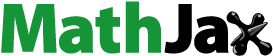
ABSTRACT
Amylases are among the most important enzymes for biotechnological and industrial applications. The present work aimed to analyse the use of agro-industrial waste as a low-cost substrate to the obtention of the amylase by the fungus Trichoderma stromaticum AM7 (CCMB617P) under solid state fermentation (SSF). The fungus was able to produce amylase using solely peach palm waste as a substrate (29.2 U/gds), however when a simplex centroid mixture design was applied to optimise the composition of the nitrogen source, peptone, and ammonium phosphate (7:3 w/w) in a total of 1% were added and an increase of the 45% (42.4 U/gds) was observed in relation to the activity initially obtained. The physicochemical characterisation of amylase revealed the optimum activity at pH 4.0 and temperature 50 °C to 60 °C. The amylase retained around 90% of its activity in a pH range from 4.0 to 7.0 and a temperature 40 °C to 70 °C, and 60% in a range from 80 °C to 100 °C, for up to 1 h. Amylase showed an increase in its activity with Al2+, Ag2+, and Co2+, however, the Cu2+negatively influenced the enzymatic activity. The enzyme was stable in the presence of SDS and β-mercaptoethanol. The cost-effective enzyme extraction and stable amylase highlight its strong potential in industry applications, especially in food processes.
1. Introduction
Amylases are enzymes that can be applied in various sectors, such as food, pharmaceuticals, textiles, chemicals, paper, and detergents, and therefore are among the most important biotechnological enzymes in the industry (Hasan et al. Citation2017; Ranke et al. Citation2020).
Amylases are glycosyl hydrolases that catalysts of starch hydrolysis reactions according to distinct ways divided into two classes. The endoamylases (e.g.: α-amylase, EC 3.2.1.1) cleave the interior of the starch molecule randomly to produce oligosaccharides of different chain lengths. The exoamylases (e.g. β-amylase, EC 3.2.1.2) and (glucoamylase, EC 3.2.1.3) cleave from the non-reducing ends of the starch, resulting in shorter products (Gupta et al. Citation2003; Souza et al. Citation2010).
In the food industry sector, the biggest market for amylase is in the production of hydrolysed starch whose final product is glucose and fructose, for example, in the production of high fructose corn syrups which are used in the sector of food and drink as a substitute for sucrose (Fonseca et al. Citation2021). In the bakery industry, the use of amylases positively influences bread volume, crumb texture, crust colour, and flavour development. In the fermentation process, supplementing the flour with α-amylases increases the fermentation rate and reduces the viscosity of the dough, resulting in an increase in volume and a better texture of the product, in addition to increasing the availability of fermentable sugar in the dough (Ruan et al. Citation2020). In this context, α-amylase contributes annually with about 25% to 30% of the total enzymes produced for industrial purposes (Paul et al. Citation2021).
Due to the significant increase in the demand for biocatalysts and to attend this demand, environmental microbial biodiversity can be a source to find new microorganisms that can be isolated and several enzyme-producing species with promising quality for industrial applicability (Roy et al. Citation2012). Among this biodiversity, research highlights that microbial enzyme, due to their ability to improve physical and chemical resistance to industrial environmental extremes, such as high temperature and pH, as well as to carry out different catalytic reactions, are favourite to meet the demands of the industry (Homaei et al. Citation2015).
Moreover, the enzymes’ costs come from a range of factors, such as the quantity produced, the production process, the expense of its recovery, and the degree of purity at which it will be marketed (Paludo et al. Citation2018). The amounts spent on processes that require enzymes to be developed can be reduced when a low-cost is employed in the production stage, for instance, with the use of agro-industrial substrates, such as coconut husk, cocoa bran, coffee husk, mushroom compound, among others (Coelho et al. Citation2008; Vriesmann et al. Citation2011; Santos et al. Citation2013; Gusmão et al. Citation2014; Paludo et al. Citation2018; Nakajima et al. Citation2018). Considering all these factors, studies are being carried out with the intention of reusing agro-industry by-products and, at the same time, with the production of enzymes through the use of microorganisms. According to Coelho et al. (Citation2001), such interest is justified because that involves low energy cost technology, with less environmental impact and use renewable raw materials. The residues from the food industry that involve large amounts of husks, flour, seeds, among others, most often discarded, are cheap organic materials that can be used as a substrate or carbon source in the fermentation process to obtain enzymes (Freitas et al. Citation2021).
The peach palm (Bactris gasipaes Kunth.) is a palm tree native from the Amazon region, currently cultivated in several Brazilian regions due to the growing interest mainly in the production of palm heart. Although its cultivation follows a series of technical recommendations aimed at sustainability, still large amounts of waste are generated after the final processing of the palm heart (Embrapa-Empresa Brasileira de Pesquisa Agropecuária Embrapa Florestas, Colombo PR Citation2021). The palm heart is found inside the peach-palm strain, the sheaths (shells) that cover the palm heart comprised between the leaf sheath and the median sheath and the part below the edible portion are the lignocellulosic by-products of the peach-palm corresponding to 83.5% of the weight of the discarded strain against 16.5% of the weight of the remaining edible portion. These by-products are discarded as solid agro-industrial waste (Bolanho et al. Citation2013; Spacki et al. Citation2022).
