Cycling for transport and public health: a systematic review of the effect of the environment on cycling. European journal of public health, 21 (6), 738–743. doi:10.1093/eurpub/ckq145.
- Freydenzon, A., et al., 2022. Association between DNA methylation variability and self-reported exposure to heavy metals. Scientific Reports, 12 (1), 10582. doi:10.1038/s41598-022-13892-w.
- Fuller, R., et al., 2022. Pollution and health: a progress update. The lancet planet health, 6 (6), e535–e47. doi:10.1016/S2542-5196(22)00090-0.
- Gaine, M.E., Chatterjee, S., and Abel, T., 2018. Sleep deprivation and the epigenome. Frontiers in neural circuits, 12, 14. doi:10.3389/fncir.2018.00014
- Gao, X., et al., 2022. Short-term exposure of PM(2.5) and epigenetic aging: a quasi-experimental study. Environmental science & technology, 56 (20), 14690–14700. doi:10.1021/acs.est.2c05534.
- Gascon, M., et al., 2015. Mental health benefits of long-term exposure to residential green and blue spaces: a systematic review. International journal of environmental research and public health, 12 (4), 4354–4379. [Internet]. doi:10.3390/ijerph120404354.
- Gibney, E.R. and Nolan, C.M., 2010. Epigenetics and gene expression. Heredity, 105 (1), 4–13. doi:10.1038/hdy.2010.54.
- Gidlöf-Gunnarsson, A. and Öhrström, E., 2007. Noise and well-being in urban residential environments: the potential role of perceived availability to nearby green areas. Landscape and urban planning, 83 (2), 115–126. doi:10.1016/j.landurbplan.2007.03.003.
- Gidlow, C.J., et al., 2016a. Natural environments and chronic stress measured by hair cortisol. Landscape and urban planning, 148, 61–67. doi:10.1016/j.landurbplan.2015.12.009.
- Gidlow, C.J., et al., 2016b. Where to put your best foot forward: psycho-physiological responses to walking in natural and urban environments. Journal of environmental psychology, 45, 22–29. doi:10.1016/j.jenvp.2015.11.003.
- Gondalia, R., et al., 2019. Methylome-wide association study provides evidence of particulate matter air pollution-associated DNA methylation. Environment international, 132, 104723. doi:10.1016/j.envint.2019.03.071.
- Gonzales, M.M., et al., 2022. Biological aging processes underlying cognitive decline and neurodegenerative disease. Journal of clinical investigation, 132 (10). doi:10.1172/JCI158453.
- Grigoratos, T. and Martini, G., 2015. Brake wear particle emissions: a review. Environmental science and pollution research, 22 (4), 2491–2504. doi:10.1007/s11356-014-3696-8.
- Grodstein, F., et al., 2021. The association of epigenetic clocks in brain tissue with brain pathologies and common aging phenotypes. Neurobiology of disease, 157, 105428. doi:10.1016/j.nbd.2021.105428.
- Guillaumet-Adkins, A., et al., 2017. Epigenetics and oxidative stress in aging. Oxidative medicine and cellular longevity, 2017, 9175806. doi:10.1155/2017/9175806
- Hahad, O., et al., 2020. Ambient air pollution increases the risk of cerebrovascular and neuropsychiatric disorders through induction of inflammation and oxidative stress. International journal of molecular sciences, 21 (12), 4306. doi:10.3390/ijms21124306.
- Hajat, A., et al., 2021. Confounding by socioeconomic status in epidemiological studies of air pollution and health: challenges and opportunities. Environmental health perspectives, 129 (6), 65001. doi:10.1289/EHP7980.
- Halperin, D., 2014. Environmental noise and sleep disturbances: a threat to health? Sleep science, 7 (4), 209–212. doi:10.1016/j.slsci.2014.11.003.
- Hannah, C.-C., Eric, T., and Glen, E.D., 2015. Access to green space, physical activity and mental health: a twin study. Journal of epidemiology and community health, 69 (6), 523. doi:10.1136/jech-2014-204667.
- Hannon, E., et al., 2018. Characterizing genetic and environmental influences on variable DNA methylation using monozygotic and dizygotic twins. PLoS genetics, 14 (8), e1007544. doi:10.1371/journal.pgen.1007544.
- Hannum, G., et al., 2013. Genome-wide methylation profiles reveal quantitative views of human aging rates. Molecular cell, 49 (2), 359–367. doi:10.1016/j.molcel.2012.10.016.
- Hara, Y., et al., 2015. Estrogen effects on cognitive and synaptic health over the lifecourse. Physiological reviews, 95 (3), 785–807. doi:10.1152/physrev.00036.2014.
- Hardeland, R., 2012. Neurobiology, pathophysiology, and treatment of melatonin deficiency and dysfunction. Scientific world journal, 2012, 640389. doi:10.1100/2012/640389
- Hasan, M., et al., 2022. Urban green space mediates spatiotemporal variation in land surface temperature: a case study of an urbanized city, Bangladesh. Environmental science and pollution research international, 29 (24), 36376–36391. doi:10.1007/s11356-021-17480-9.
- He, C., et al., 2021. Whole-transcriptome analysis of aluminum-exposed rat hippocampus and identification of ceRNA networks to investigate neurotoxicity of Al. Molecular therapy - nucleic acids, 26, 1401–1417. doi:10.1016/j.omtn.2021.11.010.
- Heusinkveld, H.J., et al., 2016. Neurodegenerative and neurological disorders by small inhaled particles. NeuroToxicology, 56, 94–106. doi:10.1016/j.neuro.2016.07.007.
