Abstract
Mobile instructional spaces such as retrofit buses, customized trucks or trailers, and repurposed shipping containers are innovative, unique venues for teaching and learning. STEM-focused mobile instructional spaces have emerged as vehicles for providing students and teachers with high-quality integrated STEM learning experiences that emphasize professional practices and 21st-century skills alongside a range of STEM content. In this article, we describe three best practices (creating a shared culture, fostering agency, and offering multiple pathways for engagement) for operating a STEM-focused mobile instructional space with K–12 students and teachers. We developed these best practices based on our own extensive work with two STEM-focused mobile instructional spaces, the BioBus and the MakerTruck. These practices have the potential to inform the work of formal and informal STEM educators who also operate mobile instructional spaces. Next steps for cementing the unique role STEM-focused mobile instructional spaces play in bridging the gap between in-school and out-of-school learning are discussed.
Mobile instructional spaces are unique venues for teaching and learning. Examples of mobile instructional spaces include retrofit buses, customized trucks or trailers, and repurposed shipping containers. Importantly—and no matter what they look like—mobile instructional spaces can move from place to place, allowing organizations to provide instruction to students, professional development to teachers, and engaging activities to community members who might not otherwise have the resources or opportunities to participate in such experiences. Because they can travel from place to place and because the staff who operate these spaces often have expertise working with a range of stakeholders and individuals of a variety of ages, mobile instructional spaces are uniquely positioned to bridge the gap between in-school and out-of-school learning. Many mobile instructional spaces also represent promising venues for exposing students, teachers, and community members to science, technology, engineering, and mathematics (STEM). As a result, these mobile instructional spaces have opportunities to inspire the young people with whom they work with a zest for STEM learning as well as equip them with skills, practices, and content knowledge they need to become competent STEM practitioners in the future.
In this article we describe several best practices for operating STEM-focused mobile instructional spaces. We begin by explaining the context for creating these spaces. Next, we briefly provide information on how some of these mobile instructional spaces operate in the wild. Finally, we take a closer look at two such mobile instructional spaces and outline the best practices they developed and deployed to fulfill their specific missions. Our overarching goal is to shine a light on STEM-focused mobile instructional spaces, celebrating the work they have done in the past and informing the work they may do in the future. Our work is guided by the question: How can we use mobile instructional spaces to improve STEM learning experiences for K–12 students and teachers?
Context for creating STEM-focused mobile instructional spaces
For the past decade, there has been a trend toward enacting integrated STEM instruction in schools (Moore and Smith Citation2014; NRC Citation2014). Although there is no universally agreed-upon vision for what integrated STEM looks like, two principles generally undergird most notions of integrated STEM instruction. The first is that teachers make interdisciplinary connections to STEM content—as opposed to treating STEM subjects as independent and siloed (Bybee Citation2010). The second is that integrated STEM instruction goes beyond simply teaching content by focusing on exposing students to the practices, dispositions, and twenty-first-century skills that STEM professionals use in their everyday work (Roehrig et al. Citation2021) and that are central to documents such as the Next Generation Science Standards (NGSS).
As integrated STEM instruction gained popularity, teachers needed resources and opportunities to infuse integrated STEM instruction into their own lessons. While part of this need was filled by individuals and corporations developing tools and materials for teachers to use in their classrooms, another part was filled by universities, out-of-school STEM learning venues, and not-for-profit organizations who either developed or already operated mobile instructional spaces that address this need. These mobile instructional spaces typically focused broadly on STEM concepts and facilitated integrated STEM learning opportunities for teachers and students alike, effectively bridging the gap between inside- and outside-of-school STEM learning.
Mobile instructional spaces can be particularly helpful for teachers and students engaging in integrated STEM instruction for several reasons. First, teachers may have limited resources and materials at their schools; mobile instructional spaces can address this by bringing materials, tools, and technology to teachers on site. Second, teachers have a limited amount of time to seek out professional training. Mobile instructional space staff eliminate the need for teachers to learn how to use and troubleshoot complex tools and equipment.
The STEM learning experiences provided by mobile instructional spaces may keep students more interested as they represent unique opportunities for learning STEM content and practices outside of the classroom. These experiences can also challenge students to creatively display what they have learned, increasing the ways in which students can demonstrate what they know. Finally, these experiences give students access to new tools and techniques that are otherwise unavailable to them.