About 100 species of the filamentous fungus of the genus Trichoderma are described in the literature, with characteristics of fast growth, use of several types of substrates and capacity to tolerate adverse environments. Currently, its main economic interest is focused on its beneficial action of mycoparasites on phytopathogenic fungi to act as an agricultural biocontrol agent, as well as its production of enzymes of industrial interest (Poveda Citation2021). Hydrolytic enzymes are produced by Trichoderma spp. and widely used in the food industry (Shuster and Schmoll Citation2010). Amylases are most commonly produced by Aspergillus and Rhizopus species, however, fungi such as Trichoderma harzianum, T. asperellum, T. atroviride, and T. reesei have been reported as substantial producers of amylases (Pandey et al. Citation1999; Grujic´ et al. Citation2015; Abdel-Aty et al. Citation2019; Kalia et al. Citation2021).
In the early studies, Trichoderma stromaticum has been described as a good xylanases producer using peach-palm waste as substrate and its application in xylan degradation into xylo-oligosaccharides and in bread texture improvement (Carvalho et al. Citation2017) and also as a cellulases producer (Bezerra et al. Citation2021). However, until now no description of the production of amylases by the species Trichoderma stromaticum has been presented, and this is the first report of amylase production by Trichoderma stromaticum AM7 using peach-palm waste as substrate. Thus, the present work intends to contribute with biotechnological information regarding to T. stromaticum AM7 in the amylase production and enzyme characterisation aiming at the potential of its application in industrial processes.
2. Material and methods
2.1 Substrate and fungus growth
The peach palm waste (peels and pomace) was provided by the company Agroceres/Inaceres, Uruçuca, Bahia, Brazil. The waste was dried, ground and used as the substrate for fermentation. The fungus Trichoderma stromaticum AM7 used was from the Culture Collection of Microorganisms in Bahia (CCMB), Feira de Santana/BA (Carvalho et al. Citation2017). The strain reaction was made in potato dextrose agar medium, grown in the BOD at 25 °C for 5 days and then stored at 4 °C until use.
2.2 Solid state fermentation (SSF) and influence of nitrogen source addition
The SSF was carried out in 125 mL Erlenmeyer flasks with 2.5 g of dehydrated peach palm waste, moistened with 5 mL of tap water (TA) (2.0 mL/g) (Carvalho et al. Citation2018) and autoclaved at 121 °C for 15 min. Then, the flasks were inoculated with 106 spores/mL and incubated at 30 °C for 6 days.
The influence of different nitrogen sources added to peach palm waste on the production of amylases was tested. The experiments were performed in triplicate adding 0.5% of the nitrogen source: yeast extract, peptone, cocoa bean peel, soybean meal, ammonium nitrate, ammonium phosphate, and ammonium sulphate to the fermentation medium.
2.3 Planning of mixtures of nitrogen sources for the optimization of amylase production by T. stromaticum AM7 (CCMB617P)
To optimise the composition of the nitrogen source (1% of the total substrate), which is the proportion of cocoa peel, peptone, and ammonium phosphate (% in mass), for the AM7-based amylase production, a simplex centroid mixture design was applied to maximise the amylase activity obtained from the microbiologic synthesis. The mixture design consisted of 10 experiments, in which 2 were repetitions of the central point, the 10th was the evaluation of the centre point (0% of each component), and the factors were the percentage of cocoa peel, peptone, and ammonium phosphate (varying from 0% to 100%) and the response variable was the amylase activity (U/mL). presents the proportions evaluated on the mixture design and used to build up the response surface to calculate the optimal point for this system. The surface responses were obtained using linear, quadratic, and special cubic models, which were evaluated using ANOVA analysis. After this, to validate the model chosen, fermentations were carried out with the optimal point obtained from the equation of the response surface and with two more points that are not within the experimental domain, as shown in , and the theorical amylase activity was compared with the experimental amylase activity for each run. The mixture design experiments, surface responses, and ANOVA analysis were performed using STATISTICA 12.0 program (Stat Soft Inc., Tulsa, OK, USA), considering 95% as confidence level for the analysis.
Table 1. Mixture planning carried out different combinations of 1.0% nitrogen sources (cocoa bean peel, peptone, and ammonium phosphate) added to the peach palm waste under SSF by Trichoderma stromaticum AM7 (CCMB617P) at 30 °C for 6 days.
Table 2. Validation test of the proposed experimental model for the variable response of mixture proportions (cocoa bean peel, peptone, and ammonium phosphate) at a final concentration of nitrogen source at 1%.
2.3 Obtaining the enzyme extract with high amylase activity
After fermentation, the cultures were resuspended in sterile distilled water with stirring at 150 r/min at 20 °C for 30 min at a ratio (1:1 w/v) of 10 mL/g of dry substrate. Then, the material was filtered through gauze and centrifuged for 15 min at a rotation of 10,000× g at 4 °C and was considered as a crude enzymatic extract and submitted to analysis.
2.4 Determination of amylase activity
The determination of amylase activity was performed by the DNS (3, 5-dinitrosalicylic acid) method (Miller Citation1959) with the reaction mixture containing 125 µL of 1% soluble starch, 125 µL of 100 mmol/L sodium phosphate buffer (pH 7.0) and 125 µL of crude enzyme extract and incubated for 10 min at 50 °C. The reaction was stopped by the addition of 1.5 mL of DNS followed by boiling in the water bath for 5 min. After cooling, distilled water was added to the final volume of 5.0 mL. The standard curve was performed using glucose, and the amount of product released was determined in a spectrophotometer (Bel Spectro S-2000) at 540 nm. The substrate blank (substrate + buffer, without enzyme addition) and an enzyme blank (enzyme extract + buffer, without substrate addition) were performed and deducted from the test in the activity calculations. The enzyme unit was established as the amount of enzyme needed to release 1 µmol of glucose per minute and was expressed as a unit per gram of dry substrate (U/gds).
