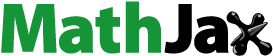
ABSTRACT
The surface shot peening treatment was carried out on the as-cast SiCw/Al composites, and the distribution of the residual stresses in the surface matrix was measured, the X-ray diffraction method for the yield strength of the surface matrix of the composites was established to explore the strengthening effect of the surface shot peening. The results show that the surface matrix of the composites after shot peening shows a large compressive residual stress state, and the FWHM, that is, the microstructure strengthening effect, is significantly increased., resulting in an increase in the yield strength of the surface matrix of the composites.
Introduction
Metal matrix composites have the advantages of high specific strength, specific stiffness and low thermal expansion coefficient, and can be widely used in many industrial fields [Citation1–4]. After years of development and research, metal matrix composites have been in the engineering application stage. As we all know, shot peening is a simple method of surface strengthening of metal materials, which can effectively improve the fatigue properties of materials [Citation5–7]. However, there are few reports about the application of shot peening technology on the surface of composite materials. The study of surface peening of composite materials is helpful to explore the potential properties of the materials, and has certain engineering application value. Practice has proved [Citation8–10] that X-ray diffraction technology can measure the stress state of the matrix in the composite material, and is an effective means to study the mechanical properties and microstructure of the composite material matrix. Many scholars have studied the influence of shot peening treatment on the residual stress and properties of aluminum alloy, among which Al-MG-Si alloy has received wide attention because of its good comprehensive properties. Alhamdany, A.A et [Citation11] Al found that due to the introduction of residual stress, shot peening treatment has a positive effect on the fatigue strength and life of 6061 alloy, and the fatigue strength can be increased to 182 MPa; Gonzalez, J et al. [Citation12] found that shot peening could refine the surface grains of 6063 alloy to 2–4 μm, improving the fatigue properties of the material by about 28%, but increasing the surface roughness to a certain extent. At present, there are few studies on the effect of shot peening treatment on SiCw /Al-Mg-Si composites. To this end, the residual stress distribution and surface yield strength of 20% SiCw / 6A02Al composite before and after shot peening were measured by X-ray stress meter in this work, and the surface strengthening effect of the composite was quantitatively revealed.
Test materials and methods
In this study, the surface shot peening of 6A02-20vol.%-SiCw aluminium alloy metal matrix composite was investigated. The matrix alloy of the SiCw/Al aluminum matrix composite material is 6A02 aluminum alloy. The primary alloying elements of 6A02 aluminum alloy are magnesium and silicon, which form the Mg2Si phase. The chemical composition of 6A02 aluminum alloy is provided in . The reinforcement in the composite is β-SiC whisker reinforcement. The main physical properties of the reinforced β-SiC are as follows: density ρ = 3.18 g/cm3, elastic modulus E = 482 GPa, tensile strength σb = 12.9∼13.7 GPa, melting point 2316°C. The material preparation includes the process of whisker preform forming and die-casting composite materials, the pouring temperature of liquid aluminum is 800°C, and the solidification temperature is about 600°C. The whisker length of the composite is 5 ∼ 15 μm, and the whisker diameter is 0.1 ∼ 1 μm. The treatment states of composite samples are the original die casting state and shot peening state. The peening process parameters are as follows: spherical glass projectile diameter 0.15 ∼ 0.25 mm, peening intensity (arc height) 0.2 mm, peening time 5 min.
Table 1. Chemical composition of 6A02 Al matrix.
The geometric relationship between the specimen size of the composite material and the measurement of X-ray stress is shown in , where the Y axis is the tensile direction. The composite was stripped layer by layer with 20% NaOH solution, and the residual stress and bearing stress in the matrix were measured by X-ray stress meter. The stress measurement parameters are as follows: tube voltage 28 kV, tube current 8 mA, Cr-Kα radiation, diffraction surface Al (222), collimated tube optical bar 2 mm, step scanning speed 3°/min, time constant 1s, ψ Angle 0° ∼ 45°, Φ Angle 0° and 90°, and the peak fixing method of the cross correlation function is adopted.
Figure 1. Dimensions (mm) of specimen of the composite and geometric relation in the X-ray stress measurement.