- Hing, B., et al., 2018. Chronic social stress induces DNA methylation changes at an evolutionary conserved intergenic region in chromosome X. Epigenetics, 13 (6), 627–641. doi:10.1080/15592294.2018.1486654.
- Ho, S.M., et al., 2012. Environmental epigenetics and its implication on disease risk and health outcomes. ILAR journal / national research council, institute of laboratory animal resources, 53 (3–4), 289–305. doi:10.1093/ilar.53.3-4.289.
- Holliday, K.M., et al., 2022. Gaseous air pollutants and DNA methylation in a methylome-wide association study of an ethnically and environmentally diverse population of U.S. adults. Environmental research, 212 (Pt C), 113360. doi:10.1016/j.envres.2022.113360.
- Holmström, K.M. and Finkel, T., 2014. Cellular mechanisms and physiological consequences of redox-dependent signalling. Nature reviews molecular cell biology, 15 (6), 411–421. doi:10.1038/nrm3801.
- Horvath, S., 2013. DNA methylation age of human tissues and cell types. Genome biology, 14 (10), 3156. doi:10.1186/gb-2013-14-10-r115.
- Horvath, S. and Raj, K., 2018. DNA methylation-based biomarkers and the epigenetic clock theory of ageing. Nature reviews genetics, 19 (6), 371–384. doi:10.1038/s41576-018-0004-3.
- Hou, Y., et al., 2019. Ageing as a risk factor for neurodegenerative disease. Nature reviews neurology, 15 (10), 565–581. doi:10.1038/s41582-019-0244-7.
- Huang, Y.T., Hong, F.F., and Yang, S.L., 2021. Atherosclerosis: the culprit and Co-victim of vascular dementia. Frontiers in neuroscience, 15, 673440. doi:10.3389/fnins.2021.673440
- Hudec, M., et al., 2020. Epigenetic regulation of circadian rhythm and its possible role in diabetes mellitus. International journal of molecular sciences, 21 (8), 3005. doi:10.3390/ijms21083005.
- Hui, L., 2017. Assessment of the role of ageing and non-ageing factors in death from non-communicable diseases based on a cumulative frequency model. Scientific reports, 7 (1), 8159. doi:10.1038/s41598-017-08539-0.
- Hunter, D.J., et al., 2019. Impact of aerobic exercise and fatty acid supplementation on global and gene-specific DNA methylation. Epigenetics, 14 (3), 294–309. doi:10.1080/15592294.2019.1582276.
- Hwang, J.Y., Aromolaran, K.A., and Zukin, R.S., 2017. The emerging field of epigenetics in neurodegeneration and neuroprotection. Nature reviews Neuroscience, 18 (6), 347–361. doi:10.1038/nrn.2017.46.
- Hystad, P., et al., 2019. Green space associations with mental health and cognitive function: results from the Quebec CARTaGENE cohort. Environmental Epidemiology, 3 (1), e040. (no pagination): doi:10.1097/EE9.0000000000000040.
- Iso-Markku, P., et al., 2022. Physical activity as a protective factor for dementia and Alzheimer’s disease: systematic review, meta-analysis and quality assessment of cohort and case-control studies. British journal of sports medicine, 56 (12), 701–709. doi:10.1136/bjsports-2021-104981.
- Iwińska, K., et al., 2018. Cycling in Warsaw, Poland – perceived enablers and barriers according to cyclists and non-cyclists. Transportation research part A: Policy and practice, 113, 291–301. doi:10.1016/j.tra.2018.04.014
- Jain, P., et al., 2022. Analysis of epigenetic age acceleration and healthy longevity among older US women. JAMA Network Open, 5 (7), e2223285. doi:10.1001/jamanetworkopen.2022.23285.
- Jennings, V. and Bamkole, O., 2019. The relationship between social cohesion and urban green space: an avenue for health promotion. International journal of environmental research and public health, 16 (3), 452. [Internet]: doi:10.3390/ijerph16030452.
- Jia, J., et al., 2023. Association between healthy lifestyle and memory decline in older adults: 10 year, population based, prospective cohort study. BMJ: British medical journal, 380, e072691. doi:10.1136/bmj-2022-072691.
- Jirtle, R.L. and Skinner, M.K., 2007. Environmental epigenomics and disease susceptibility. Nature reviews genetics, 8 (4), 253–262. doi:10.1038/nrg2045.
- Jo, S., et al., 2021. Association of NO2 and other air pollution exposures with the risk of Parkinson disease. JAMA neurology, 78 (7), 800–808. doi:10.1001/jamaneurol.2021.1335.
- Junior, D.P.M., Bueno, C., and da Silva, C.M., 2022. The effect of urban green spaces on reduction of particulate matter concentration. Bulletin of environmental contamination and toxicology, 108 (6), 1104–1110. doi:10.1007/s00128-022-03460-3.
- Ju, Y. and Tam, K.Y., 2022. Pathological mechanisms and therapeutic strategies for Alzheimer’s disease. Neural regeneration research, 17 (3), 543–549. doi:10.4103/1673-5374.320970.
- Kalia, V., et al., 2022. An exposomic framework to uncover environmental drivers of aging. Exposome, 2 (1). doi:10.1093/exposome/osac002.
- Kandlur, A., Satyamoorthy, K., and Gangadharan, G., 2020. Oxidative stress in cognitive and epigenetic aging: a retrospective glance. Frontiers in molecular neuroscience, 13, 41. doi:10.3389/fnmol.2020.00041
- Kanherkar, R.R., Bhatia-Dey, N., and Csoka, A.B., 2014. Epigenetics across the human lifespan. Frontiers in cell and developmental biology, 2, 49. doi:10.3389/fcell.2014.00049
- Kao, C.F., et al., 2020. Gene-based association analysis suggests association of HTR2A with antidepressant treatment response in depressed patients. Frontiers in pharmacology, 11, 559601. doi:10.3389/fphar.2020.559601.