2.5 Determination of pH and temperature optimum and stability of amylase from T. stromaticum AM7 (CCMB617P)
The optimal pH was established by incubating the enzyme in different pH ranges from 3.0 to 10.0. For this, different 100 mmol/L buffer solutions were prepared: sodium acetate buffer (pH 3.0 to 5.0), sodium phosphate buffer (pH 6.0 to 9.0), and glycine buffer – NaOH (pH 10.0). To analyse the stability of the enzyme in relation to pH, the enzyme extract was incubated in buffer solutions with different pH for 1 h.
To determine the optimum temperature, enzymatic activities were measured under the standard conditions of the enzymatic assay carried out at different temperatures from 30 °C to 100 °C, at intervals of 10 °C. For the thermal stability of the enzyme, the enzymatic extract was incubated for 1 h at the different temperatures tested (30 °C to 100 °C). The residual enzymatic activities were measured under standard enzymatic assay conditions and expressed in percentage (%).
2.6 Effects of metal ions and chemical reagents on the amylase activity
To evaluating the effects of ions, chemical reagents and detergents, the enzymatic extract was incubated for 15 min at room temperature (25 °C ±2 °C) with a solution containing 5 mmol/L of the ions Cu2+ (copper), Al2+ (aluminium), Li2+ (lithium), Mg2+ (magnesium), Mn2+ (manganese), Ni2+ (nickel), Na2+ (sodium), Ca2+ (calcium), Co2+ (cobalt), Zn2+ (zinc), Ag2+ (silver), and reagents ethylenediamine tetracetic acid (EDTA), β-mercaptoethanol at 5 mmol/L and sodium dodecyl sulphate (SDS) at 1%. The control was done without the addition of any ion or reagent. The residual enzymatic activities were measured under standard enzymatic assay conditions, and the control enzyme activity was considered 100%.
3 Results and discussion
3.1 Optimization of the amylase production by T. stromaticum AM7 (CCMB617P)
To improve the yield of amylase production/activity of the proposed microbiological synthesis, different nitrogen sources were added individually to the peach palm waste fermentation medium, as can be seen in the results shown in . The presence of these nitrogen sources is important and has been influenced positively the amylase production by T. stromaticum AM7 (CCMB617P) since the amylase activity for the addition of all nitrogen source types on the fermentation medium was higher than in the control (only peach palm waste). Nonetheless, it can be observed that the addition of peptone, ammonium phosphate, and cocoa bean peel to the fermentation medium showed better results on the improvement of amylase production yield, being the amylase activity of 53.82 U/gds, 52.08 U/gds, and 48.9 U/gds, respectively, for each nitrogen source ().
Figure 1. Amylase production by Trichoderma stromaticum under SSF using peach-palm waste with different nitrogen sources at 0.5%: yeast extract (YE), peptone (PP), cocoa bean peel (CBP), soybean meal (SM), ammonium nitrate (AN), ammonium phosphate (AP), ammonium sulphate (AS) at 30 °C by 6 days. The data are presented as the mean of three experiments and mean values followed by different letters (a, b, c, d and e) differ statistically (P < 0.05).
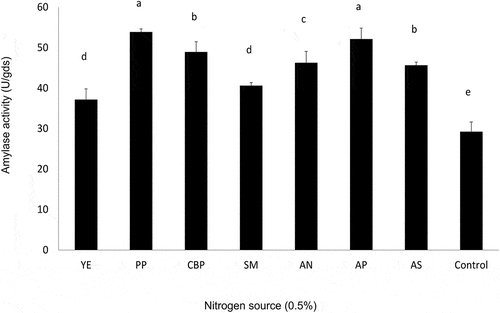
In this sense, aiming to study the synergistic effect of the nitrogen sources peptone, ammonium phosphate, and cocoa bean peel, as a mixture to compose the fermentation medium, and maximise the response of the amylase synthesis (amylase activity), a mixture simplex centroid design of experiment was performed and the surface response methodology was applied to determine the mathematical model that describes the relation between the response variable and the independent factors (nitrogen source proportions). This approach allows not only to determine the effect of pure nitrogen source added to the fermentation medium on the amylase activity but determine the optimal proportion of each factor evaluated on its response, performing for this the smallest number of experiments, and maximising the optimisation of the system (Costa et al. Citation2018). Cocoa bean peel was chosen over ammonium sulphate because it is also a low-cost agro-industrial residue for optimising amylase production.
In , the experimental matrix adopted on the mixture design is presented, as well as the values for response associated with each experiment. The amylase activity presented higher values for the proportion of 33.3% for the 3 nitrogen sources, considering the experimental point of the mixture design, indicating that the synergic effect of the different nitrogen sources on the mixtures is more important to the studied system than the pure components. However, the surface response obtained from this design can maximise this activity since the optimal proportion for the mixture can be determined from the model that describes its system (Pacheco et al. Citation2021).