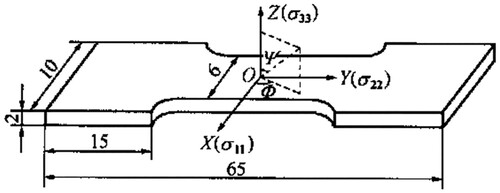
According to the principle of X-ray stress measurement [Citation13], the stress in the X and Y directions in can be expressed as:
(1)
(1)
(2)
(2) where, 2θ is the diffraction Angle of the composite matrix; K is the matrix X-ray stress constant, −92 MPa/(°).
When unidirectional tensile load is applied to the specimen, the composite matrix is not subjected to unidirectional stress, and the Mises equivalent bearing stress is the key factor determining the plastic strain of the matrix [Citation13,Citation14]. For the case of plane stress, the equivalent stress can be expressed as:
(3)
(3) Combining equations (1)–(3), the equivalent stress can be expressed as:
(4)
(4) When the sample is not loaded, the residual stress in the composite matrix can be measured according to formulas 1 and 2. When the sample is loaded, the equivalent bearing stress in the composite matrix is measured by formula 4.
Results and discussion
shows that no matter in the original cast state or shot peening state, there is a good linear relationship between 2θ and sin2ψ of the composite material matrix, indicating that there is no obvious texture or preferred orientation in the matrix of the two composite materials [Citation15]. Therefore, it is ideal to choose the X-ray stress measurement method, and the instrument has a high measurement accuracy, which can ensure the reliability of the measurement results of residual stress and equivalent bearing stress of composite matrix.
As can be seen in , there is a certain residual tensile stress in the original cast composite matrix. Since the macroscopic residual stress in the cast composite is negligible, the measured stress is actually the thermal mismatch stress caused by the difference in the coefficient of thermal expansion between the matrix and the whisker in the composite [Citation16,Citation17]. The thermal mismatch tensile stress in the matrix will reduce the tensile mechanical properties of the composite. The figure also shows that after shot peening, the surface matrix of the composite material exhibits a large residual compressive stress. The residual compressive stress of the surface matrix can improve the surface properties of the composite.
Figure 3. Residual stress (σres) in matrix vs depth (d) from surface of the 20%SiCw/6A02Al composite.
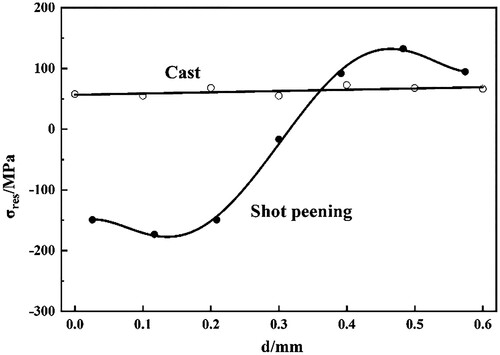
depicts the hardness distribution of SiCw/Al composite along the layer depth before and after shot peening. It is evident from the figure that shot peening significantly enhances the surface microhardness of the SiCw/Al composite, resulting in a 37% increase compared to the original sample. Additionally, the microhardness decreases with increasing layer depth. The impact of shot peening on microhardness is observed to extend to approximately 400 μm.
After a large number of projectiles impact the material's surface at high speed, significant changes occur in the surface topography of the material. The most direct characterization of this change is surface roughness. Following shot peening, the roughness of the SiCw/Al composite material increases from 0.31 μm to 1.66 μm.
shows the near-surface distribution of the half value breadth of X-ray diffraction lines in the matrix of composite materials. The broadening of the diffraction peak on the XRD pattern is related to the microstructure and microscopic stress in the material. The change of the half-height width (FWHM) of the diffraction peak is commonly used to comprehensively characterize the change in the structure of the material [Citation18,Citation19], and also to characterize the softening or hardening behavior of the material [Citation20,Citation21]. Compared with the curves in the figure, it can be found that the half value breadth (HVB) of XRD peaks of the surface matrix of the composite material after shot peening is obviously larger than that of the original cast sample, indicating that the shot peening can cause obvious subgrain refinement and lattice distortion of the surface matrix of the sample, and increase the dislocation density. In addition, the half-height and width of the diffraction peak of the shot peening layer decrease gradually with the increase of the depth of the shot peening layer, and the microstructure distortion and the grain size thinning caused by shot peening are significantly reduced. Through XRD linear analysis, the diffraction peaks can be deconvolution broadened to extract the structure information more carefully, and the statistical values of the internal crystal blocks and microscopic distortion can be obtained by calculation.