- Katayama, O., et al., 2020. The association between neighborhood amenities and cognitive function: role of lifestyle activities. Journal of clinical medicine, 9 (7), 2109. doi:10.3390/jcm9072109.
- Kawahara, M. and Kato-Negishi, M., 2011. Link between aluminum and the pathogenesis of Alzheimer’s disease: the integration of the aluminum and amyloid cascade hypotheses. International journal of Alzheimer’s disease, 2011, 1–17. doi:10.4061/2011/276393
- Kempf, A., et al., 2019. A potassium channel β-subunit couples mitochondrial electron transport to sleep. Nature, 568 (7751), 230–234. doi:10.1038/s41586-019-1034-5.
- Killin, L.O.J., et al., 2016. Environmental risk factors for dementia: a systematic review. BMC geriatrics, 16 (1), 175. doi:10.1186/s12877-016-0342-y.
- Kim, K.-H., Kabir, E., and Kabir, S., 2015. A review on the human health impact of airborne particulate matter. Environment International, 74, 136–143. doi:10.1016/j.envint.2014.10.005
- Kim, E.J., Kim, J., and Kim, H., 2020. Neighborhood walkability and active transportation: a correlation study in leisure and shopping purposes. International journal of environmental research and public health, 17 (7), 2178. [Internet]: doi:10.3390/ijerph17072178.
- Knapskog, M., et al., 2019. Exploring ways of measuring walkability. Transportation research procedia, 41, 264–282. doi:10.1016/j.trpro.2019.09.047.
- Kular, L. and Jagodic, M., 2020. Epigenetic insights into multiple sclerosis disease progression. Journal of internal medicine, 288 (1), 82–102. doi:10.1111/joim.13045.
- Lafaille-Magnan, M.E., et al., 2017. Odor identification as a biomarker of preclinical AD in older adults at risk. Neurology, 89 (4), 327–335. doi:10.1212/WNL.0000000000004159.
- Lahiri, D.K. and Maloney, B., 2010. The “LEARn” (Latent Early-life Associated Regulation) model integrates environmental risk factors and the developmental basis of Alzheimer’s disease, and proposes remedial steps. Experimental gerontology, 45 (4), 291–296. doi:10.1016/j.exger.2010.01.001.
- Lahiri, D.K., Maloney, B., and Zawia, N.H., 2009. The LEARn model: an epigenetic explanation for idiopathic neurobiological diseases. Molecular Psychiatry, 14 (11), 992–1003. doi:10.1038/mp.2009.82.
- Landgrave-Gómez, J., Mercado-Gómez, O., and Guevara-Guzmán, R., 2015. Epigenetic mechanisms in neurological and neurodegenerative diseases. Frontiers in cellular neuroscience, 9, 58. doi:10.3389/fncel.2015.00058
- Le, T.N., et al., 2017. Current insights in noise-induced hearing loss: a literature review of the underlying mechanism, pathophysiology, asymmetry, and management options. Journal of otolaryngology-head & neck surgery, 46 (1), 41. doi:10.1186/s40463-017-0219-x.
- Lee, J., et al., 2023. Particulate matter exposure and neurodegenerative diseases: a comprehensive update on toxicity and mechanisms. Ecotoxicology and environmental safety, 266, 115565. doi:10.1016/j.ecoenv.2023.115565.
- Lee, M.K., et al., 2019. Genome-wide DNA methylation and long-term ambient air pollution exposure in Korean adults. Clinical epigenetics, 11 (1), 37. doi:10.1186/s13148-019-0635-z.
- Levine, M.E., et al., 2015. Epigenetic age of the pre-frontal cortex is associated with neuritic plaques, amyloid load, and Alzheimer’s disease related cognitive functioning. Aging (Albany NY), 7 (12), 1198–1211. doi:10.18632/aging.100864.
- Levine, M.E., et al., 2018. An epigenetic biomarker of aging for lifespan and healthspan. Aging (Albany NY), 10 (4), 573–591. doi:10.18632/aging.101414.
- Li, B., et al., 2021. Developmental angiogenesis requires the mitochondrial phenylalanyl-tRNA synthetase. Frontiers in cardiovascular medicine, 8, 724846. doi:10.3389/fcvm.2021.724846.
- Li, J., et al., 2023. Landscape fire smoke enhances the association between fine particulate matter exposure and acute respiratory infection among children under 5 years of age: findings of a case-crossover study for 48 low- and middle-income countries. Environment international, 171, 107665. doi:10.1016/j.envint.2022.107665.
- Li, A., Koch, Z., and Ideker, T., 2022. Epigenetic aging: biological age prediction and informing a mechanistic theory of aging. Journal of internal medicine, 292 (5), 733–744. doi:10.1111/joim.13533.
- Lin, F.C., et al., 2022. Air pollution is associated with cognitive deterioration of Alzheimer’s disease. Gerontology, 68 (1), 53–61. doi:10.1159/000515162.
- Li, Q.S., Sun, Y., and Wang, T., 2020. Epigenome-wide association study of Alzheimer’s disease replicates 22 differentially methylated positions and 30 differentially methylated regions. Clinical epigenetics, 12 (1), 149. doi:10.1186/s13148-020-00944-z.
- Liu, C.C., et al., 2019. Association of environmental features and the risk of Alzheimer’s dementia in older adults: a nationwide longitudinal case-control study. International journal of environmental research and public health, 16 (16), 2828. doi:10.3390/ijerph16162828.