With the data presented in , and to evaluate the results, surface responses were constructed using linear, quadratic, and special cubic mathematical models. An ANOVA analysis was applied to evaluate the performance of the models (Table S1), for the linear model, the adjusted R2 presented a value of 0.167, in contrast with 0.878 and 0.986 from quadratic and special cubic models, respectively. From adjusted R2 values, the fit between the mathematical model and the experimental data is evaluated, and the closer the adjusted R2 to 1, the better the fit considering the significant variables (Costa et al. Citation2018; Pacheco et al. Citation2021). The value of 0.167 indicates that a curvature is presented on the experimental data domain and is proved by observing the adjusted R2 of the higher-order polynomial models, which increase the adjusted R2 by increasing the polynomic order. Another important parameter evaluated is the mean squares of the error, which permits to infer about the capability of the model to minimise the error and maximise the response. For the linear model, this value was of 0.177 and for quadratic and special cubic models, the values were of 0.182 and 0.039, respectively.
The application of a special cubic model, for surface response construction (), showed the best performance compared to the other models since the adjusted R2 was of 0.986 and the quadratic sum of errors was the lowest (0.039). In this type of optimisation, the algorithm behind the mathematical modelling aims to determine the best model that represents the dependence of amylase activity to the proportion of nitrogen sources, and the smaller the mean errors, that is, the difference between the correct and expected values, the better the performance of the model to describe the system evaluated. It is possible to give information about the observed values against the predicted values obtained by the model, and for the special cubic model this graph (Figure S1), showed adequate fit and correlation, indicating the predictable power of the model. Because of that, the special cubic model was applied to the optimisation.
Figure 2. Response surface for mixture design of experiment that optimises, using special cubic model, the proportions between cocoa bean peel, peptone, and ammonium phosphate added to the peach palm waste under SSF by Trichoderma stromaticum AM7 (CCMB617P) at 30 °C for 6 days, for amylase synthesis.
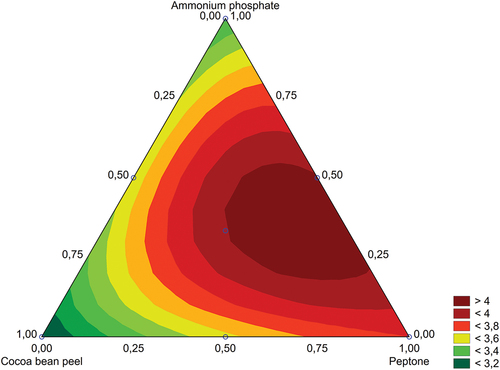
From the special cubic model surface response (), it was possible to determine the mathematical equation of the model, which shows us the parameters that influence on the response (amylase activity) for each pure factor (cocoa bean peel, peptone, and ammonium phosphate individually) and, also the parameters for the combination of each factor. From the equation of the special cubic model, the significance of each variable can be evaluated by the statistical testing of each respective parameter estimated by the response surface design. The special cubic equation is presented below, and the bold terms are the significant ones, considering the p-values associated with each of them:
Where Z is the amylase activity (U/gds), X is the cocoa bean peel (%), Y is the peptone (%), and W is the ammonium phosphate (%).
From the Eq. 1 it can be noted that all the parameters, either the pure factors or the combination of two factors, are positive, indicating that the effect of these terms enhances the amylase activity. Otherwise, the term that combines the factor X (cocoa bean peel) with the other factors, either Y and W individually or the combination of the three factors is null, which means that the synergistic or antagonistic effect of the combination of the cocoa bean peel and the other nitrogen sources were not significant for the mixture and the consequent enhancement of the amylase activity in this system. The combination of peptone and ammonium phosphate presented significant equation parameter and showed to be important for the amylase synthesis, by their addition of the peach palm waste substrate.
From the equation model, it was possible to obtain the contour graph that presents the optimal value for the synthesis optimisation (). In this way, the optimal values of each nitrogen source percentage to compose the peach palm waste medium were obtained. The desirable ranges of each component of this mixture are presented in . The best activities are related to the source of organic nitrogen (peptone) in a greater proportion and inorganic (ammonium phosphate) in a smaller proportion in a mixed form. For the cocoa bean peel, the influence of its use during fermentation was not expressive. The design of the optimum proportion for the final mixture was 70% peptone, 24.3% ammonium phosphate, and 5.7% cocoa bean peel. However, it is acceptable to use in a range comprising 0–6% cocoa bean peel, 64%–70% peptone, and 24%–30% ammonium phosphate () and this range is used in the validation experiment ().
Figure 3. Desirability function applied to mixture design to obtain the optimum values for cocoa bean peel, peptone, and ammonium phosphate proportion added to the peach palm waste under SSF by Trichoderma stromaticum AM7 (CCMB617P) at 30 °C for 6 days, for amylase synthesis.
