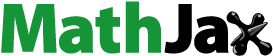
Abstract
Strawberry is the most economic important soft fruit in the world, and its production is susceptible to orchard and postharvest losses due to its high susceptibility to fungal diseases. In the last two decades, new agricultural techniques, which include application of plant growth promoting microorganisms, have been developed. Their activity is reflected in improving root volume and nutrient uptake from the soil, increased fruit yield and quality, and as an alternative to the use of synthetic fungicides in protection of disease and insects. In this study an effects of the three microbiological preparations on phenological and productivity of plants, chemical and physical properties of the fruits, and the control of B. cinerea on strawberry were studied. Total marketable fruit yield per plant ranged from 0.38 kg (control) to 0.57 kg (Fitohelp). The plants treated with T. harzianum had the highest fruit mass (34.2 g) at the first harvest, while Fitohelp-treated plants had the highest fruit mass (24.3 g) at the second harvest. The decrease in fruit mass and firmness after storage were 3.5% (Bacterie) to 9.1% (control), 10.1% to 35.2% (T. harzianum), respectively. The highest efficacy in controlling B. cinerea in storage fruits was obtained with the combination of synthetic fungicides and Fitohelp (92.86%). Plants treated with Fitohelp and T. harzianum had significantly higher values of yield of marketable fruits, while a combination of conventional fungicides and preparations based on B. subtilis was most effective in control of B. cinerea.
1. Introduction
Cultivated strawberry (Fragaria × ananassa Duch.) is the most important soft fruit in the world (Natsheh et al., Citation2015). Strawberries are of economic importance due to their unique fruit flavor, health benefits, and nutritional aspects (Shen et al., Citation2019). In addition, strawberry is an excellent source of natural antioxidants such as carotenoids, phenols, vitamins, anthocyanins, and flavonoids, which have a remarkably high capacity to scavenge free radicals (Giampieri et al., Citation2012). The annual world production of strawberries in last five years is more than 8.1 million tons (FAOSTAT, Citation2022), but losses during postharvest storage of strawberries are estimated to be up to 40% (Duran et al., Citation2016). Strawberry production is vulnerable to orchard and postharvest losses due to high susceptibility to fungal diseases such as root rot, various diseases of the aboveground organs, and gray mold. In addition, fruit texture is soft and susceptible to mechanical injury as well as proliferation of various pathogens (Hutton et al., Citation2013; Rico et al., Citation2019).
Various microorganisms such as Botrytis cinerea, Rhizopus stolonifer, Mucor spp., Colletotrichum spp., and Penicillium spp. are the most commonly reported fungal pathogens affecting strawberry fruit (Feliziani & Romanazzi, Citation2016). B. cinerea, the causal agent of gray mold, is one of the most destructive and economically important pathogens of strawberries. The fungus parasitizes almost all parts of the plant at all stages of development, and symptoms are most commonly described as gray rot, gray mold, late blight, brown rot of fruit, and seedling damping-off has also been noted (Tanović et al., Citation2011). Fruits are most susceptible during cold storage and transportation (Liu et al., Citation2013). Various strategies have been used in the past to control gray mold on fruit, such as various agricultural measures, breeding of resistant plant varieties, and application of chemical fungicides. A common practice to prevent fruit decay after harvest is the use of synthetic fungicides. Chemical control involves the use of conventional synthetic fungicides and is often an essential measure to control this pathogen. Preparations based on pyrimethanil, cyprodinil and fludioxonil, boscalid, combinations of boscalid and pyraclostrobin, fluopyram and trifloxystrobin. In addition, many pathogens are showing resistance to the pesticides used today, and customers are demanding natural, residue-free, and minimally processed foods (Alijani et al., Citation2022; Feliziani & Romanazzi, Citation2016).
In the last two decades, new agricultural techniques have been developed with an emphasis on the use of plant growth promoting microorganisms (PGPMs). Biofertilizers or biostimulants containing beneficial microorganisms have the ability to aggressively colonize the rhizosphere, plant roots, or both when applied to seeds or plants. These microorganisms have the potential to promote plant growth by releasing metabolites into the rhizosphere that stimulate growth. Their activity is reflected in plant growth through the release of plant growth hormones such as auxin, which promotes the growth of root hairs and the development of lateral roots in the plant. Improving root volume increases the rate of nutrient uptake from the soil, which leads to improved plant growth and fruit yield and promotes plant development under natural or stressed conditions and strawberry yield and quality (Cho & Lee, Citation2013; Vejan et al., Citation2016). İn addition, plant growth promoting microorganisms might reduce the cost of synthetic mineral fertilizers, which will be contribute decrease the harmful effect of synthetic fertilizers on agricultural areas and the environment (Ipek, Citation2019). They can also improve plant nutrition and enhance fruit quality in terms of flavor and nutritional value (Kundan et al., Citation2015; Morais et al., Citation2019).
As an alternative to the use of synthetic fungicides, interest in more environmentally friendly solutions has also increased in recent years, such as the use of natural products with pronounced pesticidal activity. The alternative methods should be applicable and environment-friendly and they should be presented to farmers for management of plant diseases in agriculture (Kaymak, Citation2021). According to De Silva et al. (Citation2019), one of the most economical and important agricultural strategies for disease control with minimal environmental impact is the use of plant growth-promoting microorganisms. Certain antagonistic microorganisms such as fungi, yeasts, and bacteria can be used to prevent diseases during the ripening and postharvest stages of fruits. The advantages of biopesticides are many, including: their natural origin, their lower toxicity compared to synthetic pesticides, their selectivity for beneficial organisms, their lower persistence in the environment, their shorter pre-harvest interval, and their wide range of applications. These microorganisms have several mechanisms that can act simultaneously, such as competition for nutrients and niches, antibiosis, lytic enzymes, and also induction of defense responses of the harvested plant product and others (Montesinos et al., Citation2015). The mode of action of biopesticides is different from the mode of action of synthetic pesticides, which may facilitate the suppression of resistant populations of pests. In this context, Bacillus subtilis is a biological option for the control of various strawberry diseases, which also prevents rot in the postharvest stage of the fruit (Oregel-Zamudio et al., Citation2017). In general, biopesticides and biostimulants also have some disadvantages. Some of them are more difficult to implement, have a lower spectrum of activity, act more slowly than synthetic pesticides, have only a preventive effect, have a shorter shelf life, are incompatible with some other products and need to be applied frequently (Chandler et al., Citation2011). The aim of this study was to investigate the influence of different microorganisms to improve fruit yield, quality and storage and biocontrol of gray mold on strawberry cultivar ‘Clery’.