- Liu, J., et al., 2017. Assessment of relationship on excess arsenic intake from drinking water and cognitive impairment in adults and elders in arsenicosis areas. International journal of hygiene and environmental health, 220 (2 Pt B), 424–430. doi:10.1016/j.ijheh.2016.12.004.
- Liu, R.M., 2022. Aging, cellular senescence, and Alzheimer’s disease. International journal of molecular sciences, 23 (4), 1989. doi:10.3390/ijms23041989.
- Liu, Z., et al., 2020. Underlying features of epigenetic aging clocks in vivo and in vitro. Aging cell, 19 (10), e13229. doi:10.1111/acel.13229.
- Livingston, G., et al., 2020. Dementia prevention, intervention, and care: 2020 report of the lancet commission. The lancet, 396 (10248), 413–446. doi:10.1016/S0140-6736(20)30367-6.
- Lu, A.T., et al., 2019. DNA methylation GrimAge strongly predicts lifespan and healthspan. Aging (Albany NY), 11 (2), 303–327. doi:10.18632/aging.101684.
- Lu, A.T., et al., 2023. Universal DNA methylation age across mammalian tissues. Nature aging, 3 (9), 1144–1166. doi:10.1038/s43587-023-00462-6.
- Lupolt, S.N., et al., 2022. Key considerations for assessing soil ingestion exposures among agricultural workers. Journal of exposure science & environmental epidemiology, 32 (3), 481–492. doi:10.1038/s41370-021-00339-z.
- Maas, J., et al., 2009. Social contacts as a possible mechanism behind the relation between green space and health. Health & place, 15 (2), 586–595. doi:10.1016/j.healthplace.2008.09.006.
- Maasar, M.F., et al., 2021. The comparative methylome and transcriptome after change of direction compared to straight line running exercise in human skeletal muscle. Frontiers in physiology, 12, 619447. doi:10.3389/fphys.2021.619447.
- Maher, B.A., et al., 2016. Magnetite pollution nanoparticles in the human brain. Proceedings of the national academy of sciences, 113 (39), 10797–10801. doi:10.1073/pnas.1605941113.
- Maitre, L., et al., 2022. Multi-omics signatures of the human early life exposome. Nature communications, 13 (1), 7024. doi:10.1038/s41467-022-34422-2.
- Marin, C., et al., 2018. Olfactory dysfunction in neurodegenerative diseases. Current allergy and asthma reports, 18 (8), 42. doi:10.1007/s11882-018-0796-4.
- Marini, M., et al., 2021. Daily intake of heavy metals and minerals in food – a case study of four Danish dietary profiles. Journal of cleaner production, 280, 124279. doi:10.1016/j.jclepro.2020.124279.
- Mazzoleni, E., et al., 2023. Outdoor artificial light at night and risk of early-onset dementia: a case-control study in the Modena population, Northern Italy. Heliyon, 9 (7), e17837. doi:10.1016/j.heliyon.2023.e17837.
- McCrory, C., et al., 2021. GrimAge outperforms other epigenetic clocks in the prediction of age-related clinical phenotypes and all-cause mortality. Journals of gerontology: series A, 76 (5), 741–749. doi:10.1093/gerona/glaa286.
- McGowan, P.O. and Roth, T.L., 2015. Epigenetic pathways through which experiences become linked with biology. Development & psychopathology, 27 (2), 637–648. doi:10.1017/S0954579415000206.
- Meng, L., et al., 2022. Chronic noise exposure and risk of dementia: a systematic review and dose-response meta-analysis. Frontiers in public health, 10, 832881. doi:10.3389/fpubh.2022.832881.
- Meng, Y., Kelly, F.J., and Wright, S.L., 2020. Advances and challenges of microplastic pollution in freshwater ecosystems: a UK perspective. Environmental pollution, 256, 113445. doi:10.1016/j.envpol.2019.113445
- Mielke, M.M., Vemuri, P., and Rocca, W.A., 2014. Clinical epidemiology of Alzheimer’s disease: assessing sex and gender differences. Clinical epidemiology, 6, 37–48. doi:10.2147/CLEP.S37929
- Millan, M.J., 2014. The epigenetic dimension of Alzheimer’s disease: causal, consequence, or curiosity? Dialogues in clinical neuroscience, 16 (3), 373–393. doi:10.31887/DCNS.2014.16.3/mmillan.
- Millán-Zambrano, G., et al., 2022. Histone post-translational modifications — cause and consequence of genome function. Nature reviews genetics, 23 (9), 563–580. doi:10.1038/s41576-022-00468-7.
- Morgensztern, D. et al., 2018. Mutational events in lung cancer: present and developing technologies. In: H.I. Pass, D. Ball, and G.V. Scagliotti, eds. IASLC thoracic oncology 2nd ed. Philadelphia: Elsevier, 95–103.e2.
- Morley, J.F., et al., 2018. Optimizing olfactory testing for the diagnosis of Parkinson’s disease: item analysis of the university of Pennsylvania smell identification test. Npj Parkinson’s Disease, 4 (1), 2. doi:10.1038/s41531-017-0039-8.
- Mostafavi, N., et al., 2018. Acute changes in DNA methylation in relation to 24 h personal air pollution exposure measurements: a panel study in four European countries. Environment international, 120, 11–21. doi:10.1016/j.envint.2018.07.026.
- Mueller, B., Figueroa, A., and Robinson-Papp, J., 2022. Structural and functional connections between the autonomic nervous system, hypothalamic–pituitary–adrenal axis, and the immune system: a context and time dependent stress response network. Neurological sciences, 43 (2), 951–960. doi:10.1007/s10072-021-05810-1.