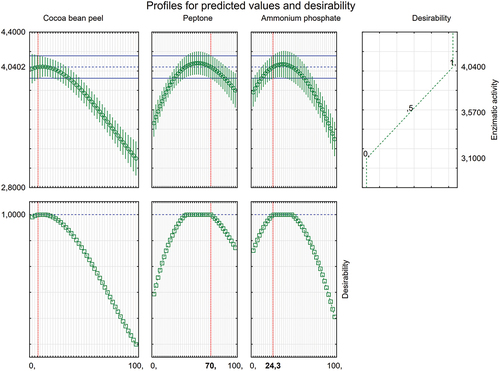
For the validation of the model, fermentations were carried out with the proportions of the mixtures of the optimum point in the 1.0% nitrogen source, within the experimental domain referring to the design, together with two other proportions that are not within the experimental domain. It was observed that the recovery percentage was as expected and the trial 3 used the optimal point nitrogen source values and gave the highest activity recovery (104.9%). The average between the three tests was 97% showing an excellent agreement between the results obtained experimentally and the theoretical ones. The mixture with the proportion of 70% peptone and 30% ammonium phosphate are optimised conditions for a greater range of enzymatic activity (42.4 U/gds) (). With the studies using different types of agro-industrial residues for amylase production, it can be inferred that residues are important raw materials, having great potential to produce enzymes and depending on their characteristics and compositions there is a great variation in enzyme production. As it was demonstrated by Aspergillus awamori producing amylase from different agro-industrial residues such as olive oil cake, which presented the highest activity of 230 U/gds, followed by banana peel (184 U/gds), corn cob (105 U/gds), rice straw (58.4 U/gds), and the lowest value obtained was with orange peel (1.05 U/gds) (Karan et al. Citation2017). The amylase production by Aspergillus niger using corn cob residue as substrate showed a higher value of 190.04 U/gds and with rice straw it reached a value of 63.82 U/gds. The lower value of enzymatic activity with the rice straw residue is because the husk particle is smaller and undergoes greater compaction, resulting in a decrease in aeration, affecting the efficiency of the microorganism in consuming the substrate and secreting the enzyme (Aliyah et al. Citation2017).
The use of these agro-industrial residues can be used both as solid support for microbial growth and their nutrition. However, to supply all the nutrients needed by microorganisms, the waste needs to be supplemented with macro and micronutrients, as nitrogen sources (Soccol et al. Citation2017).
Studies with Aspergillus niger, Penicillium chrysogenum, Microsporum, and Fusarium sp. tested different sources of nitrogen at 1% as urea, ammonium sulphate, yeast extract, and peptone. Among the sources, yeast extract and peptone provided the higher activities. For Aspergillus niger, the highest amylase production was with peptone supplementation (Sethi and Gupta Citation2015).
In the same way as the present study, it was observed that the addition of organic and inorganic nitrogen sources improved the enzyme production by A. niger LPB 28 and that the use of 1% urea and potassium nitrate added to solid fermentation increased 76% and 84% the enzyme activity when compared to fermentation without supplementation (Spier et al. Citation2006).
3.2 Physicochemical characterization of the amylase enzyme from T. stromaticum AM7
The amylase showed good activity in a pH range of 4.0 to 8.0 (), with little variation and with an optimal pH of 4.0, with an increase of approximately 50% of activity compared to pH 3.0. From pH 9.0 there was a drop of almost 70% in activity. According to the stability profile within the ranges of pH 4.0 to 7.0 the activities were maintained at approximately 90% and at pH 8.0 it kept above 70% of its residual activity showing to be highly stable (). These results demonstrate that amylase is able to work well over a wide range pH from acidic to basic.
The biochemical properties of microbial amylases can vary greatly depending on the producing microorganism. However, α-amylases are one of the most commonly used and generally have greater specificity for the starch substrate, a molecular weight of 50–60 kDa, the optimum pH in an acidic to basic range between 2.0 and 12.0 with greater stability in a range from 4.0 to 11.0 of pH, the optimum minimum temperature is between 25 °C and 30 °C up to a maximum of 100–130 °C and as it is a metalloenzyme, it has a strong affinity for the Ca2+ ion (Gupta et al. Citation2003).
For industrial applications in the food sector, amylases with a more acidic profile have a better application perspective, since a stable amylase at a more acidic pH overcomes the need to adjust the pH to 4.5 during the saccharification process, with that the formation of by-products will be reduced, is what highlights Wu et al. (Citation2018) who maintained the stability of their amylase from Bacillus licheniformis B4–423 at about 76% activity when incubated at pH 4.0 for 1 h and its ideal activity at pH 5.0.
The optimum temperature of the amylase found was between 50 °C and 60 °C (). However, the enzyme maintained its activity above 90% at higher temperatures. When thermostability was analysed, the enzyme was significantly stable, retaining above 80% activity in the range 20 °C to 60 °C and around 60% in the range 80–100 °C after 1 h of incubation at these temperatures ().
Figure 5. Effect of temperature on Trichoderma stromaticum AM7 amylase activity (a) and stability (b).
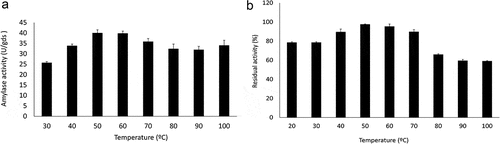
Studies with A. niger amylase showed that the enzyme has its optimum pH at 5.0 and at a temperature of 50 °C and that it maintained 85% of its activity after 1 h of incubation. However, at 70 °C, 90 °C, and 100 °C the activity was completely inactivated (Hernadéz et al. Citation2006). The amylase from Coprinus comatus also showed an increase in amylase activity at 50 °C, however at 70 °C the enzyme lost 92% of its activity (Paludo et al. Citation2018). Therefore, the enzyme amylase from T. stromaticum AM7 has favourable conditions in acidic to neutral conditions and at high temperatures, revealing a potential application for starch processing in the food industry.
For the analysis of the influence of the ions on the amylase activity, we observed that the Ag2+, Co2+, and Al2+ ions showed a positive influence, increasing the enzyme activity by 193%, 46%, and 24%, while the Ni2+, Zn2+, and the ß-mercaptoethanol practically did not influence its activity. However, Cu2+ strongly inhibited the enzyme activity, decreasing by 70% and Na2+ weakly inhibited the amylase activity (39%) ().