2. Materials and methods
2.1. Plant, research design and environmental measurements
The research was conducted in a strawberry field plantation established in 2018 on an area of 3 ha in the village of Selevac in central Serbia. The plantation was established in the first half of August by planting cool-stored plants (Frigo). A single row cultivation system on slopes was applied. The spacing of hedges on slopes was 16.5 cm, i.e. 6 plants were planted per meter of slope. The orchard was managed according to the integrated cropping system on sandy loam soil with an average pH of 6.3. The experiment was laid as a Randomized Complete Block Design (RCBD) with four replicates (30 plants per replicate). In the period just before flowering (first half of September), all flowers were removed by hand. In the experiments, the effects of the following microbiological preparations were studied: Bacteria (based on Bacillus subtilis 109 CFU/ml, Bacillus megaterium 108 CFU/mL and Saccharomyces spp.107 CFU/mL, Green Grown Group, Serbia), Fitohelp (based on Bacillus subtilis 4 × 109 CFU/mL, BTU Center, Ukraine) and Trichoderma harzianum (1010 CFU/mL, Green Grown Group, Serbia), on the productive characteristics of the strawberry plants and the physical and chemical quality of the fruits. The concentration of the MOs was determined by the manufacturer of the preparation. In addition, the effects of the microbiological preparations on the control of B. cinerea on strawberry fruit were studied. The microbiological preparations were applied using a drip irrigation system at a dosage of 5 l/ha (Bacterie and Fitohelp) and 0.5 l/ha (T. harzianum) two and four weeks after planting and the next spring four and two weeks before the expected beginning of flowering. In addition, the microbiological preparations were applied to the leaves at the same times according to the protection program. Fruits were harvested at the stage of commercial ripeness and transported to our laboratory within 2 hours. Fruits were selected based on uniformity of size, shape, and color, and absence of external physical damage and fungal infections.
Environmental measurements were performed daily. Air temperature, relative humidity, and daily precipitation were measured. Air temperature and humidity were measured at a height of 2 m with MeteosCompact automatic weather station (Pessl Instruments GmbH, Austria) placed in close proximity to the planting. Precipitation was measured every rainfully day with a ClimeMET CM1088 rain gage placed close by weather station and expressed in L/m2.
2.2. Determination of the phenological characteristics of the plants, productivity and physical properties of the fruits
The following productivity traits were analyzed: number of crowns per plant, diameter of the crowns, number of inflorescences per crown, number of fruits per inflorescence, number of leaves per plant, and yield per plant (total and separately per crop in kg). According to the UPOV code for strawberries, the following phenological characteristics were studied: Beginning of flowering - when 10% of flowers are open, full flowering - when 90% of flowers are open and beginning of harvest - when 80% of the surface has a red color. The study of physical characteristics of fruits after harvest included: mass of fruits (g), diameter of fruits (mm) and firmness (kg/cm2). The harvested fruits were stored in a refrigerator at a temperature of 6 °C, and after three days of storage, the changes in the physical and chemical quality of the fruits were studied. After storaged of fruits the following changes were observed: Decrease in mass (%) and decrease in firmness (%). The percentage weight loss was calculated according to the following EquationEquation (1)(1)
(1) :
(1)
(1)
where weight (i) = the initial weight of berries, weight (t) = the weight of berries at time t.
Fruit firmness was determined using a digital bench top firmness analyzer (AMSTAT with a diameter of 4.0 mm). The parameters for strength analysis were as follows: Release force = 10 g and penetration depth = 5 mm. The physical properties of the fruits were determined in four replicates, each replicate comprising 15 fruits. Analysis of variance was performed using the STATISTICA 9 software package. Significant differences between means determined at p < 0.05 were measured using Student’s t-test.
2.3. Determination of total soluble solids (TSS), titratable acidity (TA), and ascorbic acid (AA)
After harvest, fruits were stored at 3 °C and analyzed within 24 hours. A total of 500 g of undamaged berries were selected and manually crushed to obtain the juice. For the analyzes, the juices were centrifuged at 14,000 rpm for 20 min. The chemical properties of the fruits were determined in four replicates. The total soluble solids content (TSS) of the berries was determined using a refractometer (Atago, bag PAL -1. Kyoto, Japan). Titratable acidity (TA) was determined by titrating the berry juice with 0.1 N NaOH to a pH of 7.0. Acidity was expressed as a percentage of malic acid. Ascorbic acid was quantified using a Merck reflectometer (Merck RQflex, Darmstadt, Germany). Fruit samples (5 g) and 20 ml of oxalic acid (1%) were mixed, homogenized for 1 minute, and filtered. Polyvinyl-polypyrrolidone (PVPP) (500 g) was added to 10 mL of the filtered sample to remove phenols, and 6–7 drops of H2SO4 (25%) were added to lower the pH below 1.14. Results were expressed as mg ascorbic acid 100 g−1 FW.
2.4. Determination of total phenolic content (TP)
Total phenolic content was determined by the Folin-Ciocalteu method with slight modifications (Waterman & Mole, Citation1994). Fruits (10 g) were extracted with MeOH in an ultrasonic bath for 30 min and then filtered. Two hundred microliters of the extracts were added to 1 mL of 1:10 diluted Folin-Ciocalteu reagent. After 4 minutes, 800 μl of sodium carbonate (75 g/L) was added. After two hours of incubation at room temperature, absorbance was measured at 765 nm. Gallic acid (0–100 mg/L) was used to calibrate a standard curve. Results were expressed as milligrams of gallic acid equivalent per 100 g fresh weight (mg GAE/100 g f.w. (fresh weight)).