STEM-focused mobile instructional spaces in the wild
Universities, out-of-school STEM learning venues, private companies, school districts, and not-for-profits have all created mobile instructional spaces meant to support the teaching and learning of STEM-related content and practices. For example, the Perot Museum of Nature and Science, in Dallas, Texas, operates a mobile instructional space called the TECH Truck. The TECH Truck’s mission is to partner with community centers to provide underrepresented youth and their caregivers with STEM-focused experiences that inspire them and foster creativity and confidence (Perot Museum of Nature and Science Citation2023). This is just one example of how to use a mobile instructional space. There are many other mobile instructional spaces developed to fulfill a wide variety of STEM-related teaching and learning goals by an array of organizations. Although listing these organizations, identifying each of their missions, and describing the range of activities offered by their mobile instructional spaces is outside the scope of this paper, it is important to note that it is possible to do this important work in different ways and with a different mission at the core. However, there are several considerations for deploying STEM-focused mobile instructional spaces in the wild. In the sections that follow, we use our experiences from two mobile instructional spaces that we have been a part of, the BioBus and the MakerTruck, to describe three best practices (creating a shared culture, fostering agency, and offering multiple pathways for engagement) for operating a STEM-focused mobile instructional space with K–12 students and teachers.
The BioBus as a mobile instructional space
BioBus is a New York City–based nonprofit, founded by Dr. Ben Dubin-Thaler. Upon completing his PhD in cell biology, Dr. Ben purchased a 1974 San Francisco transit bus from Craigslist with the ambitious vision of gutting the bus and retrofitting it as an accessible mobile microscope lab (). When the BioBus launched in New York City in 2008, Dr. Ben and his colleague Sarah Weisberg were intent on using it to expose young people attending schools in underserved communities to the joys of scientific discovery. Today, BioBus’s mission has changed little since its inception, and it continues to focus on delivering high-quality, authentic science learning experiences to young people historically excluded from the scientific community. Since its inception, BioBus’s capacity to serve its intended audience has multiplied. Supported by a staff of community scientists, facilities crew, and back-office administrators, BioBus uses two mobile instructional spaces as well as its brick-and-mortar community labs to serve tens-of-thousands of students each year across the New York City metropolitan area and New England. In the sections that follow we explain how the BioBus staff creates a shared culture, fosters agency, and offers multiple pathways for engagement for K–16 students to learn and do science in a joyful way.
Best practice 1: Creating a shared culture
To create a shared culture, BioBus community scientists design learning experiences that create a “third space” in which young people (particularly those traditionally excluded from the scientific community) can participate in scientific discovery. The idea of a third space was first conceptualized by Homi Bhabha (Citation1994) to examine the social aspects of culture and later extended by urban theorist Edward Soja (Citation1996) to include physical spaces. More recently, the concept of third space has been applied by myriad education researchers to study science learning experiences both within and outside of the classroom. Creating a third space—which is essentially an intermediate space between the home and a more formal place of learning like school—has the potential to resolve cultural tensions and enhance scientific learning experiences. In practice, achieving a third space on the BioBus means designing the physical spaces of BioBus to help students feel welcome, comfortable, and excited to make scientific discoveries (). These feelings are further encouraged and facilitated by the setting in which the students use the BioBus (i.e., parked outside of a student’s school during their normal science class), the tools and technology in the interior space (i.e., research-grade microscopes attached to large digital displays), and the people (i.e., BioBus community scientists who interact with students and their teachers). In combination, these physical and social constructs have been documented to create enjoyable scientific learning experiences and demonstrated to improve student attitudes toward science (Fox Citation2015).
Best practice 2: Fostering agency
BioBus community scientists intentionally design learning experiences to foster student agency. One way they do this is by inviting students to join a community of practicing scientists who encourage and facilitate students making their own scientific discoveries. Holding the focus knobs of a stereoscope stationed in the front of the BioBus, students literally grip the controls of their learning. During a typical BioBus visit, community scientists arrange for students to examine Daphnia, a small freshwater crustacean, whose translucent shell and alien-like appearance transfix students to the screen mounted above their stereoscope (). With help from BioBus staff, students use evidence to analyze their discoveries. For example, the discovery process may begin with students making observations like, “Mine is spinning around!” or “I can see this one’s heart beating!” Soon after, observations turn into questions that are subsequently answered with help from BioBus community scientists. For instance, a student may ask, “What does Daphnia eat?” only to be guided by a BioBus community scientist to identify where they believe the organism’s mouth is and then encouraged to observe how the Daphnia’s antler-like appendages move algae toward it.