- Müller, A., et al., 2020. The pollution conveyed by urban runoff: a review of sources. Science of the total environment, 709, 136125. doi:10.1016/j.scitotenv.2019.136125
- Münzel, T., et al., 2022. Soil and water pollution and human health: what should cardiologists worry about? Cardiovascular research, 119 (2), 440–449. doi:10.1093/cvr/cvac082.
- Murata, H., Barnhill, L.M., and Bronstein, J.M., 2022. Air pollution and the risk of Parkinson’s disease: a review. Movement disorders: official journal of the movement disorder society, 37 (5), 894–904. doi:10.1002/mds.28922.
- Nichols, E., 2022. Estimation of the global prevalence of dementia in 2019 and forecasted prevalence in 2050: an analysis for the global burden of disease study 2019. Lancet public health, 7 (2), e105–e125. doi:10.1016/S2468-2667(21)00249-8.
- Noroozi, R., et al., 2021. DNA methylation-based age clocks: from age prediction to age reversion. Aging research reviews, 68, 101314. doi:10.1016/j.arr.2021.101314.
- Nous, A., Engelborghs, S., and Smolders, I., 2021. Melatonin levels in the Alzheimer’s disease continuum: a systematic review. Alzheimer’s research & therapy, 13 (1), 52. doi:10.1186/s13195-021-00788-6.
- Oppenheim, H.A., et al., 2013. Exposure to vehicle emissions results in altered blood brain barrier permeability and expression of matrix metalloproteinases and tight junction proteins in mice. Particle and fibre toxicology, 10 (1), 62. doi:10.1186/1743-8977-10-62.
- Pan, X., et al., 2022a. Associations among drinking water quality, dyslipidemia, and cognitive function for older adults in China: evidence from CHARLS. BMC Geriatrics, 22 (1). doi:10.1186/s12877-022-03375-y.
- Pan, X., et al., 2022b. Associations among drinking water quality, dyslipidemia, and cognitive function for older adults in China: evidence from CHARLS. BMC geriatrics, 22 (1), 683. doi:10.1186/s12877-022-03375-y.
- Pandics, T., et al., 2023. Exposome and unhealthy aging: environmental drivers from air pollution to occupational exposures. GeroScience, 45 (6), 3381–3408. doi:10.1007/s11357-023-00913-3.
- Panes, J.D., et al., 2022. Deciphering the role of PGC-1α in neurological disorders: from mitochondrial dysfunction to synaptic failure. Neural regeneration research, 17 (2), 237–245. doi:10.4103/1673-5374.317957.
- Pankhurst, Q., et al., 2008. Increased levels of magnetic iron compounds in Alzheimer’s disease. Journal of Alzheimer’s disease: JAD, 13 (1), 49–52. doi:10.3233/JAD-2008-13105.
- Panni, S., et al., 2020. Non-coding RNA regulatory networks. Biochimica et biophysica acta (BBA)-Gene regulatory mechanisms, 1863 (6), 194417. doi:10.1016/j.bbagrm.2019.194417.
- Parade, S.H., et al., 2017. Stress exposure and psychopathology alter methylation of the serotonin receptor 2A (HTR2A) gene in preschoolers. Development & psychopathology, 29 (5), 1619–1626. doi:10.1017/S0954579417001274.
- Patel, P.C., 2019. Light pollution and insufficient sleep: evidence from the United States. American journal of human biology, 31 (6), e23300. doi:10.1002/ajhb.23300.
- Paul, D.S. and Beck, S., 2014. Advances in epigenome-wide association studies for common diseases. Trends in molecular medicine, 20 (10), 541–543. doi:10.1016/j.molmed.2014.07.002.
- Peters, R., et al., 2019. Air pollution and dementia: a systematic review. Journal of Alzheimer’s disease: JAD, 70 (s1), S145–s63. doi:10.3233/JAD-180631.
- Pierce, B.L. and Burgess, S., 2013. Efficient design for Mendelian randomization studies: subsample and 2-sample instrumental variable estimators. American journal of epidemiology, 178 (7), 1177–1184. doi:10.1093/aje/kwt084.
- Pirooznia, S.K., et al., 2012. Epigenetic regulation of axonal growth of drosophila pacemaker cells by histone acetyltransferase tip60 controls sleep. Genetics, 192 (4), 1327–1345. doi:10.1534/genetics.112.144667.
- Plaza-Diaz, J., et al., 2022. Impact of physical activity and exercise on the epigenome in skeletal muscle and effects on systemic metabolism. Biomedicines, 10 (1), 126. doi:10.3390/biomedicines10010126.
- Poprac, P., et al., 2017. Targeting free radicals in oxidative stress-related human diseases. Trends in pharmacological sciences, 38 (7), 592–607. doi:10.1016/j.tips.2017.04.005.
- Rakyan, V.K., et al., 2011. Epigenome-wide association studies for common human diseases. Nature reviews genetics, 12 (8), 529–541. doi:10.1038/nrg3000.
- Rao, P., Belanger, M.J., and Robbins, J.M., 2022. Exercise, physical activity, and cardiometabolic health: insights into the prevention and treatment of cardiometabolic diseases. Cardiology in review, 30 (4), 167–178. doi:10.1097/CRD.0000000000000416.
- Rasmussen, M., Zierath, J.R., and Barrès, R., 2014. Dynamic epigenetic responses to muscle contraction. Drug discovery today, 19 (7), 1010–1014. doi:10.1016/j.drudis.2014.03.003.