2.5. Determination of total anthocyanin content (TAnt)
Total anthocyanin content was analyzed according to the procedure described in European Pharmacopeia 6.0 (Citation2008) with slight modifications. Fresh fruits (100 g) were crushed impromptu. Approximately 10 g of the crushed, accurately weighed berries or juice (10 g equivalent of fresh berries) was mixed with methanol (95 mL) and mechanically stirred for 30 min, then filtered into a 100-mL volumetric flask. The filtrate was rinsed and diluted to 100 mL with methanol. A 50-fold dilution of this solution in a 0.1% hydrochloric acid solution in methanol was prepared. The absorbance of the solution was measured at 528 nm using a 0.1% solution of hydrochloric acid in methanol as a balancing liquid.
The percentage content of anthocyanins, expressed as cyanidin-3-glucoside chloride, was calculated according to the following EquationEquation (2)(2)
(2) :
(2)
(2)
A = absorbance at 528 nm; 718 = specific absorbance of cyanidin-3-glucoside chloride at 528 nm; m = mass of the substance to be examined (in grams).
2.6. Determination of the effectiveness of conventional and microbiological protection
In the experiment, the effects of a conventional protection program based on synthetic fungicides (PYRUS, Arysta LifeScience, Belgium; SWİTCH, Syngenta, Switzerland), the effects of microbiological preparations based on B. subtilis and T. harzianum, and the combination of synthetic fungicides and the above-mentioned biopesticides in the control of B. cinerea on strawberry were studied (). The trial was conducted according to the EPPO standard method PP 1/16(3). Up to the time of the experiment, regular protective measures against other diseases and pests were carried out in the plantation. At the time of the experiment, the plants were in good health and showed no symptoms of disease or pest infestation. The treatments were carried out with a backpack sprayer ‘SOLO 425’ (SOLO Kleinmotoren GmbH, Germany). The formulations were applied with 500 l of water per ha. For the evaluation of the experiment, 100 undamaged strawberry fruits were picked from each experimental plot at the first harvest. The harvested fruits were stored in a refrigerator at a temperature of 6 °C. After three days of storage, the number of infected fruits was determined for each replicate and each treatment tested. The untreated plants served as controls.
Table 1. The protection program based on synthetic fungicides and microbiological preparations examined in the experiment.
Data were collected on the number of infected fruits per sample and the comparison of means, i.e. significance of differences between treatment effects, using Student’s t-test. The mean percentage of infected fruit per replicate (x) was previously converted using a statistical formula: √x + 0.5, and these data were used for analysis of variance and comparison of means (Gomez & Gomez, Citation1984). Fungicides efficacy was determined using the Abbott following EquationEquation (3)(3)
(3) :
(3)
(3)
E – efficacy (%); K – percentage of infected fruits in the untreated plot (%); and T – percentage of infected fruits in the treated plot (%).
3. Results and discussion
3.1. Environmental conditions
Environmental parameters during the spring period (between flowering and harvest) in 2019 are shown in . The highest rainfall occurred during the third week of May (55.2 L/m2), followed by the second week (36.4 L/m2), which overlapped with the beginning of the harvest period. More than 160 L/m2 of precipitation also fell during fruit development stage, which was 2-fold higher than the multi-year average. Humidity values were similar to precipitation. As expected, average humidity values were higher during the higher rainfall periods.
3.2. Phenological characteristics of plants, productivity and physical properties of fruits
The plant growth-promoting microorganisms had an influence on the phenological characteristics of the strawberry plant (). All phenological stages studied occurred earlier in the plants treated with Fitohelp. In addition, all plants treated with PGPM had an earlier harvest stage compared to control plants, while a statistically significance difference was recorded between control plants and plants supplied with Fitohelp, only in beginning of second harvest. Also, significance difference between treatments did not recorded. A similar effect was observed by Morais et al. (Citation2019) who found that PGPM stimulates plants to develop reproductively earlier, and offers potential economic benefits to growers by allowing them to harvest and sell their fruit earlier.
Plant growth-promoting microorganisms had a significant effect on strawberry plant productivity (). The number of crowns per plant ranged from 2.6 to 2.9. Plants supplied with T. harzianum and Fitohelp had significantly higher crown diameter and number of inflorescences per crown compared to plants supplied with Bacterie and control plants. In addition, these PGPMs contributed to a higher number of leaves per plant. The number of flowers per inflorescence ranged from 7.0 (control plants) to 9.8 (Bacterie-treated plants). The applied PGPMs improved and promoted the development of the root system of strawberry plants, resulting in a higher production of crowns per plant. Auxins are responsible for the division, expansion, and differentiation of plant cells and tissues and are known to increase the rate of xylem and root formation (Chebotar et al., Citation2022).
Table 2. Productivity characteristics of strawberry plants treated with PGPMs.
The results showed that inoculation with PGPMs increased the number of leaves per plant. The highest number of leaves (29.8) was observed in plants inoculated with Fitohelp (Bacillus subtilis). Similar results were observed in previous reports that application of PGPMs increased plant growth and number of leaves in three strawberry cultivars (Badar et al., Citation2022). Accorting to Siddiqui et al. (Citation2020) an availability of nutrients during critical periods of initial plants development and the synergistic plant growth-promoting effect of introduced beneficial microorganisms, which enhance the physical, chemical, and biological properties of soil. Plants treated with Fitohelp had the highest fruit yield at the first harvest (0.28 kg), followed by plants treated with T. harzianum (0.22 kg) and Bacterie (0.18 kg). All PGPMs applied contributed to a higher yield of harvested fruit at the first harvest compared to the control plants. In addition, the percentage of total fruit harvested per plant in the first harvest ranged from 19.5% (control plants) to 32.5% (Fitohelp). Plants treated with Fitohelp and T. harzianum had the significantly higher values of this trait. Higher yield of harvested fruits was recorded in the second harvest than in the first harvest for all treatments. The plants treated with T. harzianum had the highest yield in the second harvest (0.34 kg), while the plants treated with Bacterie had the lowest yield (0.24 kg). The percentage of harvested fruits was also higher in the second harvest than in the first harvest. The highest percentage of harvested fruit was in the T. harzianum treated plants and the lowest in the Bacterie treated plants. Total marketable fruit yield per plant ranged from 0.38 kg (control) to 0.57 kg (Fitohelp). Plants treated with Fitohelp and T. harzianum had significantly higher values of this trait compared to the other treatments. Results from other authors also show that plants treated with growth-promoting bacteria such as Bacillus simplex, Paenibacillus polymyxa, and Bacillus spp. have the potential to increase yield and growth of strawberries (Erturk et al., Citation2012). PGPMs specifically activate growth regulators such as auxin, gibberellins, cytokinin, solubilization of inorganic phosphorus, and mineralization of nutrients along with symbiotic N fixation (Zahir et al., Citation2004). The coexistence between the root system and rhizosphere microorganisms has resulted in plant growth being closely linked to rhizosphere microorganisms, with bacteria being the most active in influencing soil fertility. The soil bacteria settle in the rhizosphere and endo-rhizosphere of plants and make up the rhizosphere microbiomes which have a positive effect on plants by promoting their growth, development, nutrient regulation, and biocontrol activities (Hakim et al., Citation2021; Kumar & Dubey, Citation2020). In general, rich soil bacteria would improve soil fertility and create a better microecological environment in the rhizosphere, which in turn would promote plant growth (Liu et al., Citation2022; Marx, Citation2004). The inoculation of T. harzianum increase yield, nutrients absorption and agronomic biofortification of plants. Also, an inoculation of T. harzianum had positively incremented concentrations of K, Mg, Ca, Zn, Fe, Cu and Mn in leaves of many plants spesies that consequently can improve human nutrition (Gato et al., Citation2023).