Table 3. Effect of metal ions and reagents on the enzymatic activity of the amylase of the Trichoderma stromaticum AM7 (CCMB617P).
Similar to the present work, studies with Aspergillus oryzae IFO-30103 demonstrated a 46% increase in amylase activity in the presence of Co2+ and a 20% inhibition with the chelator EDTA. However, the SDS surfactant inhibited 38% of the enzymatic activity (Dey and Banerjee Citation2015), while for T. stromaticum AM7 the enzyme was able to retain 98% of the amylase activity with this compound. Wu et al. (Citation2018) showed similar results in relation to amylase from Bacillus licheniformis B4–423, which presented a relative activity of 85% with SDS, as well as an average relative activity of 81% with EDTA, while that with reducing agents β-mercaptoethanol the relative activity was slightly increased. According to these same authors, the stability of amylase against SDS is rarely reported and has great potential for industrial applicability, especially in the textile and detergent industries.
Most of the α-amylases require Ca2+ ions as a cofactor, as they have calcium-binding sites for stability, enzyme regulation, and improvement of substrate-enzyme affinity, as was shown for thermostable α-amylase of the Thermotoga petrophila, a metalloenzyme Ca2+ dependent, and this characteristic is unusual when compared to thermophilic ones that are established for not having a binding site for Ca2+ (Hameed et al. Citation2017). On the other hand, the amylase of the present work demonstrated the specificity of being an independent Ca2+ ion suffering a slight inhibition of 10% and of having a potential thermotolerant structure due to this characteristic.
The Ag2+ ions are mainly reported to be destabilising the disulphide bonds present in proteins, as they act as modifiers of the thiol or sulphhydryl group (Hameed et al. Citation2017). However, in the present work, the results demonstrate an increase of 193% in enzymatic activity in the presence of the Ag2+ ion. The α-amylase activity of Coprinus comatus was increased by 43% for the Mg2+ ion and was repressed by the Ca2+ and Cu2+ ions. In glucoamylase, it increased in the presence of the Mn2+ ion more than 168% and had a 68% repression by the Cu2+ ion (Paludo et al. Citation2018), corroborating with the repression of the Cu2+ ion of 71% in the present work.
4 Conclusion
The fungus T. stromaticum AM7 was efficient in producing amylase using peach palm waste as low-cost substrate in solid state fermentation which simplifies and makes fermentation economically viable for this enzyme production. After the addition of nitrogen sources as peptone and ammonium phosphate, the amylase production increased by 45% by T. stromaticum AM7. The biochemical properties of the amylase showed its stability to acidic pH (4.0) and high temperatures up to 50–60°C. In addition, the enzyme was little influenced by the presence of ions and denaturing agents, which gives the characteristic of the T. stromaticum AM7 amylase promising for applications mainly in the food sector.
Author contributions
All authors contributed to the study conception and design. Conceptualisation: Stephanie Alexandrina Gillet and Andréa Miura da Costa; Methodology: Stephanie Alexandrina Gillet and Murillo Nascimento Silva; Formal analysis: Stephanie Alexandrina Gillet, Erik Galvão Paranhos da Silva and Pedro dos Santos Moreau; Investigation: Andréa Miura da Costa, Ana Paula Trovatti Uetanabaro, Elck Almeida Carvalho and Stephanie Alexandrina Gillet; Funding acquisition: Ana Paula Trovatti Uetanabaro and Andréa Miura da Costa; Supervision: Andréa Miura da Costa and Erik Galvão Paranhos da Silva; Writing – reviewing and editing: Ana Paula Trovatti Uetanabaro, Andréa Miura da Costa, Elck Almeida Carvalho, Erik Galvão Paranhos da Silva, Pedro dos Santos Moreau and Stephanie Alexandrina Gillet. All authors read and approved the final manuscript.
Supplemental Material
Download TIFF Image (712.1 KB)Supplemental Material
Download MS Word (15.8 KB)Acknowledgments
The authors would like to thank the Agroceres/Inaceres company (Uruçuca city, Bahia, Brazil) for supplying the peach-palm waste, the Coordenação de Aperfeiçoamento de Pessoal de Nível Superior (CAPES) for assistance and the Fundação de Amparo à Pesquisa do Estado da Bahia – FAPESB for Gillet S. A. scholarship.
Disclosure statement
No potential conflict of interest was reported by the author(s).
Supplementary material
Supplemental data for this article can be accessed online at https://doi.org/10.1080/21501203.2023.2276728
Additional information
Funding
References
- Abdel-Aty AM, Bassuiny RI, Barakat AZ, Mohamed SA. 2019. Upgrading the phenolic content, antioxidant and antimicrobial activities of garden cress seeds using solid-state fermentation by Trichoderma reesei. J Appl Microbiol. 127:1454–1467. doi: 10.1111/jam.14394.
- Aliyah A, Alamsyah G, Ramadhani R, Hermnsyah H. 2017. Production of α-amilase and β-glucosidase from Aspergillus niger by solid state fermentation method on biomass waste substrates from rice husk, bagasse, and corn cob. Enrgy Proced. 136:418–423. doi: 10.1016/j.egypro.2017.10.269.
- Bezerra CO, Carneiro LL, Carvalho EA, Chagas TP, Carvalho LR, Uetanabaro APT, da Silva GP, da Silva EGP, Costa AM, da Silva GP, et al. 2021. Artificial intelligence as a combinatorial optimization strategy for cellulase production by Trichoderma stromaticum AM7 using peach-palm waste under solid-state fermentation. Bioenerg Res. 14(4):1161–1170. doi: 10.1007/s12155-020-10234-4.