- Relton, C. and Smith, G., 2015. Mendelian randomization: applications and limitations in epigenetic studies. Epigenomics, 7 (8), 1239–1243. doi:10.2217/epi.15.88.
- Rey, R., et al., 2019. Distinct expression pattern of epigenetic machinery genes in blood leucocytes and brain cortex of depressive patients. Molecular neurobiology, 56 (7), 4697–4707. doi:10.1007/s12035-018-1406-0.
- Richardson, E.A., et al., 2013. Role of physical activity in the relationship between urban green space and health. Public health, 127 (4), 318–324. doi:10.1016/j.puhe.2013.01.004.
- Rodriguez-Loureiro, L., et al., 2022. Long-term exposure to residential greenness and neurodegenerative disease mortality among older adults: a 13-year follow-up cohort study. Environmental health, 21 (1). doi:10.1186/s12940-022-00863-x.
- Roe, J.J., et al., 2013. Green space and stress: evidence from cortisol measures in deprived urban communities. International journal of environmental research and public health, 10 (9), 4086–4103. Internet. doi:10.3390/ijerph10094086.
- Ryu, H.W., et al., 2015. Influence of toxicologically relevant metals on human epigenetic regulation. Toxicological research, 31 (1), 1–9. doi:10.5487/TR.2015.31.1.001.
- Ryu, Y.S., et al., 2019. Particulate matter-induced senescence of skin keratinocytes involves oxidative stress-dependent epigenetic modifications. Experimental & molecular medicine, 51 (9), 1–14. doi:10.1038/s12276-019-0305-4.
- Schirmer, L., et al., 2019. Neuronal vulnerability and multilineage diversity in multiple sclerosis. Nature, 573 (7772), 75–82. doi:10.1038/s41586-019-1404-z.
- Scorza, F. and Fortunato, G., 2021. Cyclable cities: building feasible scenario through urban space morphology assessment. Journal of urban planning and development, 147 (4). doi:10.1061/(ASCE)UP.1943-5444.0000713.
- Sharma, V.K., Mehta, V., and Singh, T.G., 2020. Alzheimer’s disorder: epigenetic connection and associated risk factors. Current neuropharmacology, 18 (8), 740–753. doi:10.2174/1570159X18666200128125641.
- Shen, X.L., et al., 2014. Positive relationship between mortality from Alzheimer’s disease and soil metal concentration in mainland China. Journal of Alzheimer’s disease: JAD, 42 (3), 893–900. doi:10.3233/JAD-140153.
- Shireby, G.L., et al., 2020. Recalibrating the epigenetic clock: implications for assessing biological age in the human cortex. Brain A journal of neurology, 143 (12), 3763–3775. doi:10.1093/brain/awaa334.
- Shukla, M., et al., 2017. Mechanisms of melatonin in alleviating Alzheimer’s disease. Current neuropharmacology, 15 (7), 1010–1031. doi:10.2174/1570159X15666170313123454.
- Skinner, M.K., 2011. Role of epigenetics in developmental biology and transgenerational inheritance. Birth defects research Part C, embryo today: Reviews, 93 (1), 51–55. doi:10.1002/bdrc.20199.
- Slawsky, E.D., et al., 2022. Neighborhood greenspace exposure as a protective factor in dementia risk among U.S. adults 75 years or older: a cohort study. Environmental health: a global access science source, 21 (1), (no pagination). doi:10.1186/s12940-022-00830-6.
- Smith, R.G., et al., 2021. A meta-analysis of epigenome-wide association studies in Alzheimer’s disease highlights novel differentially methylated loci across cortex. Nature Communications, 12 (1), 3517. doi:10.1038/s41467-021-23243-4.
- Smith, G.D. and Ebrahim, S., 2003. ‘Mendelian randomization’: can genetic epidemiology contribute to understanding environmental determinants of disease? International journal of epidemiology, 32 (1), 1–22. doi:10.1093/ije/dyg070.
- Stefanova, N.A., et al., 2015. Melatonin attenuates impairments of structural hippocampal neuroplasticity in OXYS rats during active progression of Alzheimer’s disease-like pathology. Journal of pineal research, 59 (2), 163–177. doi:10.1111/jpi.12248.
- Su, D., et al., 2018. Chronic noise exposure exacerbates AD-like neuropathology in SAMP8 mice in relation to wnt signaling in the PFC and hippocampus. Scientific reports, 8 (1), 14622. doi:10.1038/s41598-018-32948-4.
- Sumien, N., et al., 2021. Neurodegenerative disease: roles for sex, hormones, and oxidative stress. Endocrinology, 162 (11). doi:10.1210/endocr/bqab185.
- Suresh, S., Singh, S.A., and Vellapandian, C., 2022. Bisphenol a exposure links to exacerbation of memory and cognitive impairment: a systematic review of the literature. Neuroscience & biobehavioral reviews, 143, 104939. doi:10.1016/j.neubiorev.2022.104939
- Światowy, W.J., et al., 2021. Physical activity and DNA methylation in humans. International journal of molecular sciences, 22 (23), 12989. doi:10.3390/ijms222312989.
- Sylvers, D.L., et al., 2022. Walkable neighborhoods and cognition: implications for the design of health promoting communities. Journal of aging and health, 34 (6–8), 893–904. doi:10.1177/08982643221075509.
- Tai, X.Y., et al., 2022. Cardiometabolic multimorbidity, genetic risk, and dementia: a prospective cohort study. Lancet healthy longevity, 3 (6), e428–e36. doi:10.1016/S2666-7568(22)00117-9.
- Takasugi, T., et al., 2021. Community-level educational attainment and dementia: a 6-year longitudinal multilevel study in Japan. BMC Geriatrics, 21 (1), 661. doi:10.1186/s12877-021-02615-x.