At the first harvest, plants treated with T. harzianum had the highest fruit mass (34.2 g), while control plants had the lowest mass (). In addition, the plants treated with Fitohelp had higher fruit length; while the plants treated with T. harzianum had higher fruit width compared to the other treatments. According to Mikiciuk et al. (Citation2019), the applied species of rhizosphere bacteria and mycorrhizal fungi increase the yield and average weight of strawberry fruits, which is due to improved root activity that contributes to the uptake of nutrients from the soil. Increased growth of root hairs and development of lateral roots in strawberry plants also had an impact on yield increase (Meng et al., Citation2016). Fruit firmness is one of the most valued traits of strawberries by consumers and an important indicator of shelf life, preservation, and market value (Virgen-Ortiz et al., Citation2019). Firmness of fruit harvested from plants treated with T. harzianum and Fitohelp was significantly higher than that of fruit from control and Bacterie-treated plants. Fruit firmness at first harvest varied from 3.4 kg/cm2 to 4.7 kg/cm2. After three days of storage, fruit mass and firmness of all fruits decreased. There was significantly less mass in the fruits of the control plants and the plants treated with Fitohelp. The decrease in fruit mass ranged from 4.5% (Bacterie) to 9.1% (control). The percent decrease in firmness, after storage ranged from 25.6% (Fitohelp) to 35.2% (T. harzianum). Nayak et al. (Citation2019) found that fruit firmness decreased with increasing storage time for all treatments. According to these authors, after 10 days of storage, the least loss of firmness was observed in fruits treated with Debaryomyces hansenii and Bacillus suptilis.
Table 3. Physical properties of the fruits harvested in the first harvest.
At the second harvest, Fitohelp-treated plants had the highest fruit mass (24.3 g), while control plants had the lowest mass (). In addition, Fitohelp-treated fruits had higher values for length and width in the second harvest compared to the other treatments. For all treatments, higher values for fruit firmness were observed in the second harvest than in the fruits of the first harvest. Similar to the first harvest, the fruit firmness of the plants treated with Fitohelp (4.8 kg/cm2) was significantly higher than the fruits of the control and plants treated with Bacterie (4.1 kg/cm2 and 4.3 kg/cm2, respectively). However, after storage, the fruits of the plants treated with Fitohelp showed a significant decrease in fruit mass (5.5%) and firmness (15.4%) compared to the other treatments.
Table 4. Physical properties of the fruits harvested in the second harvest.
3.3. Analysis of the chemical quality of the fruit
The plant growth-promoting microorganisms had a significant effect on the strawberry fruit quality at the first harvest (). Fruits from plants treated with T. harzianum had the highest content of TSS (10.7%), while those treated with Fitohelp had the lowest content (10.1%). The authors recorded that an applied of PGPMs increased the content of soluble solids (TSS) and vitamin C in fruits from different strawberry cultivars compared to control (Badar et al., Citation2022; Erturk et al., Citation2012), which is not in-line with finding in our study. This can potentially be linked to larger fruits from biostimulants treatments and dilution effects due to the increased water content of fruit (Krüger et al., Citation2012). The content of total phenolics in the fruits of the first harvest ranged from 66.3 mg to 83.2 mg. In the current study, the application of probiotic bacteria significantly increased the total phenolic and total anthocyanin content in fresh strawberry fruits compared with the untreated control. Fruits from plants treated with Bacterie had significantly higher levels of TP compared to other treatments. Total anthocyanin content at first harvest ranged from 8.2% to 12.2%. Fruit treated with Bacterie had significantly higher values of this trait compared to the other treatments. Fruits from plants inoculated with isolates of PGPMs had significantly higher levels of phenolics, carotenoids, flavonoids, and anthocyanins than the untreated control (Rahman et al., Citation2018). At the first harvest, fruit ascorbic acid contents ranged from 32.6 to 35.4 mg/100 g−1 f.w. Fruits from Fitohelp treated plants and the control had significantly higher levels of AA. After storage, fruits had similar levels of TSS. A significant decrease in the values after storage was observed in the contents of TA and AA. Bacteria treated fruits showed the highest content of TA, while control fruits showed the highest content of AA. However, an increase in the content of total phenols and total anthocyanins was observed. The highest contents of these traits were found in the fruits of the plants treated with Bacterie (94.5 mg and 15.5%, respectively), and the lowest in the fruits of the control plants (74.3 mg and 11.9%). Postharvest storage can also affect anthocyanin, phenolic compound levels and antioxidant capacity in strawberry fruit and can contribute to maintaining and increasing the content of these compounds (Ayala-Zavala et al., Citation2004). Based on the evaluation of the state of the photosynthetic apparatus and the analysis of chlorophyll ‘a’ fluorescence indices, including hierarchical cluster analysis, it was found that the rhizosphere bacteria had positive effects on the strawberry plants, which can be equated with improved chemical characteristics of the strawberry fruits (Paliwoda et al., 2022).