Figure 5. Half value breadth (HVB) of matrix for X-ray diffraction vs depth (d) from surface of the 20%SiCw/6A02Al composite.
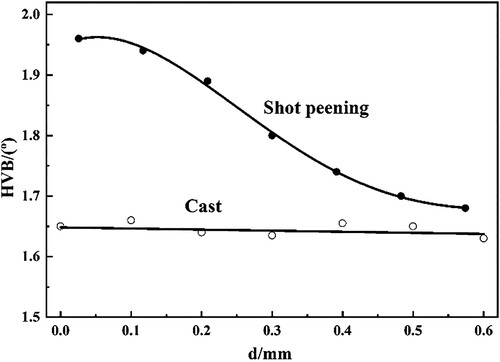
The main factors that lead to the broadening of X-ray diffraction peaks are the reduction of domain size, large amount of microscopic distortion and dislocation caused by pellets. Generally speaking, the size of the crystal block mainly depends on the thickness of the coherent scattering domain in the X-ray diffraction direction, and there is a certain difference from the grain size photographed by transmission and scanning equipment. At present, single-peak fitting is more commonly used by Vogit function approximation method. In this method, the broadening peak shape of the X-ray diffraction peak can be divided into two parts: one part is the broadening caused by the reduction of the domain size, and the diffraction line shape after the broadening accords with the Cauchy function distribution; the other part is the broadening caused by the increase of crystal microstrain. The X-ray diffraction curves caused by broadening conform to the Gaussian function distribution. The domain size and microstrain corresponding to the diffraction peak can be obtained by formulas (5) and (6).
(5)
(5)
(6)
(6) Where β is the integral width of the diffraction line, λ is the incoming wavelength, θ is the diffraction Angle, D is the domain size, and k is a constant (0.89 or 0.94). If the domain size of the shot peening layer is too large or there is only microstrain in the crystal, then the microstrain in the crystal can also be obtained by (6).
By Voigt linear analysis, the distribution of domain size with layer depth of SiCw / 6A02Al composite can be obtained. As shown in , the domain size of the shot peening layer is the smallest at the surface and increases with the increase of layer depth. When the domain size exceeds 250 μm, it approaches the size of the undeformed layer of the matrix.
The microstrain of the shot peening layer of SiCw / 6A02Al composite is shown in . The maximum microstrain of the shot peening layer of SiCw / 6A02Al composite appears on the surface, and decreases continuously with the increase of layer depth until it is close to the matrix layer. The microstrain in the shot peening layer is mainly caused by the violent plastic deformation of the material during the impact of the projectile. The thinning of the domain size and the increase of microstrain will increase the dislocation density in the material, which will affect the mechanical properties of the alloy.
As can be seen in , the equivalent stress of the surface matrix and the external load stress at the initial loading stage are basically linear. When the external load stress exceeds a certain degree, the matrix equivalent stress and the external load stress of the sample deviate from the linear relationship. Considering that the X-ray can only measure the elastic stress in the material, when the X-ray stress and the external load stress deviate from the linear relationship, it indicates that the plastic strain has occurred on the surface matrix at this time. In the figure, the vertical coordinate of the linear turning point (matrix effective stress) is the yield strength of the sample matrix surface layer. After comparison, it was found that the yield strength of the sample surface matrix was obviously improved after shot peening. It must be pointed out that the yield strength measured by X-ray in this work corresponds to the effective bearing stress of the matrix at the straight-line turning point, so the concept is different from the yield strength measured by conventional tensile tests (σ 0.2), but the X-ray diffraction method is the only test method that can independently study the stress of the matrix in the composite material.
Figure 8. Effective stress (σeff) of matrix on surface vs the applied stress (σapp) for the 20%SiCw/6A02Al composite.