- Takiguchi, M., et al., 2003. Effects of cadmium on DNA-(cytosine-5) methyltransferase activity and DNA methylation status during cadmium-induced cellular transformation. Experimental cell research, 286 (2), 355–365. doi:10.1016/S0014-4827(03)00062-4.
- Tang, B.L., 2020. Neuropathological mechanisms associated with pesticides in Alzheimer’s disease. Toxics, 8 (2), 21. doi:10.3390/toxics8020021.
- Tang, X., et al., 2022. Epigenetic clock acceleration is linked to age at onset of Parkinson’s disease. Movement disorders: official journal of the movement disorder society, 37 (9), 1831–1840. doi:10.1002/mds.29157.
- Terada, T., et al., 2020. In vivo mitochondrial and glycolytic impairments in patients with Alzheimer disease. Neurology, 94 (15), e1592–e604. doi:10.1212/WNL.0000000000009249.
- Thanassoulis, G. and O’Donnell, C.J., 2009. Mendelian randomization: nature’s randomized trial in the post-genome era. Jama, 3012009 (22), 2386–2388. doi:10.1001/jama.2009.812.
- Thompson, R.C., et al., 2009. Plastics, the environment and human health: current consensus and future trends. Philosophical transactions of the royal society B: Biological sciences, 364 (1526), 2153–2166. doi:10.1098/rstb.2009.0053.
- Timmermans, E.J., et al., 2023. Neighbourhood walkability in relation to cognitive functioning in patients with disorders along the heart-brain axis. Health & place, 79, 102956. doi:10.1016/j.healthplace.2022.102956.
- Umberson, D. and Karas Montez, J., 2010. Social relationships and health: a flashpoint for health policy. Journal of health and social behavior, 51 (1_suppl), S54–S66. doi:10.1177/0022146510383501.
- United Nations Population Division, 2019. World urbanization prospects: 2018 revision. doi:10.18356/b9e995fe-en.
- Valavanidis, A., Fiotakis, K., and Vlachogianni, T., 2008. Airborne particulate matter and human health: toxicological assessment and importance of size and composition of particles for oxidative damage and carcinogenic mechanisms. Journal of environmental science and health, part C, 26 (4), 339–362. doi:10.1080/10590500802494538.
- Villafuerte, G., et al., 2015. Sleep deprivation and oxidative stress in animal models: a systematic review. Oxidative medicine and cellular longevity, 2015, 234952. doi:10.1155/2015/234952
- Walker, V.M., et al., 2019. Using the MR-Base platform to investigate risk factors and drug targets for thousands of phenotypes. Wellcome open research, 4, 113. doi:10.12688/wellcomeopenres.15334.1.
- Walker, W.H., et al., 2020. Circadian rhythm disruption and mental health. Translational psychiatry, 10 (1), 28. doi:10.1038/s41398-020-0694-0.
- Wang, C., et al., 2020. Associations of annual ambient PM(2.5) components with DNAm PhenoAge acceleration in elderly men: the normative aging study. Environmental pollution, 258, 113690. doi:10.1016/j.envpol.2019.113690
- Wang, K., et al., 2022. Epigenetic regulation of aging: implications for interventions of aging and diseases. Signal transduction and targeted therapy, 7 (1), 374. doi:10.1038/s41392-022-01211-8.
- Wang, R.Z., et al., 2021. Genetically determined low income modifies Alzheimer’s disease risk. Annals of translational medicine, 9 (15), 1222. doi:10.21037/atm-21-344.
- Wang, Y., et al., 2020. Independent effect of main components in particulate matter on DNA methylation and DNA methyltransferase: a molecular epidemiology study. Environment international, 134, 105296. doi:10.1016/j.envint.2019.105296.
- Wang, Z., et al., 2016. Chronic exposure to aluminum and risk of Alzheimer’s disease: a meta-analysis. Neuroscience letters, 610, 200–206. doi:10.1016/j.neulet.2015.11.014.
- Warraich, U.E., Hussain, F., and Kayani, H.U.R., 2020. Aging - oxidative stress, antioxidants and computational modeling. Heliyon, 6 (5), e04107. doi:10.1016/j.heliyon.2020.e04107.
- Wei, J., et al., 2021. DNA methyltransferase 3A is involved in the sustained effects of chronic stress on synaptic functions and behaviors. Cerebral cortex (New York, NY: 1991), 31 (4), 1998–2012. doi:10.1093/cercor/bhaa337.
- White, A.J., et al., 2019. Air pollution, particulate matter composition and methylation-based biologic age. Environment international, 132, 105071. doi:10.1016/j.envint.2019.105071.
- White, L., et al., 2020. Dementia is associated with earlier mortality for men and women in the United States. Gerontology and geriatric medicine, 6, 2333721420945922. doi:10.1177/2333721420945922
- White, M.P., et al., 2020. Blue space, health and well-being: a narrative overview and synthesis of potential benefits. Environmental research, 191, 110169. doi:10.1016/j.envres.2020.110169.
- Wiegand, A., et al., 2021. DNA methylation differences associated with social anxiety disorder and early life adversity. Translational psychiatry, 11 (1), 104. doi:10.1038/s41398-021-01225-w.
- Williams, M.E., et al., 2023. Higher cortical thickness/volume in Alzheimer’s-related regions: protective factor or risk factor? Neurobiology of aging, 129, 185–194. doi:10.1016/j.neurobiolaging.2023.05.004.
- Wilson, J. and Xiao, X., 2023. The economic value of health benefits associated with urban park investment. International journal of environmental research and public health, 20 (6), 4815. doi:10.3390/ijerph20064815.