Table 5. Chemical properties of first harvest fruits treated with PGPMs after harvest and three days of storage.
In general, the fruits of the second harvest had lower chemical property content than the fruits of the first harvest, both at the time of harvest and after storage (). The greatest decrease in chemical properties in the fruits of the second harvest was observed in the values of total phenols and ascorbic acid. The decreased in the levels of those compounds can be linked to the higher number of harvested fruits in second harvest, as reported in previous studies (Schmitzer et al., Citation2023; Weber et al., Citation2018). Also, other authors have indicated a conditional-dependent trend in those parameters, which have been linked to the temperature, water, and nutrient availability, as well as light during maturing times (Simkova et al., Citation2023). However, the contents of TSS, TP and TAnt increased in the fruits after storage. The fruits of the plants treated with Bacterie had the highest levels of total phenols (65.6 mg and 72.9 mg, respectively) both at harvest and after storage compared to the other treatments. In addition, the fruits of the Fitohelp treated plants had the highest levels of total anthocyanins (8.2% and 10.2%, respectively), while the fruits of the control plants had the highest levels of ascorbic acid compared to other treatments (26.4 mg and 24.5 mg, respectively). A notable variability of anthocyanin concentrations, titratable acidity, ascorbic acid and soluble solids contents were found among samples of the same cultivar from the same harvest date, which indicating that degree of maturity, climatic factors, and post-harvest storage have strong effects on levels their contents (Fátima Lopes et al., Citation2007; Kim et al., Citation2015).
Table 6. Chemical properties of second harvest fruits treated with PGPMs after harvest and three days of storage.
3.4. Analysis of the effectiveness of conventional and microbiological protection
From the experimental results, it appears that the protection programs used provided satisfactory protection of strawberry fruit against B. cinerea (). The number of infected fruits after storage ranged from 4.5 (fungicides + Fitohelp) to 63.0 (untreated plot), with a significant difference between treatments. Among PGPMs applied individually in the control of B. cinerea, plants treated with T. harzianum had the lowest number of infected fruits. Statistical analysis also showed that between the effects of the two microbiological protection programs: Bacterie and Fitohelp there were no significant differences (p ≤ 0.05).
Table 7. Degree of infection of strawberry fruit with B. cinerea three days after storage and the effectiveness of protection programs.
Moreover, there were no significant differences in numbers of infected fruits between the effects of the two combinations of mixtures of conventional protection program and microbiological protection program (fungicides + Bacterie and fungicides + Fitohelp). However, the combinations of conventional protection program and T. harzianum had significantly lower efficacy than the previous combinations. The MOs used in the trial are not or only moderately sensitive to pyrimethanil and cyprodinil and fludioxonil (Barakat & Al-Masri, Citation2017), so each component in this combination had an effect on B. cinerea, resulting in greater efficiency. In addition, both combined protection programs had significantly lower numbers of infected fruits then the conventional protection program.
The efficacy of the conventional protection program was 84.92%. Other authors also reported the efficacy of synthetic fungicides based on cyprodinil and fludioxonil in controlling B. cinerea (Menzel et al., Citation2016). The highest efficacy in controlling B. cinerea in storage fruits was obtained with the combination of synthetic fungicides and Fitohelp (92.86%). A conventional protection program together with the preparation Bacterie was slightly less effective (89.68%). Of all the combinations of conventional and microbiological protection programs tested, the combination of synthetic fungicides and T. harzianum had the lowest efficacy (76.19%). Other authors also reported the effects of the combination of synthetic fungicides and Trichoderma spp. on B. cinerea. In a trial conducted by Barakat and Al-Masri (Citation2017), the efficacy of combining lower concentrations of pyrimethanil and T. harzianum and cyprodinil and fludioxonil with T. harzianum in controlling B. cinerea on strawberry in greenhouse was good and ranged from 90 to 92%. However, Khirallah et al. (Citation2016) demonstrated that the susceptibility of T. harzianum to fungicides may depend on both the active ingredient itself and its concentration. In addition, T. harzianum was found to be moderately sensitive to cyprodinil and fludioxonil (Hilber & Hilber-Bodmer, Citation1998).
The effectiveness of the microbiological protection program varied depending on the preparation used. The preparation Bacterie had an efficacy of 58.73% when used alone. The preparation Fitohelp had an efficacy of 60.32%. Of all the microbiological preparations used alone, the preparation based on T. harzianum had the highest efficacy (67.46%). According to Helbig and Bochow (2001), the efficacy of B. subtilis in controlling gray mold on strawberries was in the range of 16–40%. The results reported by Hang et al. (2005) showed that the efficacy of B. subtilis in controlling B. cinerea on strawberry under field conditions was 70%. In addition, there are numerous data on the efficacy of T. harzianum against B. cinerea. In a trial conducted by Barakat and Al-Masri (Citation2017), the efficacy of T. harzianum in controlling B. cinerea on strawberries was 50%. The same authors claim that the efficacy of T. harzianum may vary depending on the content of spores in the preparation. Thus, it was found that at a content of 109 spores per ml, the efficacy was 48%, while at a concentration of 108 spores per ml, a much lower efficacy (29%) was obtained. Some other authors noted the good efficacy of preparations based on T. atroviride (LC52) and T. harzianum (T22) in controlling B. cinerea on strawberries (Robinson-Boyer et al., Citation2009; Xiangming et al., Citation2010).
4. Conclusion
The plant growth promoting microorganisms had a significant effect on plant productivity and fruit quality of strawberry cultivar ‘Clery’. The use of PGPMs in strawberry production is justified as the products improve the vegetative development of strawberry plants, which produce significantly more marketable fruit. Plants supplied with Fitohelp and T. harzianum showed significantly higher values in the studied productivity traits, especially in terms of marketable fruits, 0.56 and 0.57 kg/plant, respectively. Bacterie showed significantly higher values in the chemical properties of the fruits, especially in contents of total phenols in both harvests (83.2 and 65.5 mg).