Student agency is further encouraged at another microscope station located in a small classroom at the back of the BioBus. Within this space, students can design simple experiments under the guidance of BioBus community scientists. For example, a BioBus community scientist may remind students of the activity at the front of the BioBus during which they viewed the Daphnia’s heart beating. The BioBus scientist can then use questioning to lead students to wonder how their own hearts are impacted by their diet. In turn, this conversation may prompt students to ask questions like, “What impact do stimulants like caffeine and depressants like alcohol have on heart rate?” These questions may then lead to an experiment in which students expose Daphnia to chemicals like caffeine and alcohol and subsequently observe the speed at which the Daphnia’s translucent heart beats. While this example of student agency in the design of a guided-inquiry experiment is narrow, its message is nonetheless powerful: Students are encouraged and empowered by those within the scientific community to ask and answer questions relevant and meaningful to their own interests and lived experience.
Best practice 3: Offering multiple pathways for engagement
Although the time students spend on the BioBus is brief (typically a single class period), student learning can be extended by teachers in the days before and after the visit by using supplemental materials provided by BioBus staff. For those students whose interest in scientific discovery is piqued by their time aboard the BioBus, specialized programs allow them to continue their participation. For example, “Explore” programs, primarily geared toward middle school–age students, can be experienced in after-school classes or at a summer camp hosted at BioBus’s community lab space. Participants in Explore programs have access to the tools and materials on the BioBus, are continually exposed to and welcomed into the scientific community, and meaningfully participate in a community of practicing scientists ().
Figure 4. A “Pursue” BioBus Jr. Scientist intern shares their original research with the next generation of scientists at the BioBus community lab space.
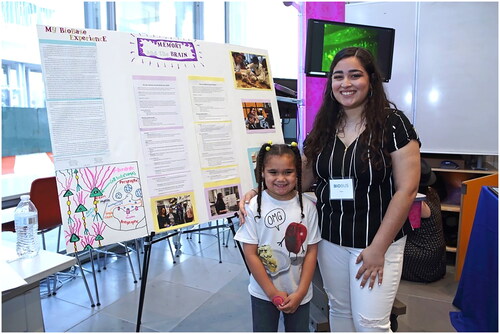
For students who successfully complete an Explore program or want to further extend their interactions with the BioBus, the “Pursue” pathway exists as an option to deepen scientific knowledge and hone research skills. Pursue, which is geared toward high school and college-age students, includes paid internships in which participants design, carry out, and present the results of their own experiments. Working under the guidance of a community scientist, BioBus Jr. Scientists enrolled in the Pursue pathway complete a semester or yearlong course of study. Past projects completed by students have ranged from examining the effects of microplastics on the now very familiar Daphnia to discovering what types of viruses are circulating in urban wild bird populations. This latter project serves as yet another pathway for further engagement within the scientific community via partnerships with the Icahn School of Medicine at Mount Sinai and the Wild Bird Fund. Indeed, programming and science research experiences like those provided through the Pursue pathway have demonstrated the ability to increase students’ science identity (Heydari Citation2020) and align closely with BioBus’s mission to address inequities in the sciences.
The MakerTruck as a mobile instructional space
The MakerTruck, a former bread delivery truck turned mobile makerspace, was previously operated by the Southern Methodist University (SMU) Maker Education Project. Part of the mission of the MakerTruck was to provide professional development free of charge to practicing teachers so that they could develop creative confidence, become proficient using a variety of tools and technologies, and learn valuable pedagogical techniques that would assist them orchestrating maker-based activities in their own school makerspaces (). The MakerTruck staff’s hope was that after participating in professional development offered by the MakerTruck, teachers would be motivated and empowered to enact similar activities with their own students—and by extension—their students would build their own skills and confidence as well as have opportunities to create personalized artifacts by engaging in maker-based activities. In the sections that follow we explain how the MakerTruck staff created a shared culture, fostered agency, and offered multiple pathways for engagement for K–12 teachers to take up maker education in their own classrooms.