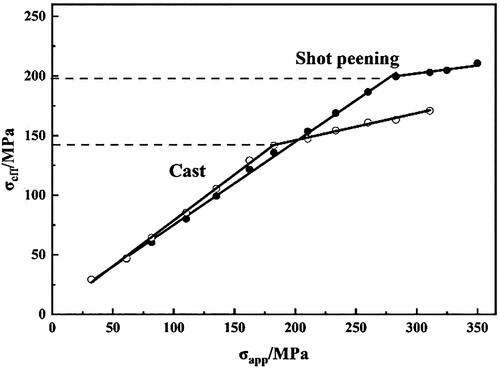
shows that the yield strength of the surface matrix of the sample after shot peening is significantly greater than that of the original cast state. When the depth exceeds 0.5 mm, the two curves tend to coincide, which is the actual surface plastic-layer thickness of the composite. The above measurement results of half value breadth of X-ray diffraction profile have confirmed that shot peening can cause subgrain refinement, lattice distortion and an increase in dislocation density in the surface matrix of the sample, that is, an obvious microstructure strengthening effect which can effectively improve the surface yield strength of the material occurs [Citation22,Citation23]. The microstructure strengthening of materials subjected to shot peening is accomplished by altering the microstructure of the plastic deformation layer. The mechanical properties of such materials primarily hinge on their structural characteristics. Shot peening aims to enhance the surface mechanical properties by modifying the surface area's structure through the impact of shot particles. According to the Hall-Petch formula, there exists a direct relationship between the material's yield strength (σ) and the block size (D):
(7)
(7) In the formula, σ0 represents the yield strength of the fully annealed material. It is evident from the equation that the yield strength of the material diminishes as the grain size increases. Moreover, the interaction among dislocations also influences the material's strength, and their relationship conforms to the Bailey-Hirsch formula:
(8)
(8) In the formula, ρ is the dislocation density, b is the Burgers vector of the dislocation, β and μ are the material constants. It can be seen from the formula that the dislocation density is also positively correlated with the yield strength of the material.
Figure 9. Yield strength (σyie) of matrix vs depth (d) from surface of the 20%SiCw/6A02Al composite.
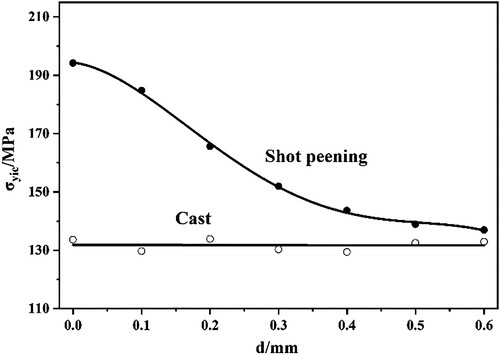
In addition, due to the residual compressive stress state of the surface matrix after shot peening, it will also contribute to improving the yield strength of the surface layer of the material.
Conclusion
X-ray diffraction technology is an effective means to study the mechanical properties and microstructure of composite materials matrix, which can not only measure the residual stress on the surface of the material, but also reveal the surface strengthening effect of the material.
After shot peening of the cast 20% SiCw / 6A02Al composite, the surface matrix of the sample presents a large residual compressive stress, and the half value breadth of the X-ray diffraction line increases obviously. The domain size of the material shot peening layer is obviously refined, and the domain size increases continuously along the depth direction until it is similar to the matrix layer. In addition, the microstrain of the shot peening layer decreases and tends to be stable with the increase of the depth.
Shot peening can significantly increase the yield strength of the surface matrix of the composite, which is mainly related to the subgrain refinement, lattice distortion and increase in dislocation density of the surface matrix.
Disclosure statement
No potential conflict of interest was reported by the author(s).
References
- Rajak DK, Pagar DD, Kumar R, et al. Recent progress of reinforcement materials: a comprehensive overview of composite materials. J Mater Res Technol. 2019;8(6):6354–6374. doi:10.1016/j.jmrt.2019.09.068
- Kim CS, Cho K, Manjili MH, et al. Mechanical performance of particulate-reinforced Al metal-matrix composites (MMCs) and Al metal-matrix nano-composites (MMNCs). J Mater Sci. 2017;52(23):13319–13349. doi:10.1007/s10853-017-1378-x
- Singh L, Kumar S, Raj S, et al. Aluminium metal matrix composites: manufacturing and applications. IOP Conf Ser Mater Sci Eng. 2021;1149:012025.
- Orifici AC, Herszberg I, Thomson RS. Review of methodologies for composite material modelling incorporating failure. Compos Struct. 2008;86(1–3):194–210. doi:10.1016/j.compstruct.2008.03.007
- Benedetti M, Fontanari V, Höhn BR, et al. Influence of shot peening on bending tooth fatigue limit of case hardened gears. Int J Fatigue. 2002;24(11):1127–1136. doi:10.1016/S0142-1123(02)00034-8
- Unal O, Varol R. Surface severe plastic deformation of AISI 304 via conventional shot peening, severe shot peening and repeening. Appl Surf Sci. 2015;351:289–295. doi:10.1016/j.apsusc.2015.05.093
- Zhang X, Liu D. Effects of electrospark surface strengthening and shot peening on fretting fatigue of Ti811 alloy at elevated temperature. J Mater Eng. 2006;9:31.