From the experimental results, it can be concluded that the application of synthetic fungicides in combination with microbiological preparations provides good results in the control of B. cinerea under the conditions of very high disease intensity in untreated plots. The most effective combination was a conventional fungicides and Fitohelp, with 92.86% uninfected fruits after storage, followed conventional fungicides and Bacterie (89.68%). Idependent application of PGPMs had the lowest efficiency in suppressing gray mold, which ranged between 58.73% (Bacterie) and 67.46% (T. harzianum).
Due to the improved productivity characteristics and increased fruit quality, the use of plant-promoting microorganisms should be part of the practice in strawberry cultivation. The results of the biocontrols also suggest that it is justified to include microbiological preparations in integrated pest management programs against B. cinerea in strawberry, considering all the benefits these biopesticides could have.
Author contributions
B.Dj., M.S. and N.T. designed the experiment; M.S. and N.Te. prepared samples for laboratory analysis; B.Dj., G.Z. and N.T. wrote the paper; N.Te. and G.Z. performed statistical analysis; N.T. and B.Dj. analysed the chemical properties; M.S. and N.M. performed proof reading and manuscript correcting. All authors have read and agreed to the published version of the manuscript.
Disclosure statement
No potential conflict of interest was reported by the author(s).
Additional information
Funding
Notes on contributors
Boban Djordjević
Boban Đorđević, full professor at the University of Belgrade-Faculty of Agriculture, research area is growing fruit trees and preserving fruits, as well as improving the biochemical properties of fruits. He has been employed at the Faculty of Agriculture since 2005.
References
- Alijani, Z., Amini, J., Ashengroph, M., Bahramnejad, B., & Mozafari, A. A. (2022). Biocontrol of strawberry anthracnose disease caused by Colletotrichum nymphaeae using Bacillus atrophaeus strain DM6120 with multiple mechanisms. Tropical Plant Pathology, 47(2), 1–13. https://doi.org/10.1007/s40858-021-00477-7
- Ayala-Zavala, F., Wang, S., Wang, C., & González-Aguilar, G. (2004). Effect of storage temperatures on antioxidant capacity and aroma compounds in strawberry fruit. Food Science and Technology, 37(7), 687–695. https://doi.org/10.1016/j.lwt.2004.03.002
- Badar, M. A., Mehmood, K., Hassan, I., Ahmed, M., Ahmad, I., Ahmad, N., & Hasan, M. U. (2022). Plant growth promothing bacterie (PGPB) enhance growth and yield of strawberry cultivars. Applied Ecology and Environmental Research, 20(3), 2187–2203. https://doi.org/10.15666/aeer/2003_21872203
- Barakat, R., & Al-Masri, M. I. (2017). Effect of Trichoderma harzianum in combination with fungicides in controlling gray mould disease (Botrytis cinerea) of strawberry. American Journal of Plant Sciences, 8(4), 651–665. https://doi.org/10.4236/ajps.2017.84045
- Chandler, D., Bailey, A. S., Tatchell, G. M., Davidson, G., Greaves, J., & Grant, W. P. (2011). The development, regulation and use of biopesticides for integrated pest management. Philosophical Transactions of the Royal Society of London. Series B, Biological Sciences, 366(1573), 1987–1998. https://doi.org/10.1098/rstb.2010.0390
- Chebotar, V. K., Chizhevskaya, E. P., Vorobyov, N. I., Bobkova, V. V., Pomyaksheva, L. V., Khomyakov, Y. V., & Konovalov, S. N. (2022). The quality and productivity of strawberry (Fragaria × ananassa Duch.) improved by the inoculation of PGPR Bacillus velezensis BS89 in field experiments. Agronomy, 12(11), 2600. https://doi.org/10.3390/agronomy12112600
- Cho, H. T., & Lee, R. D. (2013). Auxin, the organizer of the hormonal/environmental signals for root hair growth. Frontiers in Plant Science, 4, 448. https://doi.org/10.3389/fpls.2013.00448
- De Silva, N., Brooks, S., Lumyong, S., & Hyde, K. (2019). Use of endophytes as biocontrol agents. Fungal Biology Reviews, 33(2), 133–148. https://doi.org/10.1016/j.fbr.2018.10.001
- Duran, M., Aday, M., Zorba, N. N., Temizkan, R., Büyükcan, M., & Caner, C. (2016). Potential of antimicrobial active packaging ‘containing natamycin, nisin, pomegranate and grape seed extract in chitosan coating’ to extend shelf life of fresh strawberry. Food and Bioproducts Processing, 98, 354–363. https://doi.org/10.1016/j.fbp.2016.01.007
- Erturk, Y., Ercisli, S., & Cakmakci, R. (2012). Yield and growth response of strawberry to plant growth-promoting rhizobacteria inoculation. Journal of Plant Nutrition, 35(6), 817–826. https://doi.org/10.1080/01904167.2012.663437
- European Pharmacopoeia 6.0. (2008). Council of Europe. Strasbourg Cedex, pp. 1307–1308.
- FAOSTAT. (2022). FAOSTAT crops. http://faostat.fao.org/beta/en/#data/QCL
- Fátima Lopes, D. S., Escribano-Bailón, M. T., Pérez Alonso, J. J., Rivas-Gonzalo, J. C., & Santos-Buelga, C. (2007). Anthocyanin pigmentsin strawberry. LWT - Food Science and Technology, 40(2), 374–382. https://doi.org/10.1016/j.lwt.2005.09.018
- Feliziani, E., & Romanazzi, G. (2016). Postharvest decay of strawberry fruit: Etiology, epidemiology, and disease management. Journal of Berry Research, 6(1), 47–63. https://doi.org/10.3233/JBR-150113
- Gato, I. M. B., Da Silva Oliveira, C. E., Oliveira, T. J. S. S., Jalal, A., Moreira, V. A., Giolo, V. M., Vitória, L. S., De Lima, B. H., Vargas, P. F., & Filho, MCMT. (2023). Nutrition and yield of hydroponic arugula under inoculation of beneficial microorganisms. Horticulture, Environment, and Biotechnology, 64(2), 193–208. https://doi.org/10.1007/s13580-022-00476-w
- Giampieri, F., Tulipani, S., Alvarez-Suarez, J. M., Quiles, J. L., Mezzetti, B., & Battino, M. (2012). The strawberry: Composition, nutritional quality, and impact on human health. Nutrition (Burbank, Los Angeles County, Calif.), 28(1), 9–19. https://doi.org/10.1016/j.nut.2011.08.009
- Gomez, K. A., & Gomez, A. A. (1984). Statistical procedures for agricultural research (2nd ed.). John Wiley and Sons.