- Bolanho B, Danesi E, Beléia A. 2013. Peach palm (bactris gasipaes Kunth) characterization and the potential of by-products flour processing. Food Sci Technol Res. 19(6):1061–1069. doi: 10.3136/fstr.19.1061.
- Carvalho E, Góes L, Uetanabaro APT, Da Silva E, Rodrigues L, Pirovani C, Costa AM. 2017. Thermoresistant xylanases from Trichoderma stromaticum: application in bread making and manufacturing xylo-oligosaccharides. Food Chem. 221:1499–1506. doi: 10.1016/j.foodchem.2016.10.144.
- Carvalho E, Nunes L, Goes L, Silva E, Franco M, Gross E, Uetanabaro APT, Costa AM. 2018. Peach-palm (bactris gasipaes Kunth.) waste as substrate for xylanase production by Trichoderma stromaticum AM7. Chem Eng Commun. 205(7):975–985. doi: 10.1080/00986445.2018.1425208.
- Coelho MAZ, Leite SGF, Rosa MF, Furtado AAL. 2001. Aproveitamento de resíduos agroindustriais: Produção de enzimas a partir da casca de coco verde. Boletim CEPPA. 19:33–42. doi: 10.5380/cep.v19i1.1220.
- Coelho MB, Ribeiro B, Salgado A. 2008. Tecnologia Enzimática. Rio de Janeiro: editora EPUB. p. 288.
- Costa VC, Silva EGP, Lima DC, Franco M, Jesus RM, Bezerra MA, Amorim FA, Banerjee R. 2018. Use of mixture design with minimal restrictions to optimize an extraction procedure employing diluted acids assisted by ultrasound and Microwave for Nutrient element Determination in vegetal samples. J Braz Chem Soc. 29:1189–1198. doi: 10.21577/0103-5053.20170214.
- Dey T, Banerjee R. 2015. Purification, biochemical characterization and application of α-amylase produced by Aspergillus oryzae IFO-30103. Biocatal Agric Biotechnol. 4(1):83–90. doi: 10.1016/j.bcab.2014.10.002.
- Embrapa-Empresa Brasileira de Pesquisa Agropecuária Embrapa Florestas, Colombo PR. 2021. Accessed on 10 August 2021. https://www.embrapa.br/florestas/transferencia-de-tecnologia/pupunha/tema
- Fonseca M, Sterling R, Lafuente R, Tardioli P. 2021. Optimization of simultaneous saccharification and isomerization of dextrin to high fructose syrup using a mixture of immobilized amyloglucosidase and glucose isomerase. Catal Today. 362:175–183. doi: 10.1016/j.cattod.2020.03.021.
- Freitas LC, Barbosa JR, Costa ALC DA, Bezerra FWF, Pinto RHH, Carvalho Junior RN. 2021. From waste to sustainable industry: how can agro-industrial wastes help in the development of new products? Resour Conserv Recy. 169:105466. doi: 10.1016/j.resconrec.2021.105466.
- Grujic´ M, Dojnov B, Potocnik I, Duduk B, Vujcic´ Z. 2015. Spent mushroom compost as substrate for the production of industrially important hydrolytic enzymes by fungi Trichoderma spp. And Aspergillus niger in solid state fermentation. Int Biodeter Biodegr. 104:290–298. doi: 10.1016/j.ibiod.2015.04.029.
- Gupta R, Mohapatra H, Goswami VK, Chauhan B. 2003. Microbial α-amylases: Biotechnological Perspective. Process Biochem. 1–18. doi: 10.1016/S0032-9592(03)00053-0.
- Gusmão RO, Ferraz LM, Rêgo APB, Assis FGV, Leal PL. 2014. Produção de enzimas por Aspergillus spp. Sob fermentação em estado sólido em casca de café. Sci Plena. 10(11):116–202.
- Hameed U, Price I, Haq I, Ke A, Wilson D, Mirza O. 2017. Functional characterization and crystal structure of thermostable amylase from Thermotoga petrophila, reveals high thermostability and an unusual form of dimerization. Biochim Biophys Acta BBA - Proteins Proteom. 1865:1237–1245. doi: 10.1016/j.bbapap.2017.06.015.
- Hasan M, Marzan L, Hosna A, Hakim A, Azad A. 2017. Optimization of some fermentation conditions for the production of extracellular amylases by using chryseobacterium and Bacillus isolates from organic kitchen wastes. J Genet Eng Biotechnol. 15(1):59–68. doi:10.1016/j.jgeb.2017.02.009.
- Hernadéz M, Rodríguez M, Guerra N, Rosés R. 2006. Amylase production by Aspergillus niger in submerged cultivation on two wastes from food industries. J Food Eng. 73:93–100. doi: 10.1016/j.jfoodeng.2005.01.009.
- Homaei A, Ghanbarzadeh M, Monsef F. 2015. Biochemical features and kinetic properties of α-amylases from marine organisms. Int J Biol Macromol. 83:306–314. doi: 10.1016/j.ijbiomac.2015.11.080.
- Kalia S, Bhattacharya A, Prajapati S, Malik A. 2021. Utilization of starch effluent from a textile industry as a fungal growth supplement for enhanced α-amylase production for industrial application. Chemosphere. 279:130554. doi: 10.1016/j.chemosphere.2021.130554.