- Wittenberg, R., et al., 2020. Projections of care for older people with dementia in England: 2015 to 2040. Age and ageing, 49 (2), 264–269. doi:10.1093/ageing/afz154.
- Wittfeld, K., et al., 2020. Cardiorespiratory fitness and gray matter volume in the temporal, frontal, and cerebellar regions in the general population. Mayo clinic proceedings, 95 (1), 44–56. doi:10.1016/j.mayocp.2019.05.030.
- World Health Organisation, 2013. Health effects of particulate matter: policy implications for countries in eastern Europe. Caucasus and central Asia.
- World Health Organisation, 2021. Urban health. Available from: https://www.who.int/news-room/fact-sheets/detail/urban-health.
- World Health Organisation, 2021. WHO global air quality guidelines: Particulate matter (PM2.5 and PM10), ozone, nitrogen dioxide, sulfur dioxide and carbon monoxide.
- Wu, J., et al., 2022. Air pollution as a risk factor for Cognitive Impairment No Dementia (CIND) and its progression to dementia: a longitudinal study. Environment international, 160, 107067. doi:10.1016/j.envint.2021.107067.
- Wu, J.W., et al., 2021. Biological age in healthy elderly predicts aging-related diseases including dementia. Scientific reports, 11 (1), 15929. doi:10.1038/s41598-021-95425-5.
- Wu, Y.T., et al., 2020. Neighbourhood environment and dementia in older people from high-, middle- and low-income countries: results from two population-based cohort studies. Bmc public health, 20 (1). doi:10.1186/s12889-020-09435-5.
- Wu, Z., et al., 2021. Gray matter deterioration pattern during Alzheimer’s disease progression: a regions-of-interest based surface morphometry study. Frontiers in aging neuroscience, 13, 593898. doi:10.3389/fnagi.2021.593898.
- Wu, H., Eckhardt, C.M., and Baccarelli, A.A., 2023. Molecular mechanisms of environmental exposures and human disease. Nature reviews genetics, 24 (5), 332–344. doi:10.1038/s41576-022-00569-3.
- Xu, R., et al., 2021. Residential surrounding greenness and DNA methylation: an epigenome-wide association study. Environment international, 154, 106556. doi:10.1016/j.envint.2021.106556.
- Xu, S., Akioma, M., and Yuan, Z., 2021. Relationship between circadian rhythm and brain cognitive functions. Frontiers of optoelectronics, 14 (3), 278–287. doi:10.1007/s12200-021-1090-y.
- Yang, N. and Sen, P., 2018. The senescent cell epigenome. Aging (Albany NY), 10 (11), 3590–3609. doi:10.18632/aging.101617.
- Yannatos, I. et al., 2022. Contributions of neighborhood social environment and air pollution exposure to black-white disparities in epigenetic aging. PLoS One, 18 (7), e0287112. doi:10.1371/journal.pone.0287112.
- Yu, X., et al., 2020. Exposure to air pollution and cognitive impairment risk: a meta-analysis of longitudinal cohort studies with dose-response analysis. Journal of global health, 10 (1), 010417. doi:10.7189/jogh.10.010417.
- Zeng, B., Liu, G., and Huang, J., 2022. DNA methylation and histone modification in dental-derived mesenchymal stem cells. Stem cell reviews & reports, 18 (8), 2797–2816. doi:10.1007/s12015-022-10413-0.
- Zhang, L., et al., 2022a. Targeting epigenetics as a promising therapeutic strategy for treatment of neurodegenerative diseases. Biochemical pharmacology, 206, 115295. doi:10.1016/j.bcp.2022.115295.
- Zhang, L., et al., 2022b. Green space, air pollution, weather, and cognitive function in middle and old age in China. Frontiers in public health, 10. doi:10.3389/fpubh.2022.871104.
- Zhang, X., et al., 2020. Effects of green space on walking: does size, shape and density matter? Urban studies, 57 (16), 3402–3420. doi:10.1177/0042098020902739.
- Zhao, Y., et al., 2014. Aluminum-induced amyloidogenesis and impairment in the clearance of amyloid peptides from the central nervous system in Alzheimer’s disease. Frontiers in neurology, 5, 167. doi:10.3389/fneur.2014.00167
- Zheng, J., et al., 2017. Recent developments in Mendelian randomization studies. Current epidemiology reports, 4 (4), 330–345. doi:10.1007/s40471-017-0128-6.
- Zhou, A., et al., 2022. Epigenetic aging as a biomarker of dementia and related outcomes: a systematic review. Epigenomics, 14 (18), 1125–1138. doi:10.2217/epi-2022-0209.
- Zhou, C.C., et al., 2018. Lead exposure induces Alzheimer's disease (AD)-like pathology and disturbes cholesterol metabolism in the young rat brain. Toxicology letters, 296, 173–183. doi:10.1016/j.toxlet.2018.06.1065.
- Zhou, X., et al., 2022. Alcohol consumption, DNA methylation and colorectal cancer risk: results from pooled cohort studies and Mendelian randomization analysis. International journal of cancer, 151 (1), 83–94. doi:10.1002/ijc.33945.
- Zukowska, J., et al., 2022. Which transport policies increase physical activity of the whole of society? A systematic review. Journal of transport & health, 27, 101488. doi:10.1016/j.jth.2022.101488.
- Zuniga-Teran, A.A., et al., 2019. Exploring the influence of neighborhood walkability on the frequency of use of greenspace. Landscape and urban planning, 190, 103609. doi:10.1016/j.landurbplan.2019.103609.