- Hakim, S., Naqqash, T., Nawaz, M. S., Laraib, I., Siddique, M. J., Zia, R., Mirza, M. S., & Imran, A. (2021). Rhizosphere engineering with plant growth-promoting microorganisms for agriculture and ecological sustainability. Frontiers in Sustainable Food Systems, 5, 617157. https://doi.org/10.3389/fsufs.2021.617157
- Hang, N. T. T., Oh, SO., Kim, GH., Hur, J. S., & Koh, Y. J. (2005). Bacillus subtilis S1-0210 as a Biocontrol Agentagainst Botrytis cinerea in Strawberries. The Plant Pathology Journal, 21(1), 59-63. https://doi.org/10.5423/PPJ.2005.21.1.059
- Hilber, W., & Hilber-Bodmer, M. (1998). Genetic basis and monitoring of resistance of Botryotinia fuckeliana to anilinopyrimidines. Plant Disease, 82(5), 496–500. https://doi.org/10.1007/s40858-021-00439-z
- Helbig, J., & Bochow, H. (2001). Effectiveness of Bacillus subtilis (Isolate 25021) in controlling Botrytis cinerea in strawberry. Journal of Plant Diseases and Protection, 108(6), 545–559.
- Hutton, D. G., Gomez, A. O., & Mattner, S. W. (2013). Macrophomina phaseolina and its association with strawberry crown rot in Australia. International Journal of Fruit Science, 13(1–2), 149–155. https://doi.org/10.1080/15538362.2012.698143
- Ipek, M. (2019). Effect of rhizobacteria treatments on nutrient content and organic and amino acid composition in raspberry plants. Turkish Journal of Agriculture and Forestry, 43, 88–95. https://doi.org/10.3906/tar-1804-16
- Kaymak, S. (2021). Effects of some commercial products on root and crown rot caused by Phytophthora cactorum in apple cultivation. Turkish Journal of Agriculture and Forestry, 46, 19–27. https://doi.org/10.3906/tar-2106-56
- Khirallah, W., Mouden, N., Selmaoui, K., Achbani, E., Benkirane, R., Touhami, A. O., & Douira, A. (2016). Compatibility of Trichoderma spp. with some fungicides under in vitro conditions. International Journal of Recent Scientific Research, 7(2), 9060–9067. http://webagris.inra.org.ma/doc/achbani02016.pdf.
- Kim, S. K., Kim, D. S., Kim, D. Y., & Chun, C. (2015). Variation of bioactive compounds content of 14 oriental strawberry cultivars. Food Chemistry, 184, 196–202. https://doi.org/10.1016/j.foodchem.2015.03.060
- Krüger, E., Josuttis, M., Nestby, R., Toldam-Andersen, T. B., Carlen, C., & Mezzetti, B. (2012). Influence of growing conditions at different latitudes of Europe on strawberry growth performance, yield and quality. Journal of Berry Research, 2(3), 143–157. doı: https://doi.org/10.3233/JBR-2012-036
- Kumar, A., & Dubey, A. (2020). Rhizosphere microbiome: Engineering bacterial competitiveness for enhancing crop production. Journal of Advanced Research, 24, 337–352. https://doi.org/10.1016/j.jare.2020.04.014
- Kundan, R., Pant, G., Jadon, N., & Agrawal, P. K. (2015). Plant growth promoting rhizobacteria: Mechanism and current prospective. Journal of Fertilizers & Pesticides, 06(02), 155. https://doi.org/10.4172/jbfbp.1000155
- Liu, J., Sui, Y., Wisniewski, M., Droby, S., & Liu, Y. (2013). Review: Utilization of antagonistic yeasts to manage postharvest fungal diseases of fruit. International Journal of Food Microbiology, 167(2), 153–160. https://doi.org/10.1016/j.ijfoodmicro.2013.09.004
- Liu, L., Xian, L., Tianyu, L., Xie, Y., Zhuoyang, C., & Fang, P. (2022). Bio-organic fertilizer with Bacillus subtilis F2 promotes strawberry plant growth and changes rhizosphere microbial community. Journal of Soil Science and Plant Nutrition, 22(3), 3045–3055. https://doi.org/10.1007/s42729-022-00866-0
- Marx, J. (2004). The roots of plant-microbe collaborations. Science (New York, N.Y.), 304(5668), 234–236. https://doi.org/10.1126/science.304.5668.234
- Meng, Q., Jiang, H., & Hao, J. J. (2016). Effects of Bacillus velezensis strain BAC03 in promoting plant growth. Biological Control, 98, 18–26. https://doi.org/10.1016/j.biocontrol.2016.03.010
- Menzel, C. M., Gomez, A., & Smith, L. A. (2016). Control of grey mould and stem-end rot in strawberry plants growing in a subtropical environment. Australasian Plant Pathology, 45(5), 489–498. https://doi.org/10.1007/s13313-016-0440-5
- Mikiciuk, G., Sas-Paszt, L., Mikiciuk, M., Derkowska, E., Trzciński, P., Głuszek, S., Lisek, A., Wera-Bryl, J., & Rudnicka, I. (2019). Mycorrhizal frequency, physiological parameters, and yield of strawberry plants inoculated with endomycorrhizal fungi and rhizosphere bacteria. Mycorrhiza, 29(5), 489–501. https://doi.org/10.1007/s00572-019-00905-2
- Montesinos, E., Francés, J., Badosa, E., & Bonaterra, A. (2015). Post harvest control. In B. Lugtenberg (Ed.), Principles of plant- microbe interactions. Microbes for sustainable agriculture (pp 193–202). Springer.