- Karan E, Wahab W, Saleh S, Hassan M, Kansoh A, Esawy M. 2017. Production, immobilization and thermodynamic studies of free and immobilized Aspergillus awamori amylase. Int J Biol Macromol. 102:694–703. doi: 10.1016/j.ijbiomac.2017.04.033.
- Miller L. 1959. Use of dinitrosalicylic acid reagent for determination of reducing sugar. Anal Chem. 3(31):426–428. doi: 10.1021/ac60147a030.
- Nakajima VM, Soares FEF, Queiroz JHQ. 2018. Screening and decolorizing potential of enzymes from spent mushroom composts of six different mushrooms. Biocatal Agric Biotechnol. 13:58–61. doi: 10.1016/j.bcab.2017.11.011.
- Pacheco CSV, Costa FS, Guedes WN, Jesus MS, Chagas TP, Santos AM, Lima DC, Silva EGP. 2021. Application of mixture design and Kohonen Neural Network for Determination of macro- and microelement in mullet (mugil cephalus) by MIP OES. Food Anal Method. 14:1239–1249. doi: 10.1007/s12161-021-01969-7.
- Paludo L, Frantz S, Ançay R, Stustz H, Dantas T, Spier M. 2018. Optimization, kinetic and bioprocess parameters of amylases production from Coprinus comatus under submerged culture using starch-based simple medium: Partial enzyme characterization. Biocatal Agric Biotechnol. 16:529–537. doi: 10.1016/j.bcab.2018.09.022.
- Pandey A, Selvakumar P, Soccol C, Nigam P. 1999. Solid state fermentation for the production of industrial enzymes. Curr Sci. 77(1):149–162.
- Paul JS, Gupta N, Beliya E, Tiwari S, Jadhav SK. 2021. Aspects and recent trends in microbial α-amylase: a review. Appl Biochem Biotechnol. 193(8):2649–2698. doi:10.1007/s12010-021-03546-4.
- Poveda J. 2021. Trichoderma as biocontrol agent against pests: new uses for a mycoparasite. Biol Control. 159:104634. doi: 10.1016/j.biocontrol.2021.104634.
- Ranke FF, Shinya T, Figueiredo F, Núñez EG, Cabral H, De Oliva Neto P. 2020. Ethanol from rice byproduct using amylases secreted by Rhizopus microsporus var. Oligosporus. Enzyme partial purification and characterization. J Environ Manage. 266:110591. doi: 10.1016/j.jenvman.2020.110591.
- Roy J, Rai S, Mukherjee A. 2012. Characterization and application of a detergent-stable alkaline α-amylase from Bacillus subtilis strain AS-s01a. Int J Biol Macromol. 50(1):219–229. doi:10.1016/j.ijbiomac.2011.10.026.
- Ruan Y, Xu Y, Zhang W, Zhang R. 2020. A New Maltogenic amylase from Bacillus licheniformis R-53 significantly improves bread quality and extends shelf life. Food Chem. 344:128599. doi: 10.1016/j.foodchem.2020.128599.
- Santos TC, Rocha TJO, Oliveira AC, Abreu-Filho G, Franco M. 2013. Aspergillus niger como produtor de enzimas celulolíticas a partir farelo de cacau (Theobroma cacao). Arq Inst Biol São Paulo. 80(1):65–71. doi: 10.1590/S1808-16572013000100010.
- Sethi S, Gupta S. 2015. Isolation, characterization and optimization of cultural conditions for amylase production from fungi. J Global Biosci. 4(9):3356–3363.
- Shuster A, Schmoll M. 2010. Biology and biotechnology of Trichoderma. Appl Microbiol Biotechnol. 87:787–799. doi: 10.1007/s00253-010-2632-1.
- Soccol C, Costa E, Letti L, Karp S, Woiciechowski A, Vandenberghe L. 2017. Recent developments and innovations in solid state fermentation. Biotechnol Res Innov. 1:52–71. doi: 10.1016/j.biori.2017.01.002.
- Souza PMD, Magalhães PDO, de Oliveira P. 2010. Application of microbial α-amylase in industry-A review. Braz J Microbiol. 41:850–861. doi: 10.1590/S1517-83822010000400004.
- Spacki DECK, Corrêa RCG, Uber TM, Barros L, Ferreira ICFR, Peralta RA, De Fátima Peralta Muniz Moreira R, Helm CV, De Lima EA, Bracht A, et al. 2022. Full exploitation of peach palm (bactris gasipaes Kunth.): state of the art and perspectives. Plants. 11:3175. doi: 10.3390/plants11223175.
- Spier M, Woiciechowski A, Vandenbergh L, Soccol C. 2006. Production and characterization of amylases by Aspergillus niger under solid state fermentation using agro industrials products. Int J Food Eng. 2(3). doi: 10.2202/1556-3758.1116.
- Vriesmann LC, Amboni RDMC, Petkowicz CLO. 2011. Cacao pod husks (theobroma cacao L.): composition and hot-water-soluble pectins. Ind Crops Prod. 34:1173–1181. doi: 10.1016/j.indcrop.2011.04.004.
- Wu X, Wang Y, Tong B, Chen X, Chen J. 2018. Purification and biochemical characterization of a thermostable and acid-stable alpha-amylase from Bacillus licheniformis B4-423. Int J Biol Macromol. 109:329–337. doi: 10.1016/j.ijbiomac.2017.12.004.