Best practice 1: Creating a shared culture
Because culture often shapes teachers’ perceptions of an activity, creating a shared positive culture around an activity is critically important for mobile instructional space staff interested in working with practicing teachers. One way in which the MakerTruck’s staff created a shared culture around STEM learning was to plan teacher professional development with the intention that it would be joyful, broadly accessible, promote meaningful collaboration among teachers, and provide opportunities for teachers to learn through experimentation and failure, all of which are core tenants of the maker movement in education (Martin Citation2015). The MakerTruck staff found that creating a shared culture increased the likelihood that teachers would engage with activities and attempt challenging and outside-of-the-box maker-based activities in their own classrooms and makerspaces.
When planning professional development for teachers, the MakerTruck staff also intentionally embraced forms of pedagogy that value people working together to build skills and knowledge while simultaneously building a physical artifact. Specifically, they adopted a constructionist approach when working with teachers. Constructionism is the theory that individuals build knowledge while building tangible objects (Papert Citation1991). Embracing a constructionist approach meant that they were accountable for creating hands-on, engaging professional development sessions in which teachers would leave with a personalized product that they could proudly showcase to their colleagues and students, and demonstrate what they learned during the professional development. Finally, adopting a constructionist approach meant that the teachers who participated in professional development had the opportunity to build the very same skills their own students would need to complete similar projects in the future. Doing this made teachers more confident and prepared them to manage the issues and challenges they encountered when enacting similar activities with their own students.
Best practice 2: Fostering agency
When working with teachers, the MakerTruck staff fostered agency in two ways. First, they sought to leverage teachers’ knowledge, skills, and expertise. They did this by working in consultation with educators to design professional development experiences that allowed teachers to connect to the content they regularly taught in classrooms. They also intentionally built in time for reflection during professional development. During this time, teachers connected their new experiences to the content and classes they had taught in the past. This allowed teachers to visualize how to integrate new experiences into their future classroom instruction in a way that authentically connected to their curriculum. In some cases, MakerTruck staff also developed and distributed curriculum resources for teachers so that they had concrete materials they could use in their own classrooms (). This greatly reduced the planning workload for teachers, and MakerTruck staff quickly learned that leaving teachers with resources such as lesson plans vastly increased the chances they would enact similar projects after the MakerTruck departed.
The second way MakerTruck staff fostered teacher agency during professional development sessions was to deemphasize the importance of needing high-tech tools for high-quality makerspace instruction. As a rule, MakerTruck staff used tools and technologies that were inexpensive, web-based, and easy to troubleshoot—increasing the likelihood that teachers would use them with their own students. At the same time, MakerTruck staff also highlighted that high-quality makerspace instruction is process driven rather than tool driven. By emphasizing processes rather than tools, teachers were empowered with the belief that they could plan and enact engaging maker-based lessons without needing access to complex (and often expensive) tools and machinery.
Best practice 3: Offering multiple pathways for engagement
Offering teachers more than one way to engage with the MakerTruck over time increased the MakerTruck’s impact. However, at the beginning of a partnership, staff typically followed the same model for deploying the MakerTruck. In this model staff sought to establish a positive relationship with a school by attending an event at their invitation (). An example of one such event is “STEM night.” During STEM night, teachers at a school organize a set of STEM-focused activities for students, parents, and community members to participate in so that they can learn in a fun, non-intimidating way. When attending STEM nights with the MakerTruck, staff performed simple activities where participants walked away with a small, personalized product. Staff also invited participants to board and tour the MakerTruck. Sometimes attending STEM night was the extent of a partnership. However, when teachers expressed additional interest beyond STEM night, staff developed a range of options meant to provide teachers with short-, medium-, and long-term engagements with the MakerTruck.
For short-term engagements, staff typically returned to the school with the MakerTruck to enact a personalized professional development session for a selection of the school’s teachers. Short-term engagements often resulted in a handful of teachers getting more exposure to the tools and techniques useful for enacting maker-based activities, preparing a subset of the school’s teachers to take up doing this work in the school’s makerspace. For medium-term engagements, staff typically returned to the school with the MakerTruck several times to do a series of professional developments with teachers, such as crafting a plan for launching a school makerspace or creating curriculum modules suitable for teachers to use in the school’s makerspace. Medium-term engagements often resulted in teachers creating lessons and activities that were aligned with the goals and values of the school. For long-term engagements, staff not only worked with teachers to create materials suitable for use in their own school makerspaces but also returned with the MakerTruck and assisted in enacting projects with the students at the school. During one such project, staff worked with a teacher to create vinyl-cut colonization flags as a part of an extended unit related to planning a rocket mission to Mars (). As the length and depth of these engagements increased, teachers developed increased confidence and skills in planning and enacting maker-based activities.