- Morais, M. C., Mucha, Â., Ferreira, H., Gonçalves, B., Bacelar, E., & Marques, G. (2019). Comparative study of plant growth promoting bacteria on the physiology, growth and fruit quality of strawberry. Journal of the Science of Food and Agriculture, 99(12), 5341–5349. https://doi.org/10.1002/jsfa.9773
- Natsheh, B., Abu-Khalaf, N., & Mousa, S. (2015). Strawberry (Fragaria ananassa Duch.) plant productivity quality in relation to soil depth and water requirements. International Journal of Plant Research, 5, 1–6. https://doi.org/10.5923/j.plant.20150501.01
- Nayak, S. L., Sethi, S., Sharma, R. R., Singh, D., & Singh, S. (2019). Improved control on decay and postharvest quality deterioration of strawberry by microbial antagonists. Indian Journal of Horticulture, 76(3), 502–507. https://doi.org/10.5958/0974-0112.2019.00079.3
- Oregel-Zamudio, E., Angoa-Pérez, V., Oyoque-Salcedo, G., Aguilar-González, C., & Mena-Violante, H. (2017). Effect of candelilla wax edible coatings combined with biocontrol bacteria on strawberry quality during the shelf-life. Scientia Horticulturae, 214, 273–279. https://doi.org/10.1016/j.scienta.2016.11.038
- Paliwoda, D., Mikiciuk, G., Mikiciuk, M., Kisiel, A., Sas-Paszt, L., & Miller, T. (2002). Effects of rhizosphere bacteria on strawberry plants (Fragaria × ananassa Duch.) under water deficit. International Journal of Molecular Sciences, 23(18), 10449. https://doi.org/10.3390/ijms231810449
- Rahman, M., Sabir, S., Mukta, J. A., Khan, M. A., Mohi-Ud-Din, M., Miah, G., Rahman, M., & Islam, T. (2018). Plant probiotic bacteria Bacillus and Paraburkholderia improve growth, yield and content of antioxidants in strawberry fruit. Scientific Reports, 8(1), 2504. https://doi.org/10.1038/s41598-018-20235-1
- Rico, D., Barcenilla, B., Meabe, A., González, C., & Martín-Diana, A. B. (2019). Mechanical properties and quality parameters of Chitosan-edible algae (Palmaria palmata) on eady-to-eat strawberries. Journal of the Science of Food and Agriculture, 99(6), 2910–2921. https://doi.org/10.1002/jsfa.9504
- Robinson-Boyer, L., Jeger, M. J., Xiang-Ming, X., & Jeffries, P. (2009). Management of strawberry grey mould using mixtures of biocontrol agents with different mechanisms of action. Biocontrol Science and Technology, 19(10), 1051–1065. https://doi.org/10.1080/09583150903289105
- Schmitzer, V., Stampar, F., Turk, A., Jakopic, J., Hudina, M., Veberic, R., & Smrke, T. (2023). Before or after planting? Mycorrhizal and bacterial biostimulants and extracts in ıntense strawberry (Fragaria × ananassa Duch.) production. Horticulturae, 9(7), 769. https://doi.org/10.3390/horticulturae9070769
- Shen, H., Wei, Y., Wang, X., Xu, C., & Shao, X. (2019). The marine yeast Sporidiobolus pararoseus ZMY-1 has antagonistic properties against Botrytis cinerea in vitro and in strawberry fruit. Postharvest Biology and Technology, 150, 1–8. https://doi.org/10.1016/j.postharvbio.2018.12.009
- Siddiqui, Y., Munusamy, U., Naidu, Y., & Ahmad, K. (2020). Integrated effect of plant growth-promoting compost and NPK fertilizer on nutrient uptake, phenolic content, and antioxidant properties of Orthosiphon stamineus and Cosmos caudatus. Horticulture, Environment, and Biotechnology, 61(6), 1051–1062. https://doi.org/10.1007/s13580-020-00277-z
- Simkova, K., Veberic, R., Hudina, M., Grohar, M. C., Ivancic, T., Smrke, T., Pelacci, M., & Jakopic, J. (2023). Variability in ‘Capri’ everbearing strawberry quality during a harvest season. Foods (Basel, Switzerland), 12(6), 1349. https://doi.org/10.3390/foods12061349
- Tanović, B., Hrustic, J., Mihajlovic, M., Grahovac, M., Delibasic, G., & Vuksa, P. (2011). Suppression of Botrytis cinerea and the problem of resistance to fungicides. Journal of Pesticides & Phytomedicine, 26(2), 99–110. https://doi.org/10.2298/PIF1102099T
- Vejan, P., Abdullah, R., Khadiran, T., Ismail, S., & Nasrulhaq, B. A. (2016). Role of plant growth promoting rhizobacteria in agricultural sustainability: A review. Molecules (Basel, Switzerland), 21(5), 573. https://doi.org/10.3390/molecules21050573
- Virgen-Ortiz, J., Morales-Ventura, J., Colín-Chávez, C., Esquivel-Chávez, F., Vargas-Arispuro, I., Aispuro-Hernández, E., & Martínez-Téllez, M. (2019). Postharvest application of pectic-oligosaccharides on quality attributes activities, of defense-related enzymes, and anthocyanin accumulation in strawberry. Journal of the Science of Food and Agriculture, 100(5), 1949–1961. https://doi.org/10.1002/jsfa.10207
- Waterman, P., & Mole, S. (1994). Analysis of phenolic plant metabolites. Blackwell Scientific Publication.
- Weber, N., Schmitzer, V., Jakopic, J., & Stampar, F. (2018). First fruit in season: Seaweed extract and silicon advance organic strawberry (Fragaria × ananassa Duch.) fruit formation and yield. Scientia Horticulturae, 242, 103–109. https://doi.org/10.1016/j.scienta.2018.07.038
- Xiangming, X., Robinson, L., Jeger, M., & Jeffries, P. (2010). Using combinations of biocontrol agents to control Botrytis cinerea on strawberry leaves under fluctuating temperatures. Biocontrol Science and Technology, 20(4), 359–373. https://doi.org/10.1080/09583150903528114
- Zahir, Z. A., Arshad, M., & Frankenberger, W. T. (2004). Advances in agronomy. Elsevere Science.