- Huang JJ, Wang Z, Bian K, et al. Investigation for different peening techniques on residual stress field of SiCw/Al composite. J Mater Eng Perform. 2013;22(3):782–786. doi:10.1007/s11665-012-0303-3
- Liu HB, Zhu WL, Jiang CH, et al. Microstructure evolution and residual stress distribution of nanostructured Mg-8Gd-3Y alloy induced by severe shot peening. Surf Coat Technol. 2020;404:(126465), doi:10.1016/j.surfcoat.2020.126465
- Chen M, Liu HB, Wang LB, et al. Investigation on the thermostability of residual stress and microstructure in shot peened SAF 2507 duplex stainless steel. Vacuum. 2018;153:145–153. doi:10.1016/j.vacuum.2018.04.015
- Alhamdany AA, Khenyab AY, Sabah A. Influence of shot peening on the corrosion and fatigue behavior of Al 6061. AIP Conf Proc. 2022: 060001.
- González J, Bagherifard S, Guagliano M, et al. Influence of different shot peening treatments on surface state and fatigue behaviour of Al 6063 alloy. Eng Fract Mech. 2017;185:72–81. doi:10.1016/j.engfracmech.2017.03.017
- Jiang CH, Zhan K, Yang CZ. Shot peening and X-ray diffraction characterization of materials. Science Press; 2019. p. 523–567.
- Wang CX, Jiang CH, Chai Z, et al. Estimation of microstructure and corrosion properties of peened nickel aluminum bronze. Surf Coat Technol. 2017;313:136–142. doi:10.1016/j.surfcoat.2017.01.073
- Wang LB, Zhao YT, Jiang CH, et al. Investigation on microstructure and properties of electrodeposited Ni-Ti-CeO 2 composite coating. J Alloys Compd. 2018;754:93–104. doi:10.1016/j.jallcom.2018.04.288
- Zhu KY, Li ZQ, Fan GL, et al. Thermal relaxation of residual stress in shot-peened CNT/Al-Mg-Si alloy composites. J Mater Res Technol. 2019;8(2):2201–2208. doi:10.1016/j.jmrt.2019.01.023
- Zhu KY, Jiang CH, Li ZQ, et al. Residual stress and microstructure of the CNT/6061 composite after shot peening. Mater Des. 2016;107:333–340. doi:10.1016/j.matdes.2016.06.030
- Zhang Z-P, Qi Y-H, Denis D, et al. Microstructure variation and hardness diminution during low cycle fatigue of 55NiCrMoV7 steel. J Iron Steel Res Int. 2007;14(6):68–73. doi:10.1016/S1006-706X(07)60093-4
- Xie ZW, Wang LP, Wang XF, et al. Influence of high temperature annealing on the structure, hardness and tribological properties of diamond-like carbon and TiAlSiCN nanocomposite coatings. Appl Surf Sci. 2011;258(3):1206–1211. doi:10.1016/j.apsusc.2011.09.072
- Bagherifard S, Pariente IF, Ghelichi R, et al. Effect of shot peening on residual stresses and surface work-hardening in cold sprayed coatings. 8th international conference on fracture and damage mechanics, Malta; 2009. p. 397-+.
- Fathallah R, Sidhom H, Braham C, et al. Effect of surface properties on high cycle fatigue behaviour of shot peened ductile steel. Mater Sci Technol. 2003;19(8):1050–1056. doi:10.1179/026708303225003027
- Luan WZ, Jiang CH, Ji V, et al. Effect of shot peening on surface mechanical properties of TiB2/Al composite. J Mater Sci. 2009;44(10):2454–2458. doi:10.1007/s10853-009-3310-5
- Wang CX, Jiang CH, Zhao YT, et al. Surface mechanical property and residual stress of peened nickel-aluminum bronze determined by in-situ X-ray diffraction. Appl Surf Sci. 2017;420:28–33. doi:10.1016/j.apsusc.2017.05.108