Discussion
Reflecting on our previous experiences with STEM-focused mobile instructional spaces, we would like to acknowledge the fast-paced, challenging nature of this work. Operating a mobile instructional space entails performing physically demanding tasks in a variety of environments, delivering high-quality educational experiences to a wide range of stakeholders with a breadth of knowledge and interests, and managing an array of complex tools and technologies. All told, this can add up to a lot of work. Therefore, we commend the individuals and organizations who operate STEM-focused mobile instructional spaces.
In this article, we shared how mobile instructional spaces are excellent vehicles for improving integrated STEM learning experiences for K–12 students and teachers. Specifically, we listed and described three best practices (i.e., creating a shared culture, fostering agency, and offering multiple pathways for engagement) that we have found to be valuable guides when deploying STEM-focused mobile instructional spaces. These three best practices provide a beginning framework for ensuring that the interactions between mobile instructional space staff and the students and teachers with whom they work are inclusive, impactful, and extend across time and space.
While we have found these best practices to be quite helpful for designing learning experiences and would recommend them to colleagues who run mobile instructional spaces or who are considering creating a mobile instructional space, we also recognize that this list is incomplete. There are a variety of other best practices (especially depending on the mission of the specific mobile instructional space) that are valuable and would allow students and teachers to have high-quality STEM learning experiences.
Next steps
We believe that demonstrating the effectiveness of STEM-focused mobile instructional spaces through increased research will help cement the important role they play in bridging the gap between in-school and out-of-school STEM learning. Thus, one of our recommendations for the future is for organizations who operate STEM-focused mobile instructional spaces to engage in research by measuring outcomes (e.g., academic, attitudinal, motivational) related to working with students and teachers in different contexts. There are several ways to do this, including by deploying surveys and questionnaires (both before and after working with populations of students and teachers) or by collecting interview or focus group data from participants.
Our own efforts to measure STEM skills, practices, and content knowledge have often taken a back seat to designing engaging learning environments and creating broadly accessible learning activities for teachers and students. However, the future of STEM-focused instructional spaces would be even brighter if there was ongoing research surrounding these endeavors that supported the activities and actions of STEM-focused mobile instructional space staff. Looking forward in our own work related to STEM-focused mobile instructional spaces, we recognize the need to continue to share our experiences and what we have learned. By sharing our experiences, we can ensure that formal and informal STEM educators who make up this unique community can learn from each other and that STEM-focused mobile instructional spaces can remain a viable place for students and teachers to learn STEM skills, practices, and content knowledge.
References
- Bhabha, H. 1994. The Location of Culture. London, UK and New York, NY: Routledge.
- Bybee, R. W. 2010. “Advancing STEM Education: A 2020 Vision.” Technology and Engineering Teacher 70 (1): 30–35.
- Fox, J. 2015. “Changes in Urban Youths’ Attitude towards Science and Perception of a Mobile Science Lab Experience.” Unpublished doctoral diss., Columbia University.
- Heydari, R. 2020. “The Impacts of Informal Science Education on Students’ Science Identity and Understanding of Science Inquiry.” Unpublished doctoral diss., Columbia University.
- Martin, L. 2015. “The Promise of the Maker Movement for Education.” Journal of Pre-College Engineering Education Research (J-PEER) 5 (1): 30–39.
- Moore, T. J., and K. A. Smith. 2014. “Advancing the State of the Art of STEM Integration.” Journal of STEM Education: Innovations and Research 15 (1): 5–10.
- NRC (National Research Council). 2014. STEM Integration in K–12 Education: Status, Prospects, and an Agenda for Research. Washington: National Academies Press.
- Papert, S. 1991. “Situating Constructionism.” In Constructionism, edited by S. Papert and I. Harel. Cambridge, MA: MIT Press.
- Perot Museum of Nature and Science. 2023. “Community engagement: TECH Truck.” September 7. https://www.perotmuseum.org/events/children-and-families/tech-truck/
- Roehrig, G. H., E. A. Dare, J. A. Ellis, and E. Ring-Whalen. 2021. “Beyond the Basics: A Detailed Conceptual Framework of Integrated STEM.” Disciplinary and Interdisciplinary Science Education Research 3 (1): 1–18.
- Soja, E. 1996. Thirdspace: Journeys to Los Angeles and Other Real-and-Imagined Places. Oxford, UK: Basil Blackwell.